Abstract
The stress in the endothelial cells induced by blood flow depends on the waviness of the blood-endothelium interface and the slopes at the junctions of neighboring cells in the direction of flow. The height and slope in the third dimension of the living endothelial cells cannot be measured by ordinary optical and electron microscopy. Here we show that interference microscopy meets the challenge. We measured the geometry of cultured confluent human vascular endothelial cells in a flow, and we found that in a normal section parallel to the flow, the absolute values of the surface slopes at the cell junctions were 0.70 +/- 0.02 (SE) and 0.80 +/- 0.02 (SE) at the leading and trailing edges of the cells, respectively, in a culture medium of osmolarity 310 mosM with a shear stress of approximately 1 N/m2. A reversal of the flow direction led to a reversal of the slope pattern. An increase in medium osmolarity above 310 mosM induced an initial decrease in the slopes followed by a return to normal, whereas a decrease in the osmolarity had a reversed effect. These results, in light of our previous theoretical analyses, show that tensile stress exists in the endothelial cell membrane, and that the mechanism of tension accumulation is a reality. The accumulation is not 100% because the membranes are not smooth at the cell junctions.
Full text
PDF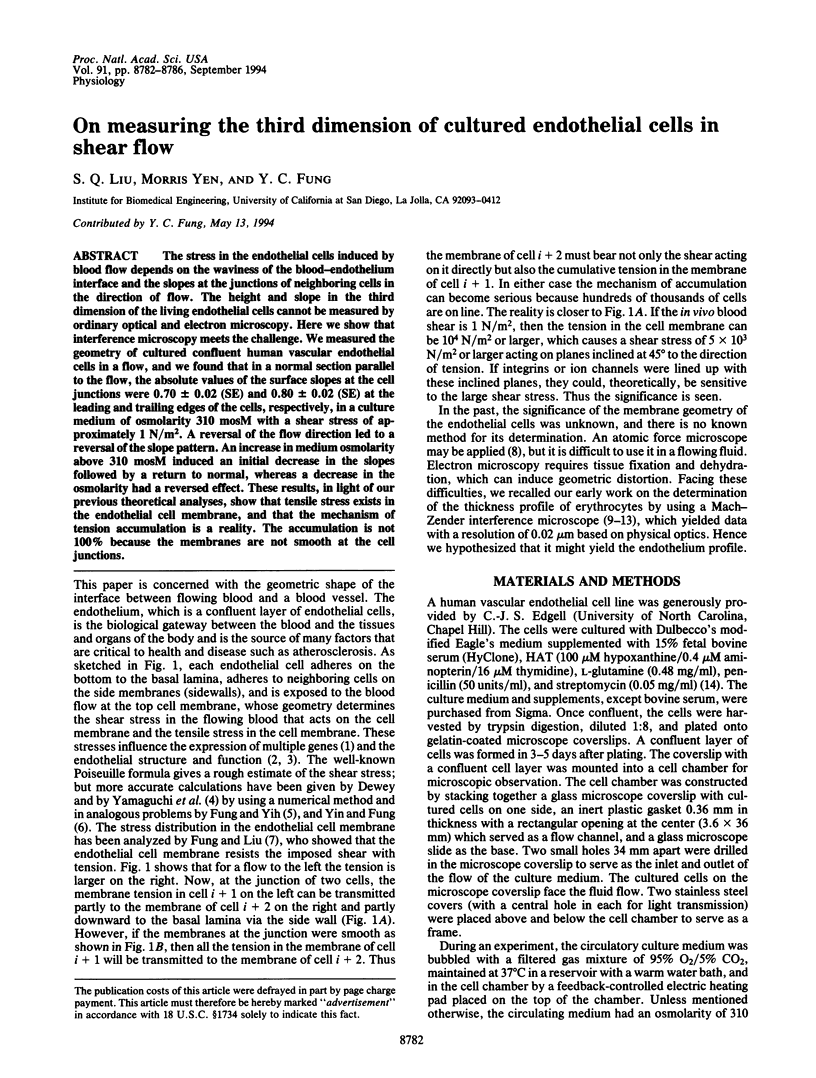
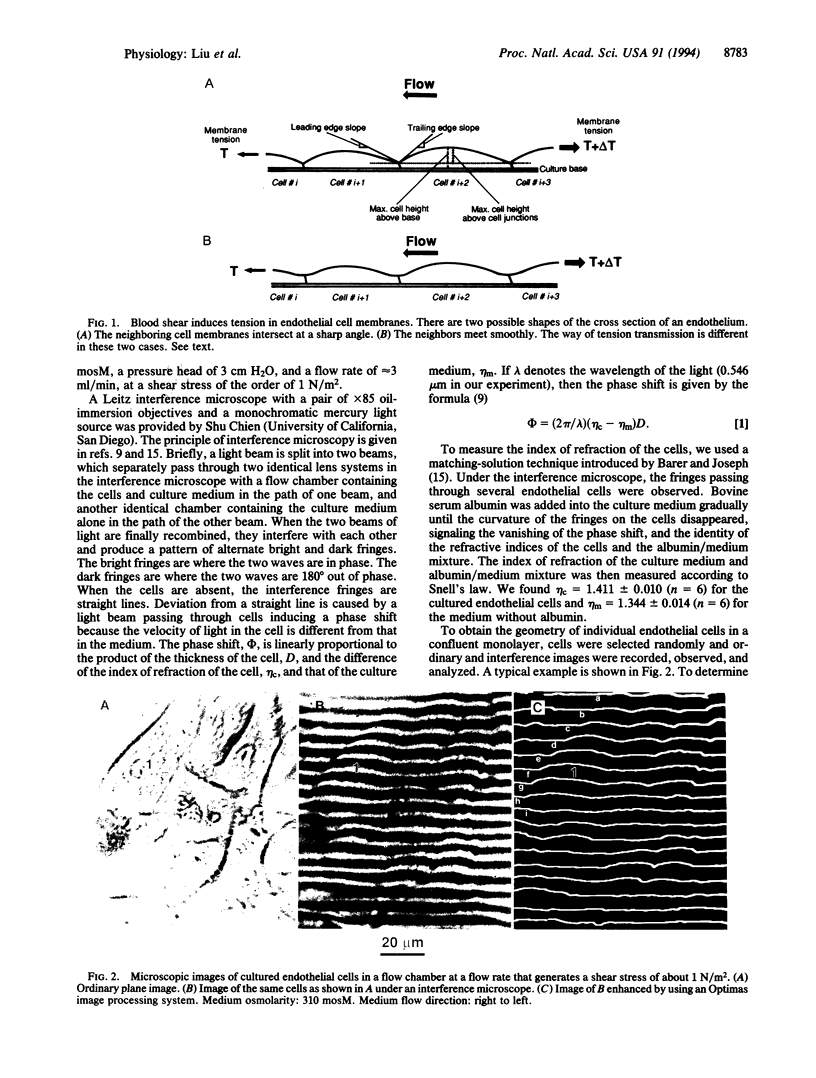
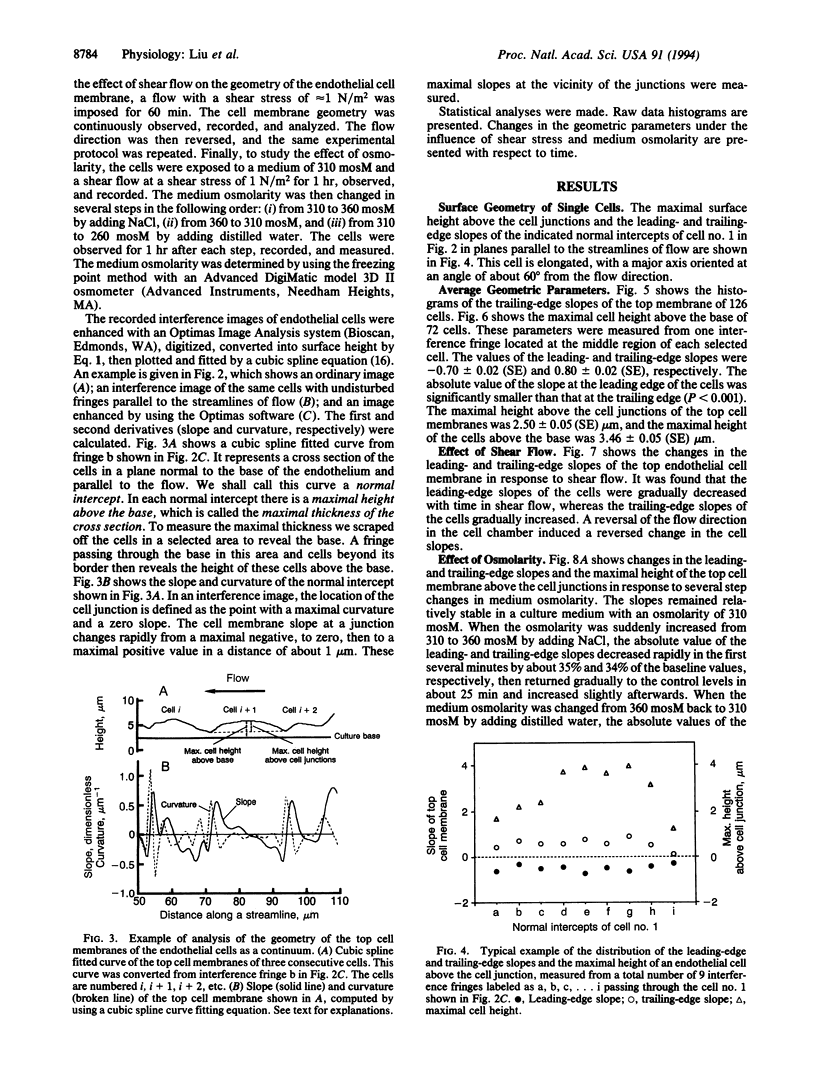
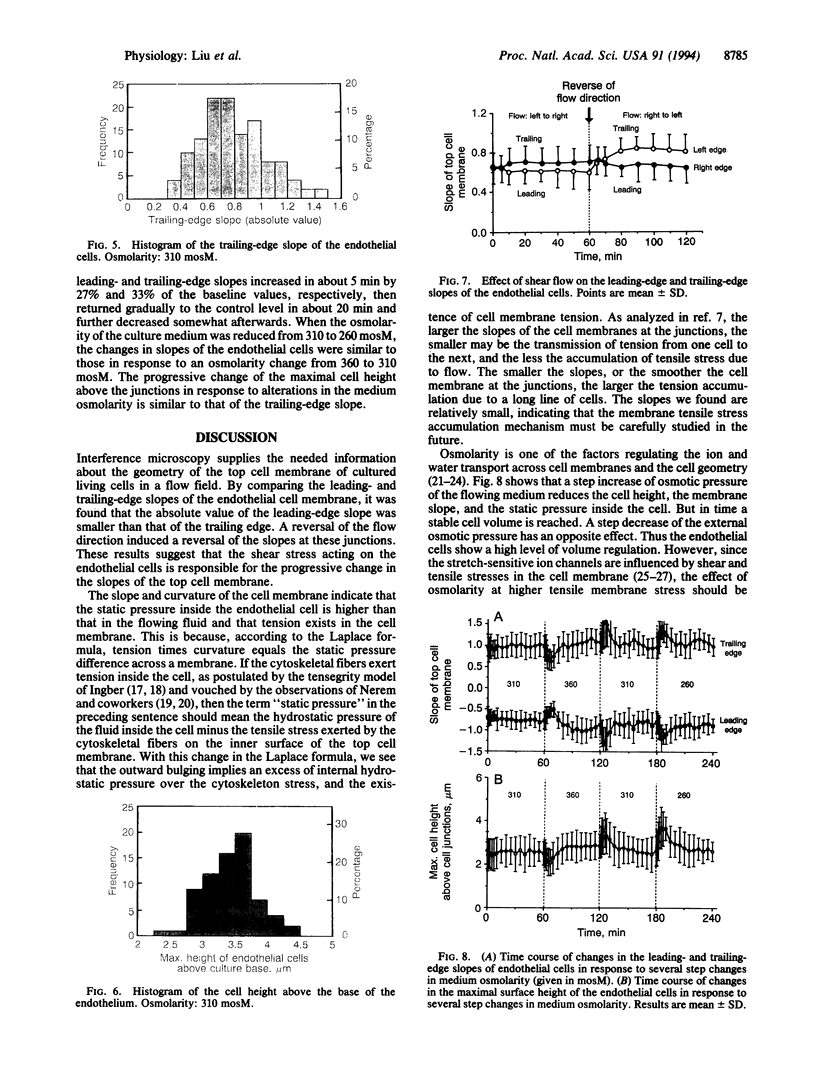
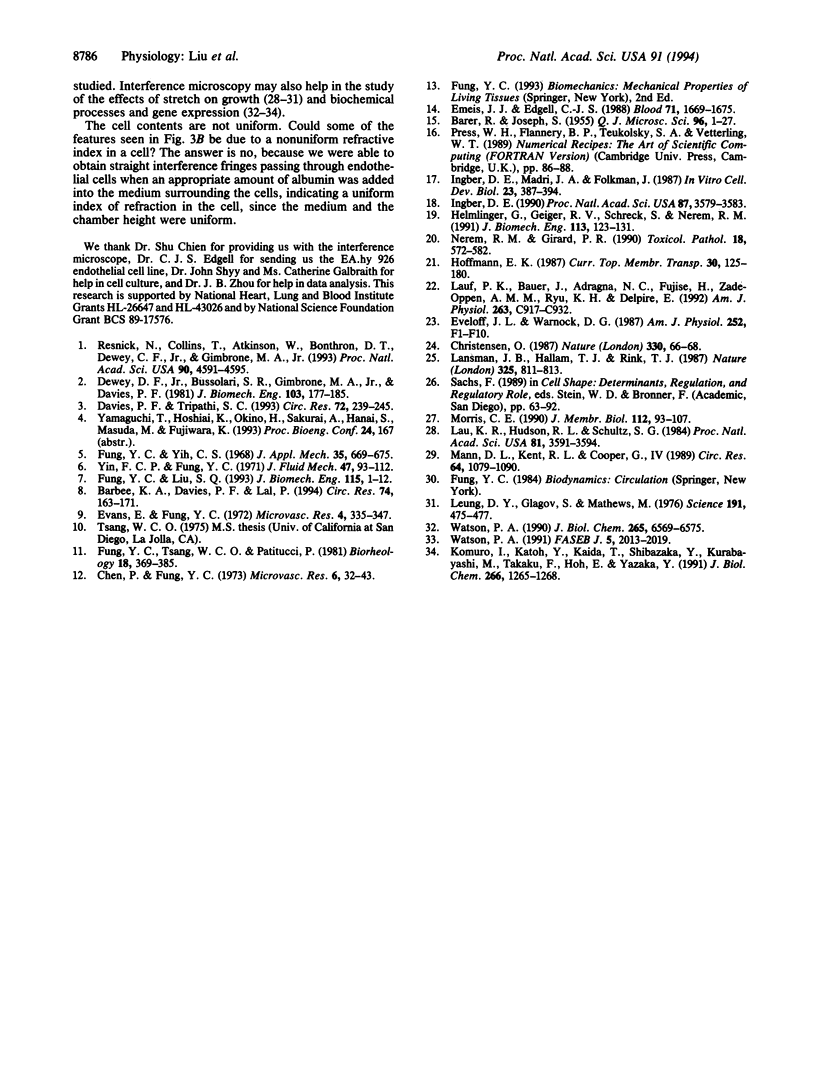
Images in this article
Selected References
These references are in PubMed. This may not be the complete list of references from this article.
- Barbee K. A., Davies P. F., Lal R. Shear stress-induced reorganization of the surface topography of living endothelial cells imaged by atomic force microscopy. Circ Res. 1994 Jan;74(1):163–171. doi: 10.1161/01.res.74.1.163. [DOI] [PubMed] [Google Scholar]
- Chen P. C., Fung Y. C. Extreme-value statistics of human red blood cells. Microvasc Res. 1973 Jul;6(1):32–43. doi: 10.1016/0026-2862(73)90004-6. [DOI] [PubMed] [Google Scholar]
- Christensen O. Mediation of cell volume regulation by Ca2+ influx through stretch-activated channels. Nature. 1987 Nov 5;330(6143):66–68. doi: 10.1038/330066a0. [DOI] [PubMed] [Google Scholar]
- Davies P. F., Tripathi S. C. Mechanical stress mechanisms and the cell. An endothelial paradigm. Circ Res. 1993 Feb;72(2):239–245. doi: 10.1161/01.res.72.2.239. [DOI] [PubMed] [Google Scholar]
- Dewey C. F., Jr, Bussolari S. R., Gimbrone M. A., Jr, Davies P. F. The dynamic response of vascular endothelial cells to fluid shear stress. J Biomech Eng. 1981 Aug;103(3):177–185. doi: 10.1115/1.3138276. [DOI] [PubMed] [Google Scholar]
- Emeis J. J., Edgell C. J. Fibrinolytic properties of a human endothelial hybrid cell line (Ea.hy 926). Blood. 1988 Jun;71(6):1669–1675. [PubMed] [Google Scholar]
- Evans E., Fung Y. C. Improved measurements of the erythrocyte geometry. Microvasc Res. 1972 Oct;4(4):335–347. doi: 10.1016/0026-2862(72)90069-6. [DOI] [PubMed] [Google Scholar]
- Eveloff J. L., Warnock D. G. Activation of ion transport systems during cell volume regulation. Am J Physiol. 1987 Jan;252(1 Pt 2):F1–10. doi: 10.1152/ajprenal.1987.252.1.F1. [DOI] [PubMed] [Google Scholar]
- Fung Y. C., Liu S. Q. Elementary mechanics of the endothelium of blood vessels. J Biomech Eng. 1993 Feb;115(1):1–12. doi: 10.1115/1.2895465. [DOI] [PubMed] [Google Scholar]
- Fung Y. C., Tsang W. C., Patitucci P. High-resolution data on the geometry of red blood cells. Biorheology. 1981;18(3-6):369–385. doi: 10.3233/bir-1981-183-606. [DOI] [PubMed] [Google Scholar]
- Helmlinger G., Geiger R. V., Schreck S., Nerem R. M. Effects of pulsatile flow on cultured vascular endothelial cell morphology. J Biomech Eng. 1991 May;113(2):123–131. doi: 10.1115/1.2891226. [DOI] [PubMed] [Google Scholar]
- Ingber D. E. Fibronectin controls capillary endothelial cell growth by modulating cell shape. Proc Natl Acad Sci U S A. 1990 May;87(9):3579–3583. doi: 10.1073/pnas.87.9.3579. [DOI] [PMC free article] [PubMed] [Google Scholar]
- Ingber D. E., Madri J. A., Folkman J. Endothelial growth factors and extracellular matrix regulate DNA synthesis through modulation of cell and nuclear expansion. In Vitro Cell Dev Biol. 1987 May;23(5):387–394. doi: 10.1007/BF02620997. [DOI] [PubMed] [Google Scholar]
- Komuro I., Katoh Y., Kaida T., Shibazaki Y., Kurabayashi M., Hoh E., Takaku F., Yazaki Y. Mechanical loading stimulates cell hypertrophy and specific gene expression in cultured rat cardiac myocytes. Possible role of protein kinase C activation. J Biol Chem. 1991 Jan 15;266(2):1265–1268. [PubMed] [Google Scholar]
- Lansman J. B., Hallam T. J., Rink T. J. Single stretch-activated ion channels in vascular endothelial cells as mechanotransducers? 1987 Feb 26-Mar 4Nature. 325(6107):811–813. doi: 10.1038/325811a0. [DOI] [PubMed] [Google Scholar]
- Lau K. R., Hudson R. L., Schultz S. G. Cell swelling increases a barium-inhibitable potassium conductance in the basolateral membrane of Necturus small intestine. Proc Natl Acad Sci U S A. 1984 Jun;81(11):3591–3594. doi: 10.1073/pnas.81.11.3591. [DOI] [PMC free article] [PubMed] [Google Scholar]
- Lauf P. K., Bauer J., Adragna N. C., Fujise H., Zade-Oppen A. M., Ryu K. H., Delpire E. Erythrocyte K-Cl cotransport: properties and regulation. Am J Physiol. 1992 Nov;263(5 Pt 1):C917–C932. doi: 10.1152/ajpcell.1992.263.5.C917. [DOI] [PubMed] [Google Scholar]
- Leung D. Y., Glagov S., Mathews M. B. Cyclic stretching stimulates synthesis of matrix components by arterial smooth muscle cells in vitro. Science. 1976 Feb 6;191(4226):475–477. doi: 10.1126/science.128820. [DOI] [PubMed] [Google Scholar]
- Mann D. L., Kent R. L., Cooper G., 4th Load regulation of the properties of adult feline cardiocytes: growth induction by cellular deformation. Circ Res. 1989 Jun;64(6):1079–1090. doi: 10.1161/01.res.64.6.1079. [DOI] [PubMed] [Google Scholar]
- Morris C. E. Mechanosensitive ion channels. J Membr Biol. 1990 Feb;113(2):93–107. doi: 10.1007/BF01872883. [DOI] [PubMed] [Google Scholar]
- Nerem R. M., Girard P. R. Hemodynamic influences on vascular endothelial biology. Toxicol Pathol. 1990;18(4 Pt 1):572–582. [PubMed] [Google Scholar]
- Resnick N., Collins T., Atkinson W., Bonthron D. T., Dewey C. F., Jr, Gimbrone M. A., Jr Platelet-derived growth factor B chain promoter contains a cis-acting fluid shear-stress-responsive element. Proc Natl Acad Sci U S A. 1993 May 15;90(10):4591–4595. doi: 10.1073/pnas.90.10.4591. [DOI] [PMC free article] [PubMed] [Google Scholar]
- Watson P. A. Direct stimulation of adenylate cyclase by mechanical forces in S49 mouse lymphoma cells during hyposmotic swelling. J Biol Chem. 1990 Apr 25;265(12):6569–6575. [PubMed] [Google Scholar]
- Watson P. A. Function follows form: generation of intracellular signals by cell deformation. FASEB J. 1991 Apr;5(7):2013–2019. doi: 10.1096/fasebj.5.7.1707019. [DOI] [PubMed] [Google Scholar]