Abstract
It has been proposed that oxygen free radicals mediate damage that occurs during postischemic reperfusion. Recombinant human superoxide dismutase (r-h-SOD) has been shown to be effective at reducing reperfusion injury, but it is not known if this infused enzyme actually reduces oxygen free radical concentrations in the myocardial tissue. Electron paramagnetic resonance spectroscopy was used to directly measure the effect of r-h-SOD on free radical concentrations in the postischemic heart. Hearts were freeze clamped at 77 degrees K after 10 min of normothermic global ischemia followed by 10 s of reflow with control perfusate (n = 7) or perfusate containing 60,000 U r-h-SOD (n = 7). The spectra of these hearts exhibited three different signals: signal A isotropic, g = 2.004, identical to the carbon-centered ubiquinone free radical; signal B anisotropic with axial symmetry, g parallel = 2.033, g perpendicular = 2.005, identical to the oxygen-centered alkyl peroxyl free radical; and the signal C an isotropic triplet, g parallel = 2.000, an = 24 G, similar to a nitrogen-centered free radical such as a peroxyl amine. With r-h-SOD administration the concentration of the oxygen free radical, signal B, was reduced 49% from 6.8 +/- 0.3 microM to 3.5 +/- 0.3 microM (P less than 0.01) and the concentration of the nitrogen free radical, signal C, was reduced 38% from 3.4 +/- 0.3 to 2.1 +/- 0.3 microM (P less than 0.01). The concentration of the carbon-centered free radical, signal A, however, was increased 51% from 3.3 +/- 0.2 to 5.0 +/- 0.2 microM (P less than 0.01). Identical reperfusion with peroxide-inactivated r-h-SOD did not alter the concentrations of free radicals indicating that the specific enzymatic activity of r-h-SOD is required to decrease the concentrations of reactive oxygen free radicals. Additional measurements performed varying the duration of reflow demonstrate a burst of oxygen free radical generation peaking at 10 s of reperfusion. r-h-SOD entirely abolished this burst. These studies demonstrate that superoxide-derived free radicals are generated during postischemic reperfusion and suggest that the beneficial effect of r-h-SOD is due to its specific enzymatic scavenging of superoxide free radicals.
Full text
PDF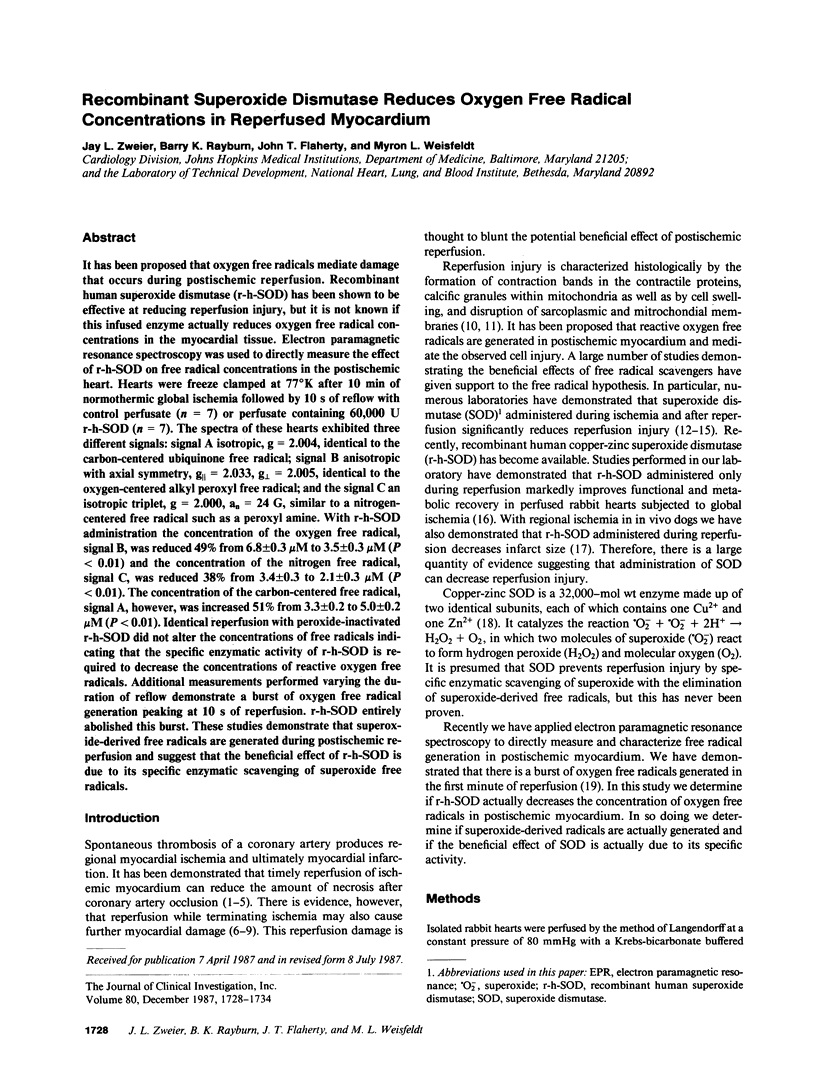
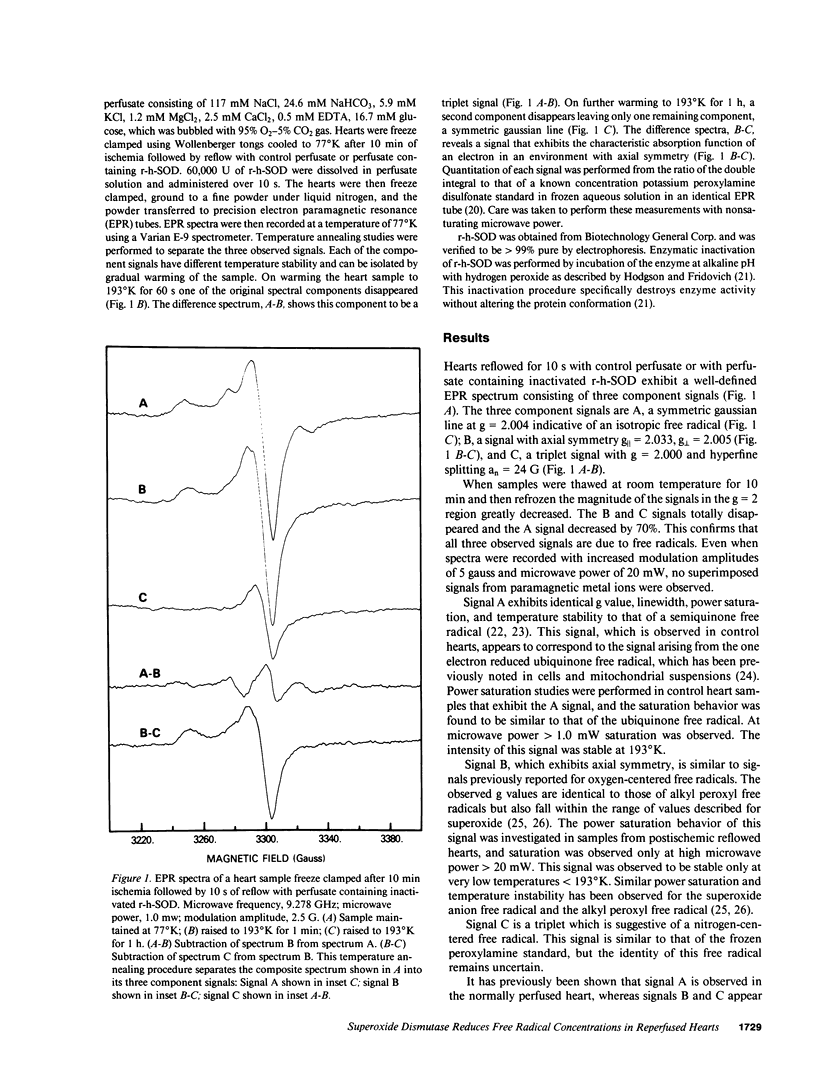
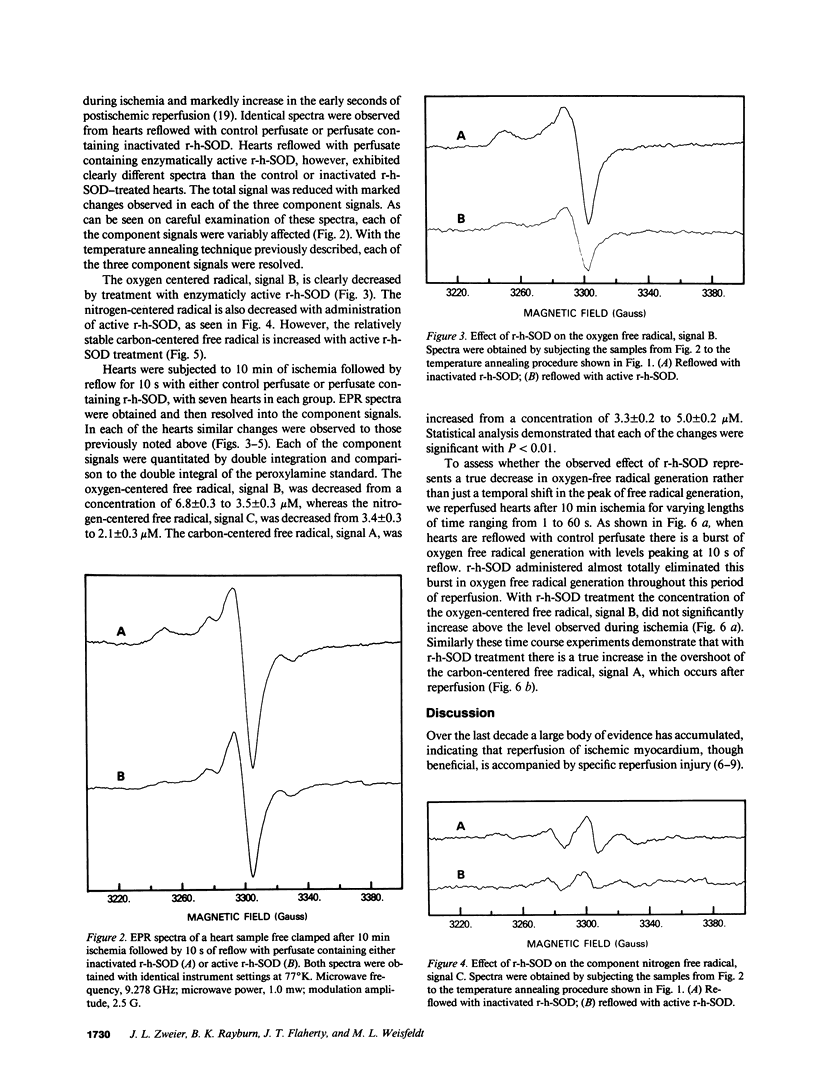
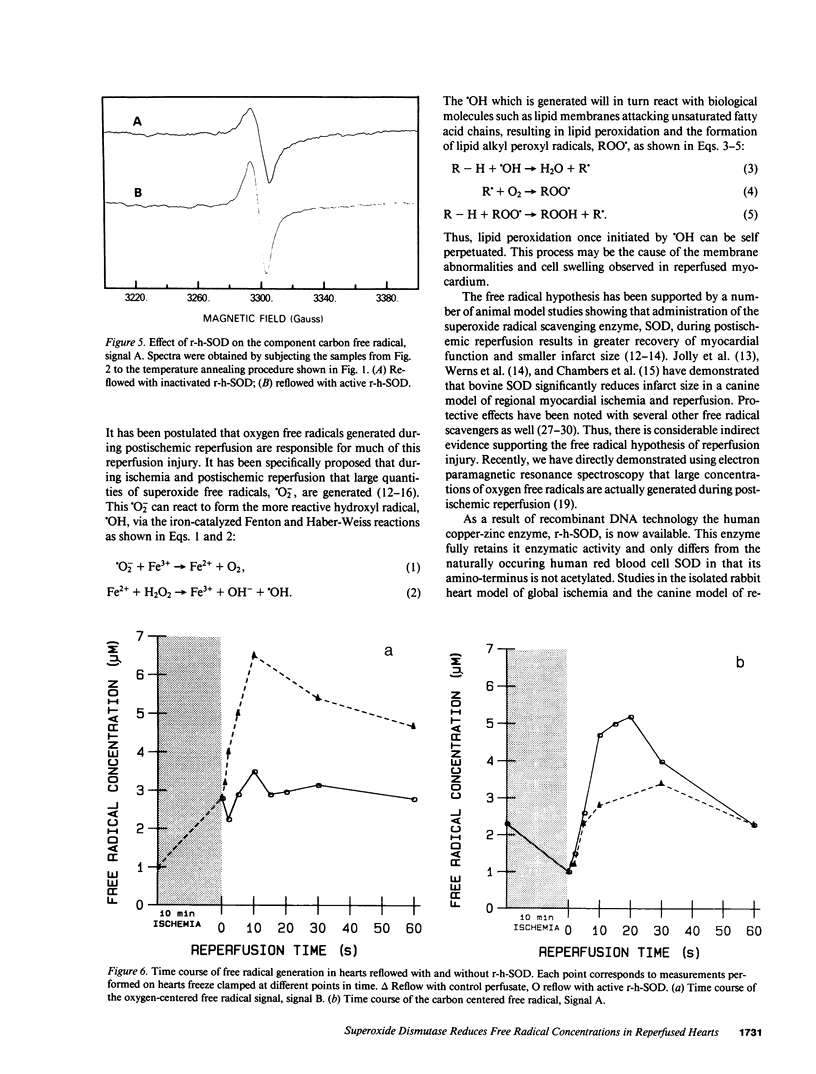
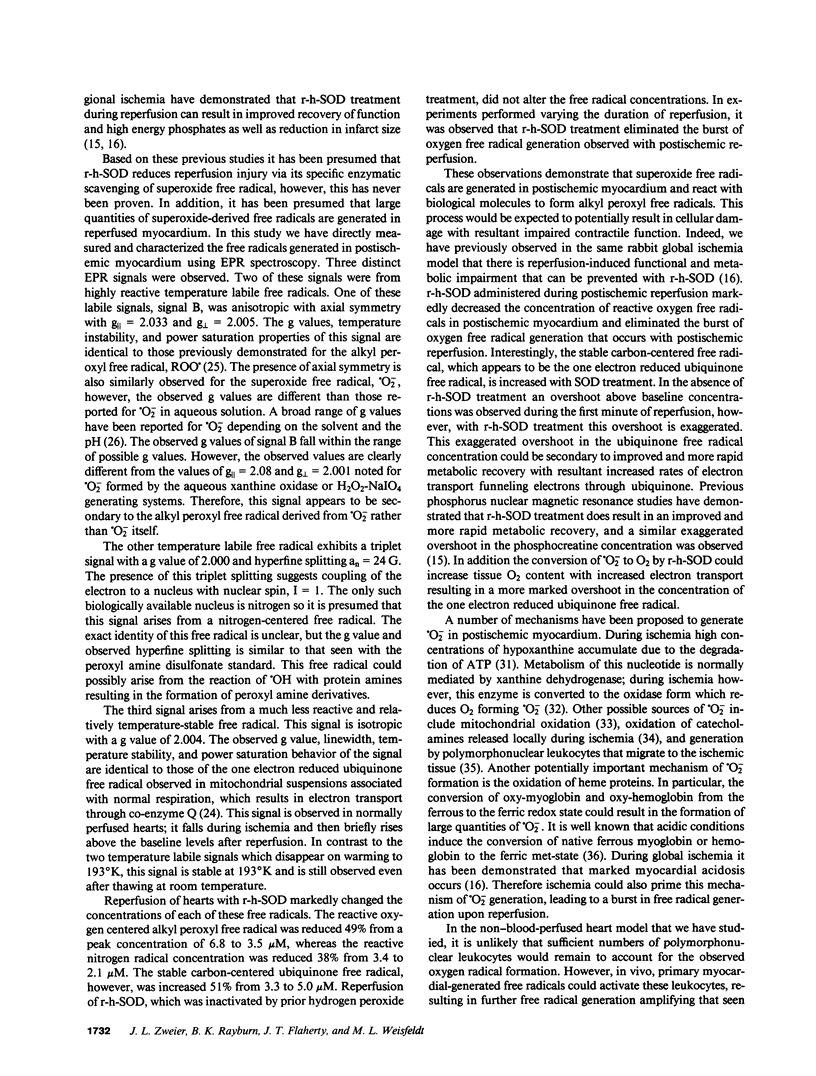
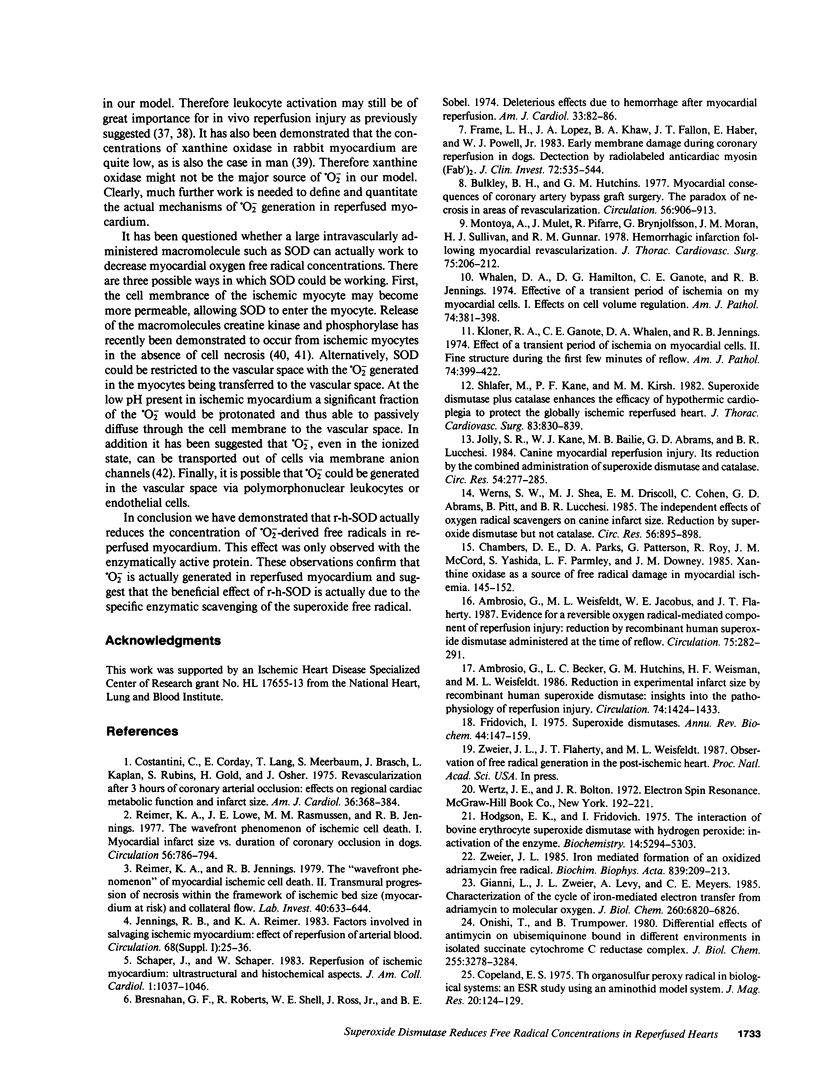
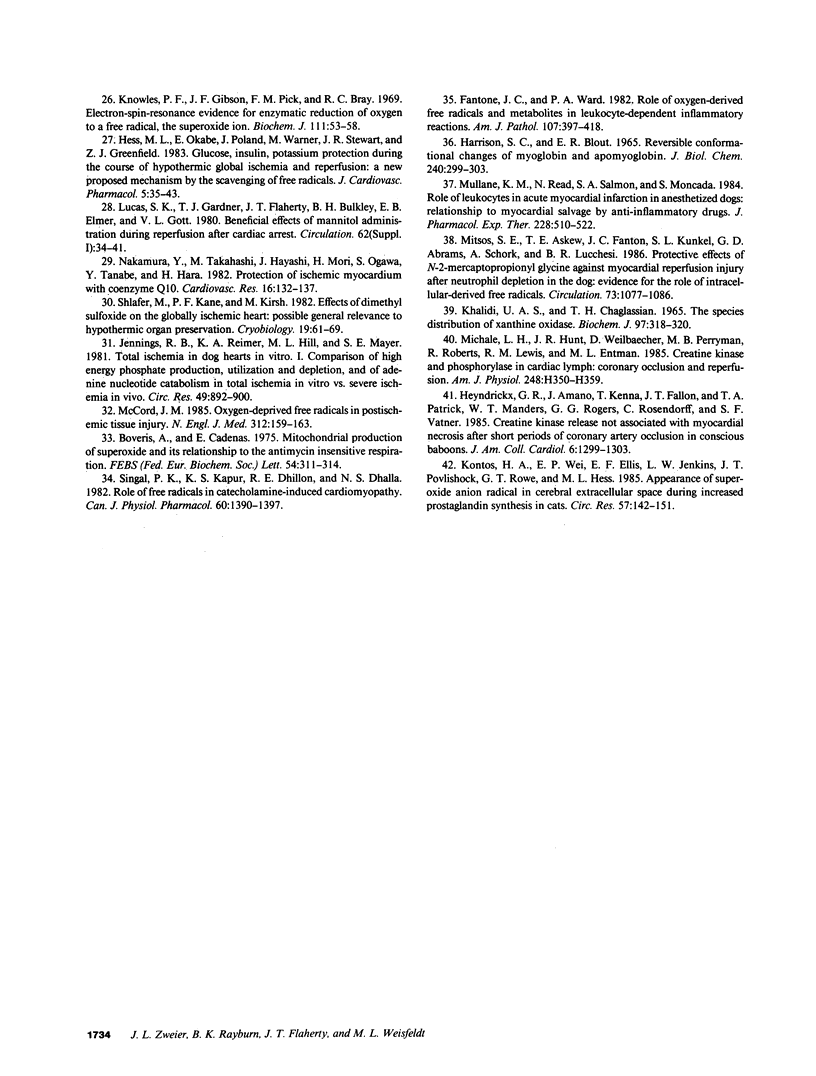
Images in this article
Selected References
These references are in PubMed. This may not be the complete list of references from this article.
- Al-Khalidi U. A., Chaglassian T. H. The species distribution of xanthine oxidase. Biochem J. 1965 Oct;97(1):318–320. doi: 10.1042/bj0970318. [DOI] [PMC free article] [PubMed] [Google Scholar]
- Ambrosio G., Becker L. C., Hutchins G. M., Weisman H. F., Weisfeldt M. L. Reduction in experimental infarct size by recombinant human superoxide dismutase: insights into the pathophysiology of reperfusion injury. Circulation. 1986 Dec;74(6):1424–1433. doi: 10.1161/01.cir.74.6.1424. [DOI] [PubMed] [Google Scholar]
- Ambrosio G., Weisfeldt M. L., Jacobus W. E., Flaherty J. T. Evidence for a reversible oxygen radical-mediated component of reperfusion injury: reduction by recombinant human superoxide dismutase administered at the time of reflow. Circulation. 1987 Jan;75(1):282–291. doi: 10.1161/01.cir.75.1.282. [DOI] [PubMed] [Google Scholar]
- Boveris A., Cadenas E. Mitochondrial production of superoxide anions and its relationship to the antimycin insensitive respiration. FEBS Lett. 1975 Jul 1;54(3):311–314. doi: 10.1016/0014-5793(75)80928-8. [DOI] [PubMed] [Google Scholar]
- Bresnahan G. F., Roberts R., Shell W. E., Ross J., Jr, Sobel B. E. Deleterious effects due to hemorrhage after myocardial reperfusion. Am J Cardiol. 1974 Jan;33(1):82–86. doi: 10.1016/0002-9149(74)90742-5. [DOI] [PubMed] [Google Scholar]
- Bulkely B. H., Hutchins G. M. Myocardial consequences of coronary artery bypass graft surgery. The paradox of necrosis in areas of revascularization. Circulation. 1977 Dec;56(6):906–913. doi: 10.1161/01.cir.56.6.906. [DOI] [PubMed] [Google Scholar]
- Chambers D. E., Parks D. A., Patterson G., Roy R., McCord J. M., Yoshida S., Parmley L. F., Downey J. M. Xanthine oxidase as a source of free radical damage in myocardial ischemia. J Mol Cell Cardiol. 1985 Feb;17(2):145–152. doi: 10.1016/s0022-2828(85)80017-1. [DOI] [PubMed] [Google Scholar]
- Costantino C., Corday E., Lang T. W., Meerbaum S., Brasch J., Kaplan L., Rubins S., Gold H., Osher J. Revascularization after 3 hours of coronary arterial occlusion: effects on regional cardiac metabolic function and infarct size. Am J Cardiol. 1975 Sep;36(3):368–384. doi: 10.1016/0002-9149(75)90492-0. [DOI] [PubMed] [Google Scholar]
- Fantone J. C., Ward P. A. Role of oxygen-derived free radicals and metabolites in leukocyte-dependent inflammatory reactions. Am J Pathol. 1982 Jun;107(3):395–418. [PMC free article] [PubMed] [Google Scholar]
- Frame L. H., Lopez J. A., Khaw B. A., Fallon J. T., Haber E., Powell W. J., Jr Early membrane damage during coronary reperfusion in dogs. Detection by radiolabeled anticardiac myosin (Fab')2. J Clin Invest. 1983 Aug;72(2):535–544. doi: 10.1172/JCI111001. [DOI] [PMC free article] [PubMed] [Google Scholar]
- Fridovich I. Superoxide dismutases. Annu Rev Biochem. 1975;44:147–159. doi: 10.1146/annurev.bi.44.070175.001051. [DOI] [PubMed] [Google Scholar]
- Gianni L., Zweier J. L., Levy A., Myers C. E. Characterization of the cycle of iron-mediated electron transfer from Adriamycin to molecular oxygen. J Biol Chem. 1985 Jun 10;260(11):6820–6826. [PubMed] [Google Scholar]
- HARRISON S. C., BLOUT E. R. REVERSIBLE CONFORMATIONAL CHANGES OF MYOGLOBIN AND APOMYOGLOBIN. J Biol Chem. 1965 Jan;240:299–303. [PubMed] [Google Scholar]
- Hess M. L., Okabe E., Poland J., Warner M., Stewart J. R., Greenfield L. J. Glucose, insulin, potassium protection during the course of hypothermic global ischemia and reperfusion: a new proposed mechanism by the scavenging of free radicals. J Cardiovasc Pharmacol. 1983 Jan-Feb;5(1):35–43. doi: 10.1097/00005344-198301000-00005. [DOI] [PubMed] [Google Scholar]
- Heyndrickx G. R., Amano J., Kenna T., Fallon J. T., Patrick T. A., Manders W. T., Rogers G. G., Rosendorff C., Vatner S. F. Creatine kinase release not associated with myocardial necrosis after short periods of coronary artery occlusion in conscious baboons. J Am Coll Cardiol. 1985 Dec;6(6):1299–1303. doi: 10.1016/s0735-1097(85)80216-3. [DOI] [PubMed] [Google Scholar]
- Hodgson E. K., Fridovich I. The interaction of bovine erythrocyte superoxide dismutase with hydrogen peroxide: inactivation of the enzyme. Biochemistry. 1975 Dec 2;14(24):5294–5299. doi: 10.1021/bi00695a010. [DOI] [PubMed] [Google Scholar]
- Jennings R. B., Reimer K. A., Hill M. L., Mayer S. E. Total ischemia in dog hearts, in vitro. 1. Comparison of high energy phosphate production, utilization, and depletion, and of adenine nucleotide catabolism in total ischemia in vitro vs. severe ischemia in vivo. Circ Res. 1981 Oct;49(4):892–900. doi: 10.1161/01.res.49.4.892. [DOI] [PubMed] [Google Scholar]
- Jolly S. R., Kane W. J., Bailie M. B., Abrams G. D., Lucchesi B. R. Canine myocardial reperfusion injury. Its reduction by the combined administration of superoxide dismutase and catalase. Circ Res. 1984 Mar;54(3):277–285. doi: 10.1161/01.res.54.3.277. [DOI] [PubMed] [Google Scholar]
- Kloner R. A., Ganote C. E., Whalen D. A., Jr, Jennings R. B. Effect of a transient period of ischemia on myocardial cells. II. Fine structure during the first few minutes of reflow. Am J Pathol. 1974 Mar;74(3):399–422. [PMC free article] [PubMed] [Google Scholar]
- Knowles P. F., Gibson J. F., Pick F. M., Bray R. C. Electron-spin-resonance evidence for enzymic reduction of oxygen to a free radical, the superoxide ion. Biochem J. 1969 Jan;111(1):53–58. doi: 10.1042/bj1110053. [DOI] [PMC free article] [PubMed] [Google Scholar]
- Kontos H. A., Wei E. P., Ellis E. F., Jenkins L. W., Povlishock J. T., Rowe G. T., Hess M. L. Appearance of superoxide anion radical in cerebral extracellular space during increased prostaglandin synthesis in cats. Circ Res. 1985 Jul;57(1):142–151. doi: 10.1161/01.res.57.1.142. [DOI] [PubMed] [Google Scholar]
- McCord J. M. Oxygen-derived free radicals in postischemic tissue injury. N Engl J Med. 1985 Jan 17;312(3):159–163. doi: 10.1056/NEJM198501173120305. [DOI] [PubMed] [Google Scholar]
- Michael L. H., Hunt J. R., Weilbaecher D., Perryman M. B., Roberts R., Lewis R. M., Entman M. L. Creatine kinase and phosphorylase in cardiac lymph: coronary occlusion and reperfusion. Am J Physiol. 1985 Mar;248(3 Pt 2):H350–H359. doi: 10.1152/ajpheart.1985.248.3.H350. [DOI] [PubMed] [Google Scholar]
- Mitsos S. E., Askew T. E., Fantone J. C., Kunkel S. L., Abrams G. D., Schork A., Lucchesi B. R. Protective effects of N-2-mercaptopropionyl glycine against myocardial reperfusion injury after neutrophil depletion in the dog: evidence for the role of intracellular-derived free radicals. Circulation. 1986 May;73(5):1077–1086. doi: 10.1161/01.cir.73.5.1077. [DOI] [PubMed] [Google Scholar]
- Montoya A., Mulet J., Pifarré R., Brynjolfsson G., Moran J. M., Sullivan H. J., Gunnar R. M. Hemorrhagic infarct following myocardial revascularization. J Thorac Cardiovasc Surg. 1978 Feb;75(2):206–212. [PubMed] [Google Scholar]
- Mullane K. M., Read N., Salmon J. A., Moncada S. Role of leukocytes in acute myocardial infarction in anesthetized dogs: relationship to myocardial salvage by anti-inflammatory drugs. J Pharmacol Exp Ther. 1984 Feb;228(2):510–522. [PubMed] [Google Scholar]
- Nakamura Y., Takahashi M., Hayashi J., Mori H., Ogawa S., Tanabe Y., Hara K. Protection of ischaemic myocardium with coenzyme Q10. Cardiovasc Res. 1982 Mar;16(3):132–137. doi: 10.1093/cvr/16.3.132. [DOI] [PubMed] [Google Scholar]
- Ohnishi T., Trumpower B. L. Differential effects of antimycin on ubisemiquinone bound in different environments in isolated succinate . cytochrome c reductase complex. J Biol Chem. 1980 Apr 25;255(8):3278–3284. [PubMed] [Google Scholar]
- Reimer K. A., Jennings R. B. The "wavefront phenomenon" of myocardial ischemic cell death. II. Transmural progression of necrosis within the framework of ischemic bed size (myocardium at risk) and collateral flow. Lab Invest. 1979 Jun;40(6):633–644. [PubMed] [Google Scholar]
- Reimer K. A., Lowe J. E., Rasmussen M. M., Jennings R. B. The wavefront phenomenon of ischemic cell death. 1. Myocardial infarct size vs duration of coronary occlusion in dogs. Circulation. 1977 Nov;56(5):786–794. doi: 10.1161/01.cir.56.5.786. [DOI] [PubMed] [Google Scholar]
- Schaper J., Schaper W. Reperfusion of ischemic myocardium: ultrastructural and histochemical aspects. J Am Coll Cardiol. 1983 Apr;1(4):1037–1046. doi: 10.1016/s0735-1097(83)80106-5. [DOI] [PubMed] [Google Scholar]
- Shlafer M., Kane P. F., Kirsh M. M. Effects of dimethyl sulfoxide on the globally ischemic heart: possible general relevance to hypothermic organ preservation. Cryobiology. 1982 Feb;19(1):61–69. doi: 10.1016/0011-2240(82)90125-0. [DOI] [PubMed] [Google Scholar]
- Shlafer M., Kane P. F., Kirsh M. M. Superoxide dismutase plus catalase enhances the efficacy of hypothermic cardioplegia to protect the globally ischemic, reperfused heart. J Thorac Cardiovasc Surg. 1982 Jun;83(6):830–839. [PubMed] [Google Scholar]
- Singal P. K., Kapur N., Dhillon K. S., Beamish R. E., Dhalla N. S. Role of free radicals in catecholamine-induced cardiomyopathy. Can J Physiol Pharmacol. 1982 Nov;60(11):1390–1397. doi: 10.1139/y82-207. [DOI] [PubMed] [Google Scholar]
- Werns S. W., Shea M. J., Driscoll E. M., Cohen C., Abrams G. D., Pitt B., Lucchesi B. R. The independent effects of oxygen radical scavengers on canine infarct size. Reduction by superoxide dismutase but not catalase. Circ Res. 1985 Jun;56(6):895–898. doi: 10.1161/01.res.56.6.895. [DOI] [PubMed] [Google Scholar]
- Whalen D. A., Jr, Hamilton D. G., Ganote C. E., Jennings R. B. Effect of a transient period of ischemia on myocardial cells. I. Effects on cell volume regulation. Am J Pathol. 1974 Mar;74(3):381–397. [PMC free article] [PubMed] [Google Scholar]
- Zweier J. L. Iron-mediated formation of an oxidized adriamycin free radical. Biochim Biophys Acta. 1985 Apr 17;839(2):209–213. doi: 10.1016/0304-4165(85)90038-8. [DOI] [PubMed] [Google Scholar]