Abstract
Sarcoplasmic reticulum Ca2+ in vascular smooth muscle may be separated into at least two types of Ca2+ stores, one releasable by inositol 1,4,5-trisphosphate and the other releasable by caffeine and ryanodine. We employed digital imaging with fura-2 to study the effects of thapsigargin (which blocks Ca2+ sequestration in the inositol trisphosphate-sensitive store) and caffeine on the cytosolic free Ca2+ concentration ([Ca2+]cyt) in cultured arterial myocytes. These agents increased [Ca2+]cyt by depleting different Ca2+ stores in the absence of extracellular Ca2+. Moreover, Ca2+ could be transferred between the two stores, as prior application of caffeine, which alone evoked little or no rise in [Ca2+]cyt, significantly augmented the response to thapsigargin. Conversely, a substantial caffeine-induced rise in [Ca2+]cyt was observed only after the ability of the thapsigargin-sensitive Ca2+ store to sequester Ca2+ was inhibited. This suggests that the caffeine-sensitive store may have a thapsigargin-insensitive Ca(2+)-sequestration mechanism. To complement these fura-2 experiments, chlortetracycline was used to visualize the Ca2+ stores directly. The latter studies revealed spatial differences in the location of the thapsigargin-sensitive and caffeine-sensitive Ca2+ stores (measured as thapsigargin-sensitive and caffeine-sensitive chlortetracycline fluorescence). Thus, these two types of stores appear to be both functionally and spatially distinct.
Full text
PDF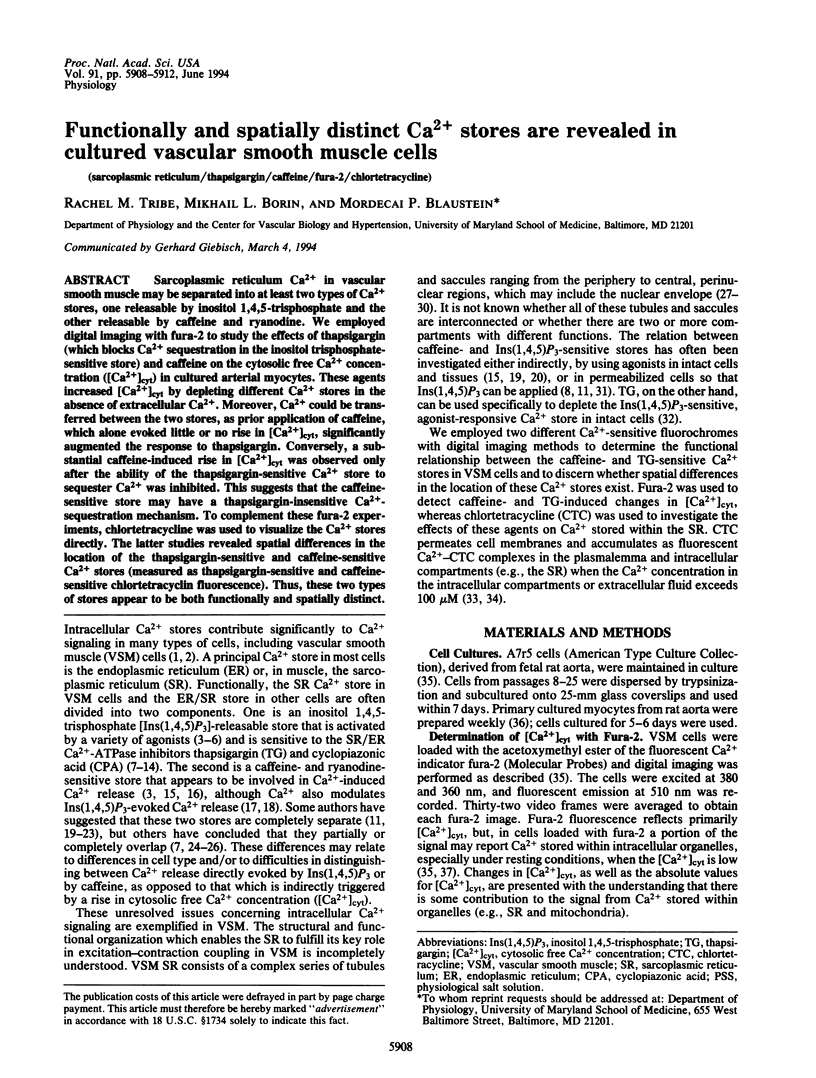
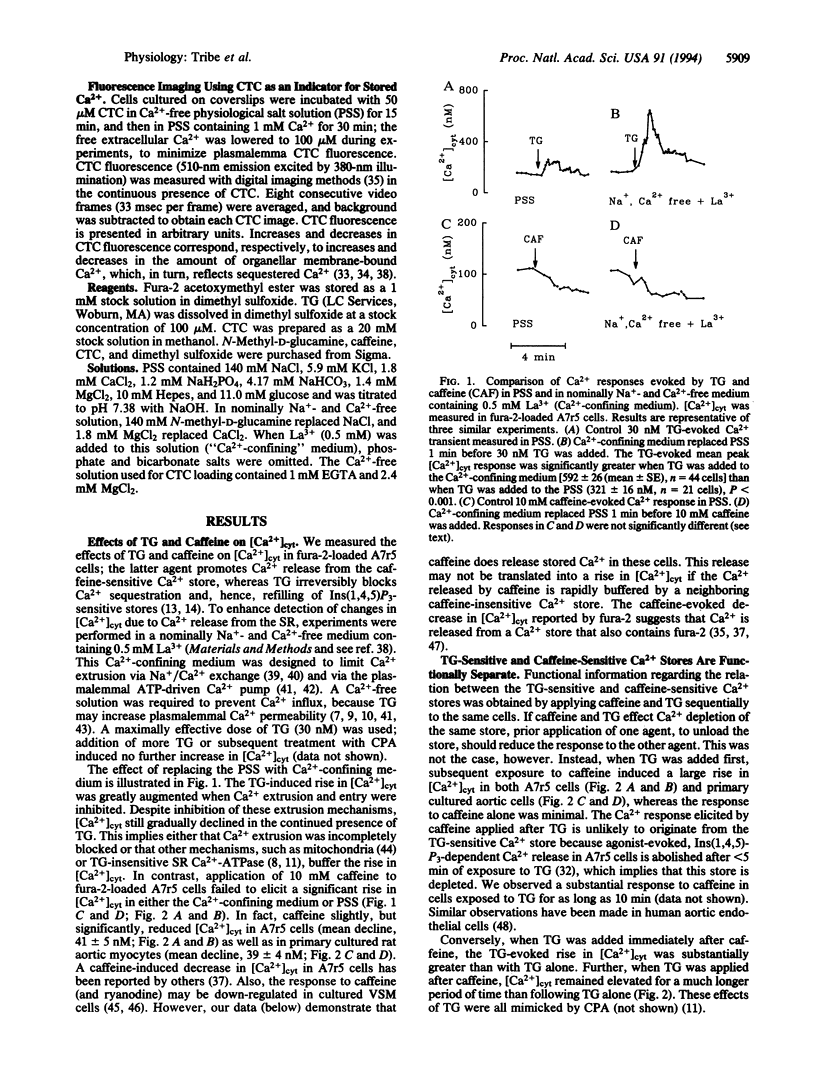
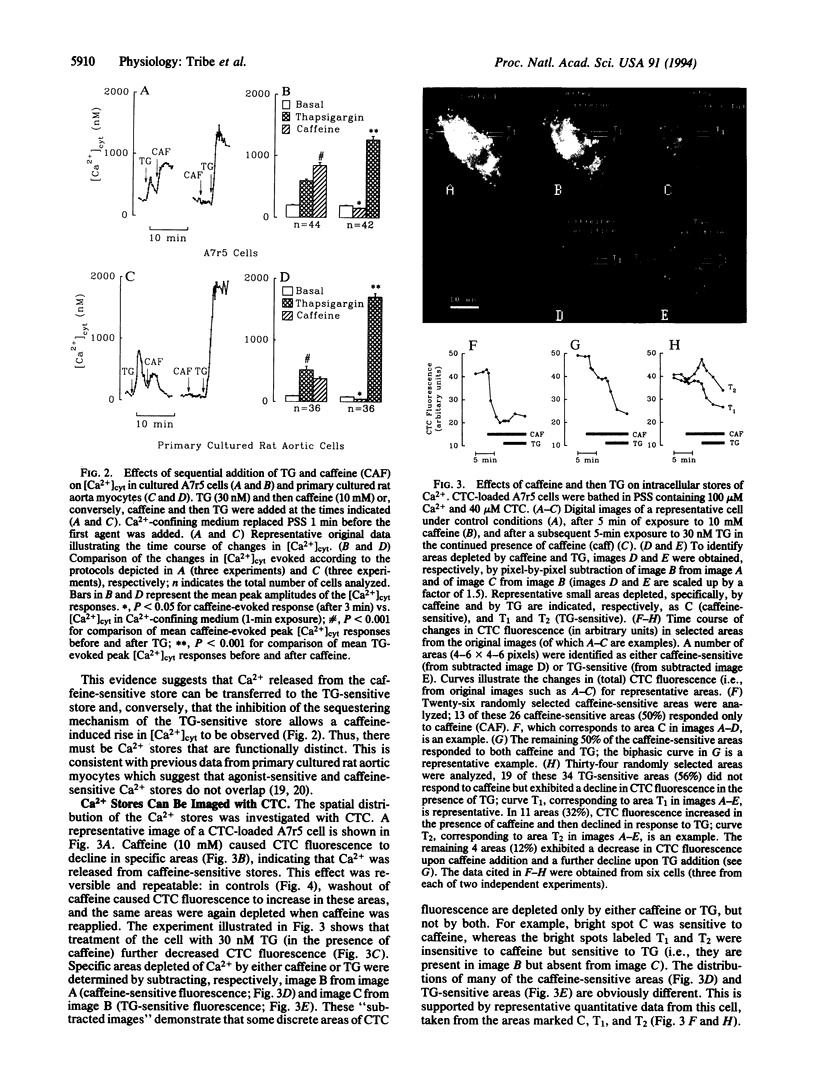
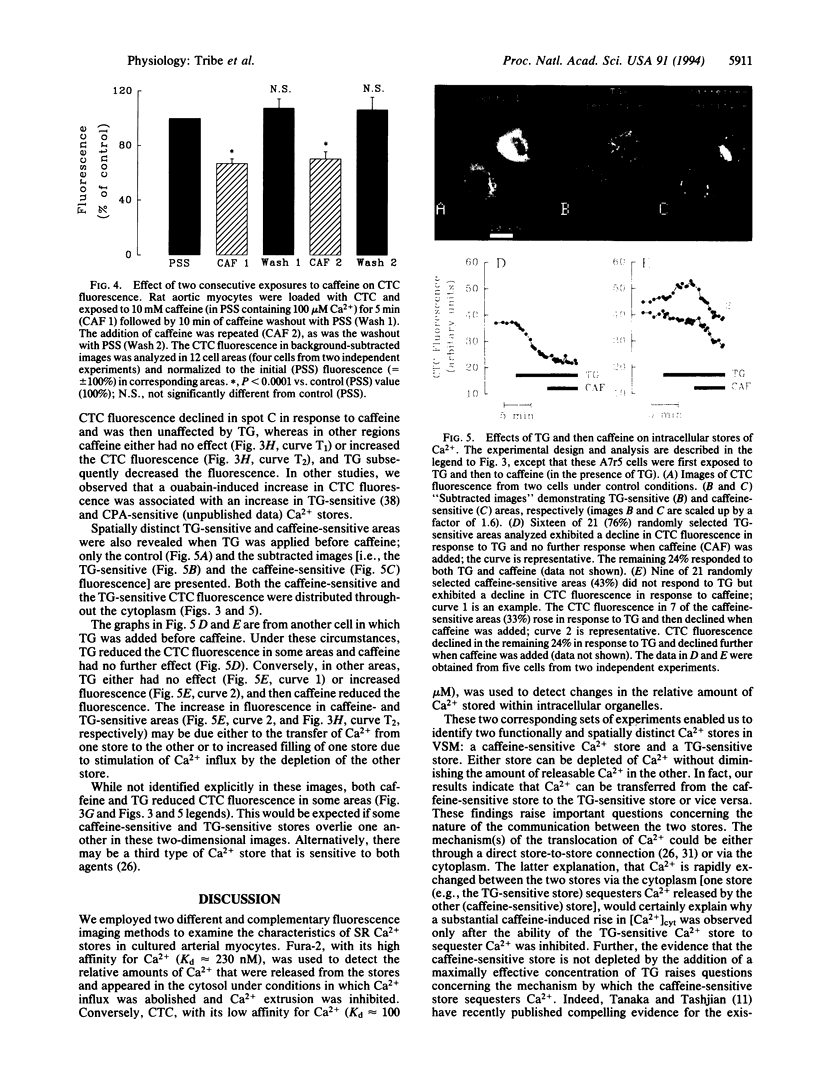
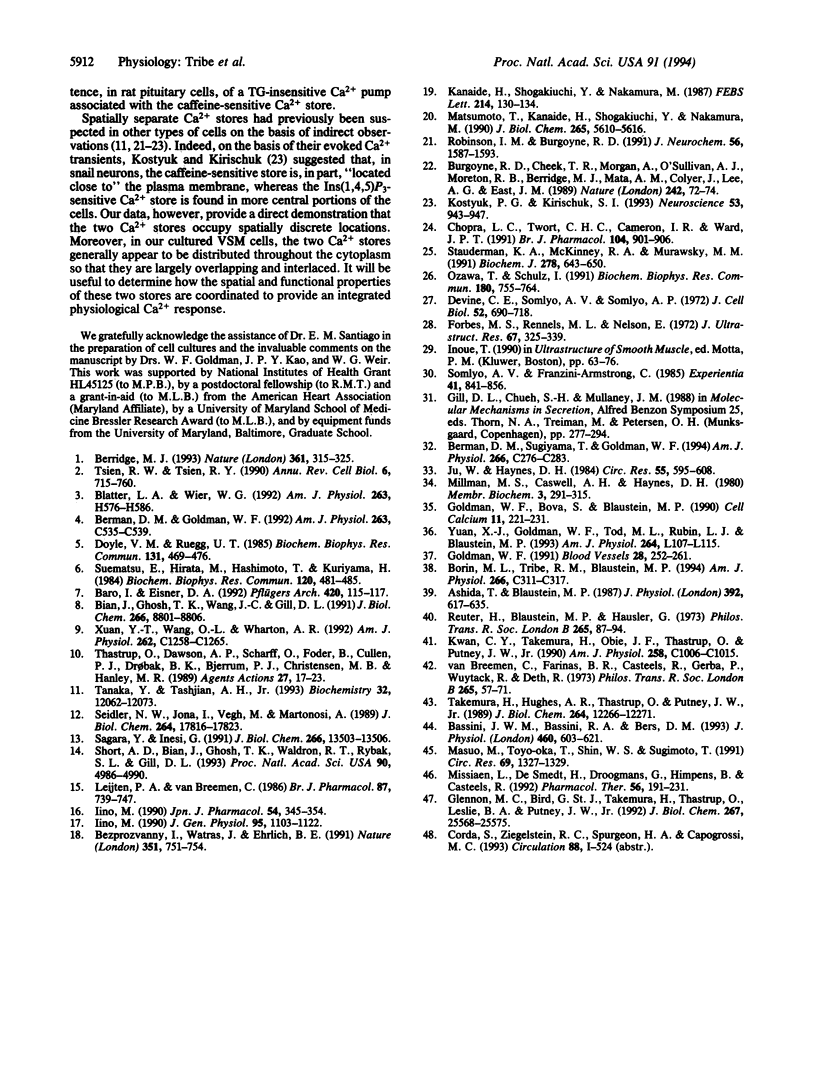
Images in this article
Selected References
These references are in PubMed. This may not be the complete list of references from this article.
- Ashida T., Blaustein M. P. Regulation of cell calcium and contractility in mammalian arterial smooth muscle: the role of sodium-calcium exchange. J Physiol. 1987 Nov;392:617–635. doi: 10.1113/jphysiol.1987.sp016800. [DOI] [PMC free article] [PubMed] [Google Scholar]
- Baró I., Eisner D. A. The effects of thapsigargin on [Ca2+]i in isolated rat mesenteric artery vascular smooth muscle cells. Pflugers Arch. 1992 Jan;420(1):115–117. doi: 10.1007/BF00378652. [DOI] [PubMed] [Google Scholar]
- Bassani J. W., Bassani R. A., Bers D. M. Ca2+ cycling between sarcoplasmic reticulum and mitochondria in rabbit cardiac myocytes. J Physiol. 1993 Jan;460:603–621. doi: 10.1113/jphysiol.1993.sp019489. [DOI] [PMC free article] [PubMed] [Google Scholar]
- Berman D. M., Goldman W. F. Stored calcium modulates inositol phosphate synthesis in cultured smooth muscle cells. Am J Physiol. 1992 Aug;263(2 Pt 1):C535–C539. doi: 10.1152/ajpcell.1992.263.2.C535. [DOI] [PubMed] [Google Scholar]
- Berman D. M., Sugiyama T., Goldman W. F. Ca2+ stores in smooth muscle cells: Ca2+ buffering and coupling to AVP-evoked inositol phosphate synthesis. Am J Physiol. 1994 Jan;266(1 Pt 1):C276–C283. doi: 10.1152/ajpcell.1994.266.1.C276. [DOI] [PubMed] [Google Scholar]
- Berridge M. J. Inositol trisphosphate and calcium signalling. Nature. 1993 Jan 28;361(6410):315–325. doi: 10.1038/361315a0. [DOI] [PubMed] [Google Scholar]
- Bezprozvanny I., Watras J., Ehrlich B. E. Bell-shaped calcium-response curves of Ins(1,4,5)P3- and calcium-gated channels from endoplasmic reticulum of cerebellum. Nature. 1991 Jun 27;351(6329):751–754. doi: 10.1038/351751a0. [DOI] [PubMed] [Google Scholar]
- Bian J. H., Ghosh T. K., Wang J. C., Gill D. L. Identification of intracellular calcium pools. Selective modification by thapsigargin. J Biol Chem. 1991 May 15;266(14):8801–8806. [PubMed] [Google Scholar]
- Blatter L. A., Wier W. G. Agonist-induced [Ca2+]i waves and Ca(2+)-induced Ca2+ release in mammalian vascular smooth muscle cells. Am J Physiol. 1992 Aug;263(2 Pt 2):H576–H586. doi: 10.1152/ajpheart.1992.263.2.H576. [DOI] [PubMed] [Google Scholar]
- Borin M. L., Tribe R. M., Blaustein M. P. Increased intracellular Na+ augments mobilization of Ca2+ from SR in vascular smooth muscle cells. Am J Physiol. 1994 Jan;266(1 Pt 1):C311–C317. doi: 10.1152/ajpcell.1994.266.1.C311. [DOI] [PubMed] [Google Scholar]
- Burgoyne R. D., Cheek T. R., Morgan A., O'Sullivan A. J., Moreton R. B., Berridge M. J., Mata A. M., Colyer J., Lee A. G., East J. M. Distribution of two distinct Ca2+-ATPase-like proteins and their relationships to the agonist-sensitive calcium store in adrenal chromaffin cells. Nature. 1989 Nov 2;342(6245):72–74. doi: 10.1038/342072a0. [DOI] [PubMed] [Google Scholar]
- Chopra L. C., Twort C. H., Cameron I. R., Ward J. P. Inositol 1,4,5-trisphosphate- and guanosine 5'-O-(3-thiotriphosphate)-induced Ca2+ release in cultured airway smooth muscle. Br J Pharmacol. 1991 Dec;104(4):901–906. doi: 10.1111/j.1476-5381.1991.tb12524.x. [DOI] [PMC free article] [PubMed] [Google Scholar]
- Devine C. E., Somlyo A. V., Somlyo A. P. Sarcoplasmic reticulum and excitation-contraction coupling in mammalian smooth muscles. J Cell Biol. 1972 Mar;52(3):690–718. doi: 10.1083/jcb.52.3.690. [DOI] [PMC free article] [PubMed] [Google Scholar]
- Doyle V. M., Rüegg U. T. Vasopressin induced production of inositol trisphosphate and calcium efflux in a smooth muscle cell line. Biochem Biophys Res Commun. 1985 Aug 30;131(1):469–476. doi: 10.1016/0006-291x(85)91826-1. [DOI] [PubMed] [Google Scholar]
- Forbes M. S., Rennels M. L., Nelson E. Caveolar systems and sarcoplasmic reticulum in coronary smooth muscle cells of the mouse. J Ultrastruct Res. 1979 Jun;67(3):325–339. doi: 10.1016/s0022-5320(79)80032-5. [DOI] [PubMed] [Google Scholar]
- Glennon M. C., Bird G. S., Takemura H., Thastrup O., Leslie B. A., Putney J. W., Jr In situ imaging of agonist-sensitive calcium pools in AR4-2J pancreatoma cells. Evidence for an agonist- and inositol 1,4,5-trisphosphate-sensitive calcium pool in or closely associated with the nuclear envelope. J Biol Chem. 1992 Dec 15;267(35):25568–25575. [PubMed] [Google Scholar]
- Goldman W. F., Bova S., Blaustein M. P. Measurement of intracellular Ca2+ in cultured arterial smooth muscle cells using Fura-2 and digital imaging microscopy. Cell Calcium. 1990 Feb-Mar;11(2-3):221–231. doi: 10.1016/0143-4160(90)90073-4. [DOI] [PubMed] [Google Scholar]
- Goldman W. F. Spatial and temporal resolution of serotonin-induced changes in intracellular calcium in a cultured arterial smooth muscle cell line. Blood Vessels. 1991;28(1-3):252–261. doi: 10.1159/000158870. [DOI] [PubMed] [Google Scholar]
- Iino M. Biphasic Ca2+ dependence of inositol 1,4,5-trisphosphate-induced Ca release in smooth muscle cells of the guinea pig taenia caeci. J Gen Physiol. 1990 Jun;95(6):1103–1122. doi: 10.1085/jgp.95.6.1103. [DOI] [PMC free article] [PubMed] [Google Scholar]
- Iino M. Calcium release mechanisms in smooth muscle. Jpn J Pharmacol. 1990 Dec;54(4):345–354. doi: 10.1254/jjp.54.345. [DOI] [PubMed] [Google Scholar]
- Jy W., Haynes D. H. Intracellular calcium storage and release in the human platelet. Chlorotetracycline as a continuous monitor. Circ Res. 1984 Nov;55(5):595–608. doi: 10.1161/01.res.55.5.595. [DOI] [PubMed] [Google Scholar]
- Kanaide H., Shogakiuchi Y., Nakamura M. The norepinephrine-sensitive Ca2+-storage site differs from the caffeine-sensitive site in vascular smooth muscle of the rat aorta. FEBS Lett. 1987 Apr 6;214(1):130–134. doi: 10.1016/0014-5793(87)80027-3. [DOI] [PubMed] [Google Scholar]
- Kostyuk P. G., Kirischuk S. I. Spatial heterogeneity of caffeine- and inositol 1,4,5-trisphosphate-induced Ca2+ transients in isolated snail neurons. Neuroscience. 1993 Apr;53(4):943–947. doi: 10.1016/0306-4522(93)90479-y. [DOI] [PubMed] [Google Scholar]
- Kwan C. Y., Takemura H., Obie J. F., Thastrup O., Putney J. W., Jr Effects of MeCh, thapsigargin, and La3+ on plasmalemmal and intracellular Ca2+ transport in lacrimal acinar cells. Am J Physiol. 1990 Jun;258(6 Pt 1):C1006–C1015. doi: 10.1152/ajpcell.1990.258.6.C1006. [DOI] [PubMed] [Google Scholar]
- Leijten P. A., van Breemen C. The relationship between noradrenaline-induced contraction and 45Ca efflux stimulation in rabbit mesenteric artery. Br J Pharmacol. 1986 Dec;89(4):739–747. doi: 10.1111/j.1476-5381.1986.tb11178.x. [DOI] [PMC free article] [PubMed] [Google Scholar]
- Masuo M., Toyo-oka T., Shin W. S., Sugimoto T. Growth-dependent alterations of intracellular Ca(2+)-handling mechanisms of vascular smooth muscle cells. PDGF negatively regulates functional expression of voltage-dependent, IP3-mediated, and CA(2+)-induced Ca2+ release channels. Circ Res. 1991 Nov;69(5):1327–1339. doi: 10.1161/01.res.69.5.1327. [DOI] [PubMed] [Google Scholar]
- Matsumoto T., Kanaide H., Shogakiuchi Y., Nakamura M. Characteristics of the histamine-sensitive calcium store in vascular smooth muscle. Comparison with norepinephrine- or caffeine-sensitive stores. J Biol Chem. 1990 Apr 5;265(10):5610–5616. [PubMed] [Google Scholar]
- Millman M. S., Caswell A. H., Haynes D. H. Kinetics of chlorotetracycline permeation in fragmented, ATPase-rich sarcoplasmic reticulum. Membr Biochem. 1980;3(4):291–315. doi: 10.3109/09687688009063890. [DOI] [PubMed] [Google Scholar]
- Missiaen L., De Smedt H., Droogmans G., Himpens B., Casteels R. Calcium ion homeostasis in smooth muscle. Pharmacol Ther. 1992 Nov;56(2):191–231. doi: 10.1016/0163-7258(92)90017-t. [DOI] [PubMed] [Google Scholar]
- Ozawa T., Schulz I. H+ uptake increases GTP-induced connection of inositol 1,4,5-trisphosphate- and caffeine-sensitive calcium pools in pancreatic microsomal vesicles. Biochem Biophys Res Commun. 1991 Oct 31;180(2):755–764. doi: 10.1016/s0006-291x(05)81130-1. [DOI] [PubMed] [Google Scholar]
- Reuter H., Blaustein M. P., Haeusler G. Na-Ca exchange and tension development in arterial smooth muscle. Philos Trans R Soc Lond B Biol Sci. 1973 Mar 15;265(867):87–94. doi: 10.1098/rstb.1973.0011. [DOI] [PubMed] [Google Scholar]
- Robinson I. M., Burgoyne R. D. Characterisation of distinct inositol 1,4,5-trisphosphate-sensitive and caffeine-sensitive calcium stores in digitonin-permeabilised adrenal chromaffin cells. J Neurochem. 1991 May;56(5):1587–1593. doi: 10.1111/j.1471-4159.1991.tb02055.x. [DOI] [PubMed] [Google Scholar]
- Sagara Y., Inesi G. Inhibition of the sarcoplasmic reticulum Ca2+ transport ATPase by thapsigargin at subnanomolar concentrations. J Biol Chem. 1991 Jul 25;266(21):13503–13506. [PubMed] [Google Scholar]
- Seidler N. W., Jona I., Vegh M., Martonosi A. Cyclopiazonic acid is a specific inhibitor of the Ca2+-ATPase of sarcoplasmic reticulum. J Biol Chem. 1989 Oct 25;264(30):17816–17823. [PubMed] [Google Scholar]
- Short A. D., Bian J., Ghosh T. K., Waldron R. T., Rybak S. L., Gill D. L. Intracellular Ca2+ pool content is linked to control of cell growth. Proc Natl Acad Sci U S A. 1993 Jun 1;90(11):4986–4990. doi: 10.1073/pnas.90.11.4986. [DOI] [PMC free article] [PubMed] [Google Scholar]
- Somlyo A. V., Franzini-Armstrong C. New views of smooth muscle structure using freezing, deep-etching and rotary shadowing. Experientia. 1985 Jul 15;41(7):841–856. doi: 10.1007/BF01970000. [DOI] [PubMed] [Google Scholar]
- Stauderman K. A., McKinney R. A., Murawsky M. M. The role of caffeine-sensitive Ca2+ stores in agonist- and inositol 1,4,5-trisphosphate-induced Ca2+ release from bovine adrenal chromaffin cells. Biochem J. 1991 Sep 15;278(Pt 3):643–650. doi: 10.1042/bj2780643. [DOI] [PMC free article] [PubMed] [Google Scholar]
- Suematsu E., Hirata M., Hashimoto T., Kuriyama H. Inositol 1,4,5-trisphosphate releases Ca2+ from intracellular store sites in skinned single cells of porcine coronary artery. Biochem Biophys Res Commun. 1984 Apr 30;120(2):481–485. doi: 10.1016/0006-291x(84)91279-8. [DOI] [PubMed] [Google Scholar]
- Takemura H., Hughes A. R., Thastrup O., Putney J. W., Jr Activation of calcium entry by the tumor promoter thapsigargin in parotid acinar cells. Evidence that an intracellular calcium pool and not an inositol phosphate regulates calcium fluxes at the plasma membrane. J Biol Chem. 1989 Jul 25;264(21):12266–12271. [PubMed] [Google Scholar]
- Tanaka Y., Tashjian A. H., Jr Functional identification and quantitation of three intracellular calcium pools in GH4C1 cells: evidence that the caffeine-responsive pool is coupled to a thapsigargin-resistant, ATP-dependent process. Biochemistry. 1993 Nov 16;32(45):12062–12073. doi: 10.1021/bi00096a017. [DOI] [PubMed] [Google Scholar]
- Thastrup O., Dawson A. P., Scharff O., Foder B., Cullen P. J., Drøbak B. K., Bjerrum P. J., Christensen S. B., Hanley M. R. Thapsigargin, a novel molecular probe for studying intracellular calcium release and storage. Agents Actions. 1989 Apr;27(1-2):17–23. doi: 10.1007/BF02222186. [DOI] [PubMed] [Google Scholar]
- Tsien R. W., Tsien R. Y. Calcium channels, stores, and oscillations. Annu Rev Cell Biol. 1990;6:715–760. doi: 10.1146/annurev.cb.06.110190.003435. [DOI] [PubMed] [Google Scholar]
- Xuan Y. T., Wang O. L., Whorton A. R. Thapsigargin stimulates Ca2+ entry in vascular smooth muscle cells: nicardipine-sensitive and -insensitive pathways. Am J Physiol. 1992 May;262(5 Pt 1):C1258–C1265. doi: 10.1152/ajpcell.1992.262.5.C1258. [DOI] [PubMed] [Google Scholar]
- Yuan X. J., Goldman W. F., Tod M. L., Rubin L. J., Blaustein M. P. Ionic currents in rat pulmonary and mesenteric arterial myocytes in primary culture and subculture. Am J Physiol. 1993 Feb;264(2 Pt 1):L107–L115. doi: 10.1152/ajplung.1993.264.2.L107. [DOI] [PubMed] [Google Scholar]
- van Breemen C., Farinas B. R., Casteels R., Gerba P., Wuytack F., Deth R. Factors controlling cytoplasmic Ca 2+ concentration. Philos Trans R Soc Lond B Biol Sci. 1973 Mar 15;265(867):57–71. doi: 10.1098/rstb.1973.0009. [DOI] [PubMed] [Google Scholar]