Abstract
We previously showed that glucosylation of lysine residues of low density lipoproteins (LDL) blocks high-affinity degradation by cultured human fibroblasts, and markedly slows LDL turnover in guinea pigs. The present studies were done to evaluate glucosylated (GLC) LDL as a tracer of receptor-independent LDL catabolism, and to compare it with two other modified LDL, methylated (MET) LDL, and cyclohexanedione (CHD)-treated LDL, which have been used previously for this purpose. Glucosylation of LDL did not affect receptor-independent degradation in vivo, as the turnover of GLC-LDL and native LDL were similar in the LDL receptor-deficient, Watanabe heritable hyperlipidemic rabbit. Each modified radiolabeled LDL preparation was injected into eight guinea pigs, and fractional catabolic rates (FCR) determined. The FCR of GLC-LDL (0.024 +/- 0.005 h-1; SD) was similar to that of MET-LDL (0.023 +/- 0.006 h-1), and approximately 22% of that of native LDL (0.105 +/- 0.02 h-1). The FCR of CHD-LDL was greater than that of the other modified LDL, and it varied depending on how soon after preparation the CHD-LDL was injected: when used within 2 h of preparation, the mean FCR was 0.044 +/- 0.007 h-1 (n = 4); when used after overnight dialysis at 4 degrees C, the mean FCR was 0.082 +/- 0.03 h-1 (n = 4). This suggests that CHD-LDL overestimates the amount of LDL degraded by receptor-independent pathways, perhaps because the CHD modification is spontaneously reversible. The present studies indicate that GLC-LDL is a useful tracer of receptor-independent LDL catabolism in animals.
Full text
PDF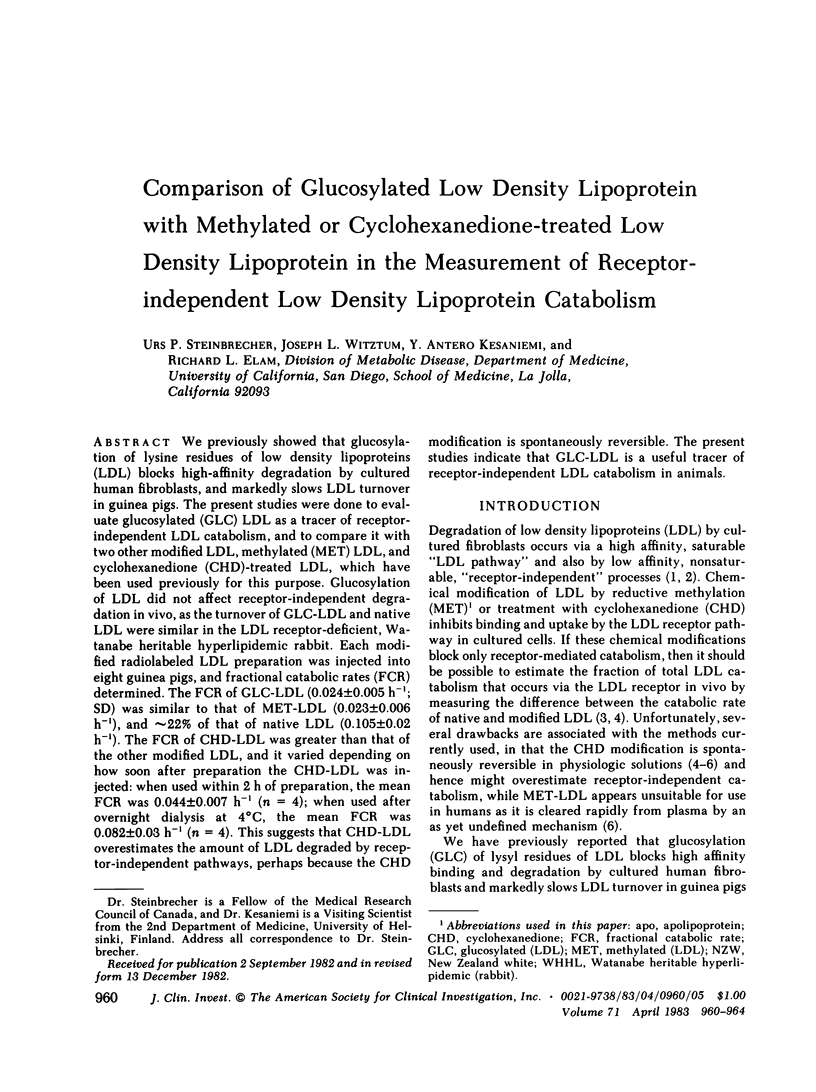
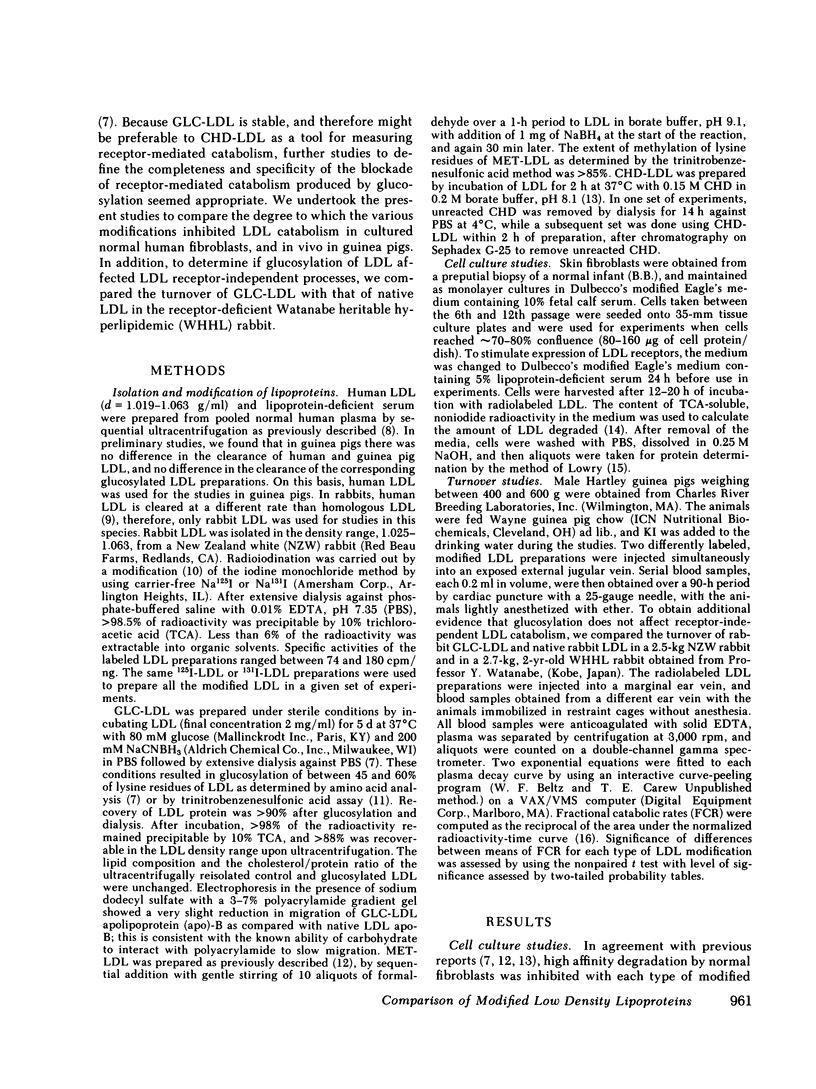
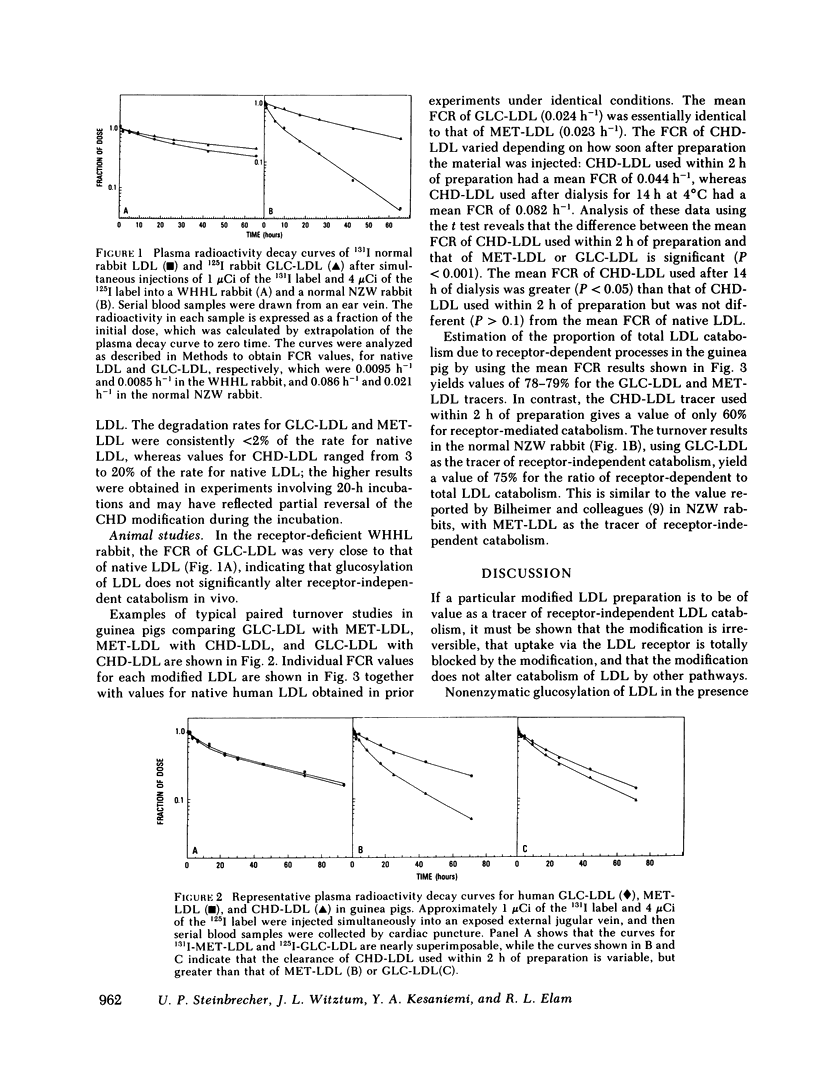
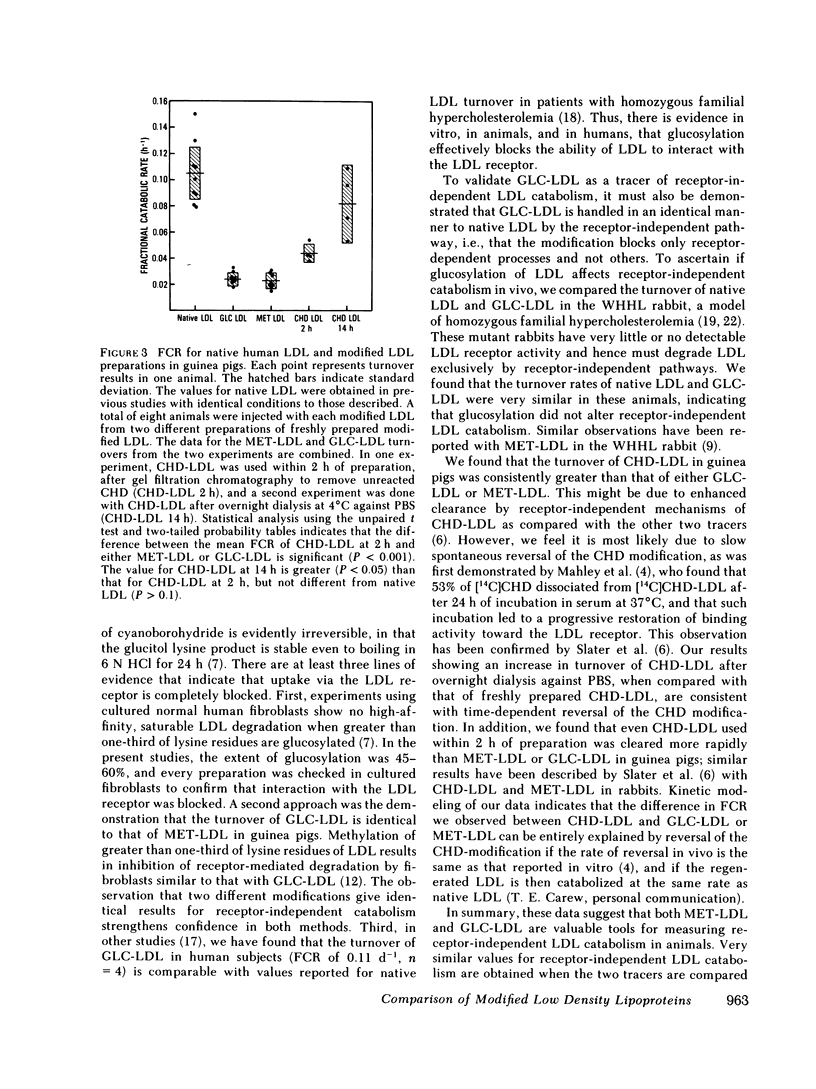
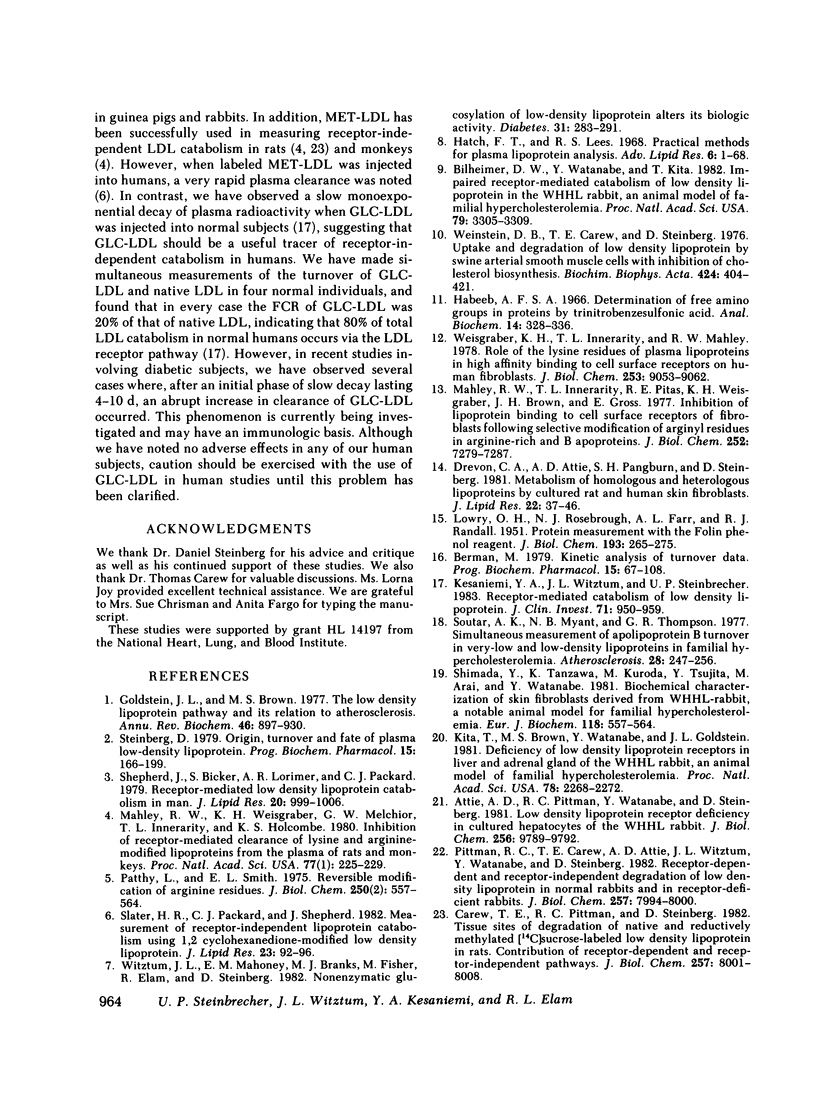
Selected References
These references are in PubMed. This may not be the complete list of references from this article.
- Attie A. D., Pittman R. C., Watanabe Y., Steinberg D. Low density lipoprotein receptor deficiency in cultured hepatocytes of the WHHL rabbit. Further evidence of two pathways for catabolism of exogenous proteins. J Biol Chem. 1981 Oct 10;256(19):9789–9792. [PubMed] [Google Scholar]
- Berman M. Kinetic analysis of turnover data. Prog Biochem Pharmacol. 1979;15:67–108. [PubMed] [Google Scholar]
- Bilheimer D. W., Watanabe Y., Kita T. Impaired receptor-mediated catabolism of low density lipoprotein in the WHHL rabbit, an animal model of familial hypercholesterolemia. Proc Natl Acad Sci U S A. 1982 May;79(10):3305–3309. doi: 10.1073/pnas.79.10.3305. [DOI] [PMC free article] [PubMed] [Google Scholar]
- Carew T. E., Pittman R. C., Steinberg D. Tissue sites of degradation of native and reductively methylated [14C]sucrose-labeled low density lipoprotein in rats. Contribution of receptor-dependent and receptor-independent pathways. J Biol Chem. 1982 Jul 25;257(14):8001–8008. [PubMed] [Google Scholar]
- Drevon C. A., Attie A. D., Pangburn S. H., Steinberg D. Metabolism of homologous and heterologous lipoproteins by cultured rat and human skin fibroblasts. J Lipid Res. 1981 Jan;22(1):37–46. [PubMed] [Google Scholar]
- Goldstein J. L., Brown M. S. The low-density lipoprotein pathway and its relation to atherosclerosis. Annu Rev Biochem. 1977;46:897–930. doi: 10.1146/annurev.bi.46.070177.004341. [DOI] [PubMed] [Google Scholar]
- Habeeb A. F. Determination of free amino groups in proteins by trinitrobenzenesulfonic acid. Anal Biochem. 1966 Mar;14(3):328–336. doi: 10.1016/0003-2697(66)90275-2. [DOI] [PubMed] [Google Scholar]
- Hatch F. T. Practical methods for plasma lipoprotein analysis. Adv Lipid Res. 1968;6:1–68. [PubMed] [Google Scholar]
- Kesaniemi Y. A., Witztum J. L., Steinbrecher U. P. Receptor-mediated catabolism of low density lipoprotein in man. Quantitation using glucosylated low density lipoprotein. J Clin Invest. 1983 Apr;71(4):950–959. doi: 10.1172/JCI110849. [DOI] [PMC free article] [PubMed] [Google Scholar]
- Kita T., Brown M. S., Watanabe Y., Goldstein J. L. Deficiency of low density lipoprotein receptors in liver and adrenal gland of the WHHL rabbit, an animal model of familial hypercholesterolemia. Proc Natl Acad Sci U S A. 1981 Apr;78(4):2268–2272. doi: 10.1073/pnas.78.4.2268. [DOI] [PMC free article] [PubMed] [Google Scholar]
- LOWRY O. H., ROSEBROUGH N. J., FARR A. L., RANDALL R. J. Protein measurement with the Folin phenol reagent. J Biol Chem. 1951 Nov;193(1):265–275. [PubMed] [Google Scholar]
- Mahley R. W., Innerarity T. L., Pitas R. E., Weisgraber K. H., Brown J. H., Gross E. Inhibition of lipoprotein binding to cell surface receptors of fibroblasts following selective modification of arginyl residues in arginine-rich and B apoproteins. J Biol Chem. 1977 Oct 25;252(20):7279–7287. [PubMed] [Google Scholar]
- Mahley R. W., Weisgraber K. H., Melchior G. W., Innerarity T. L., Holcombe K. S. Inhibition of receptor-mediated clearance of lysine and arginine-modified lipoproteins from the plasma of rats and monkeys. Proc Natl Acad Sci U S A. 1980 Jan;77(1):225–229. doi: 10.1073/pnas.77.1.225. [DOI] [PMC free article] [PubMed] [Google Scholar]
- Patthy L., Smith E. L. Reversible modification of arginine residues. Application to sequence studies by restriction of tryptic hydrolysis to lysine residues. J Biol Chem. 1975 Jan 25;250(2):557–564. [PubMed] [Google Scholar]
- Pittman R. C., Carew T. E., Attie A. D., Witztum J. L., Watanabe Y., Steinberg D. Receptor-dependent and receptor-independent degradation of low density lipoprotein in normal rabbits and in receptor-deficient mutant rabbits. J Biol Chem. 1982 Jul 25;257(14):7994–8000. [PubMed] [Google Scholar]
- Shepherd J., Bicker S., Lorimer A. R., Packard C. J. Receptor-mediated low density lipoprotein catabolism in man. J Lipid Res. 1979 Nov;20(8):999–1006. [PubMed] [Google Scholar]
- Shimada Y., Tanzawa K., Kuroda M., Tsujita Y., Arai M., Watanabe Y. Biochemical characterization of skin fibroblasts derived from WHHL-rabbit, a notable animal model for familial hypercholesterolemia. Eur J Biochem. 1981 Sep 1;118(3):557–564. doi: 10.1111/j.1432-1033.1981.tb05555.x. [DOI] [PubMed] [Google Scholar]
- Slater H. R., Packard C. J., Shepherd J. Measurement of receptor-independent lipoprotein catabolism using 1,2 cyclohexanedione-modified low density lipoprotein. J Lipid Res. 1982 Jan;23(1):92–96. [PubMed] [Google Scholar]
- Soutar A. K., Myant N. B., Thompson G. R. Simultaneous measurement of apolipoprotein B turnover in very-low-and low-density lipoproteins in familial hypercholesterolaemia. Atherosclerosis. 1977 Nov;28(3):247–256. doi: 10.1016/0021-9150(77)90174-5. [DOI] [PubMed] [Google Scholar]
- Steinberg D. Origin, turnover and fate of plasma low-density lipoprotein. Prog Biochem Pharmacol. 1979;15:166–199. [PubMed] [Google Scholar]
- Weinstein D. B., Carew T. E., Steinberg D. Uptake and degradation of low density lipoprotein by swine arterial smoot muscle cells with inhibition of cholesterol biosynthesis. Biochim Biophys Acta. 1976 Mar 26;424(3):404–421. doi: 10.1016/0005-2760(76)90030-8. [DOI] [PubMed] [Google Scholar]
- Weisgraber K. H., Innerarity T. L., Mahley R. W. Role of lysine residues of plasma lipoproteins in high affinity binding to cell surface receptors on human fibroblasts. J Biol Chem. 1978 Dec 25;253(24):9053–9062. [PubMed] [Google Scholar]
- Witztum J. L., Mahoney E. M., Branks M. J., Fisher M., Elam R., Steinberg D. Nonenzymatic glucosylation of low-density lipoprotein alters its biologic activity. Diabetes. 1982 Apr;31(4 Pt 1):283–291. doi: 10.2337/diab.31.4.283. [DOI] [PubMed] [Google Scholar]