Abstract
We extend the technique of fluorescence resonance energy transfer (FRET) by introducing a luminescent terbium chelate as a donor and an organic dye, tetramethylrhodamine, as an acceptor. The results are consistent with a Förster theory of energy transfer, provided the appropriate parameters are used. The use of lanthanide donors, in general, and this pair, in particular, has many advantages over more conventional FRET pairs, which rely solely on organic dyes. The distance at which 50% energy transfer occurs is large, 65 A; the donor lifetime is a single exponential and long (millisecond), making lifetime measurements facile and accurate. Uncertainty in the orientation factor, which creates uncertainty in measured distances, is minimized by the donor's multiple electronic transitions and long lifetime. The sensitized emission of the acceptor can be measured with little or no interfering background, yielding a > 25-fold improvements in the signal-to-background ratio over standard donor-acceptor pairs. These improvements are expected to make distances > 100 A measurable via FRET. We also report measurement of the sensitized emission lifetime, a measurement that is completely insensitive to total concentration and incomplete labeling.
Full text
PDF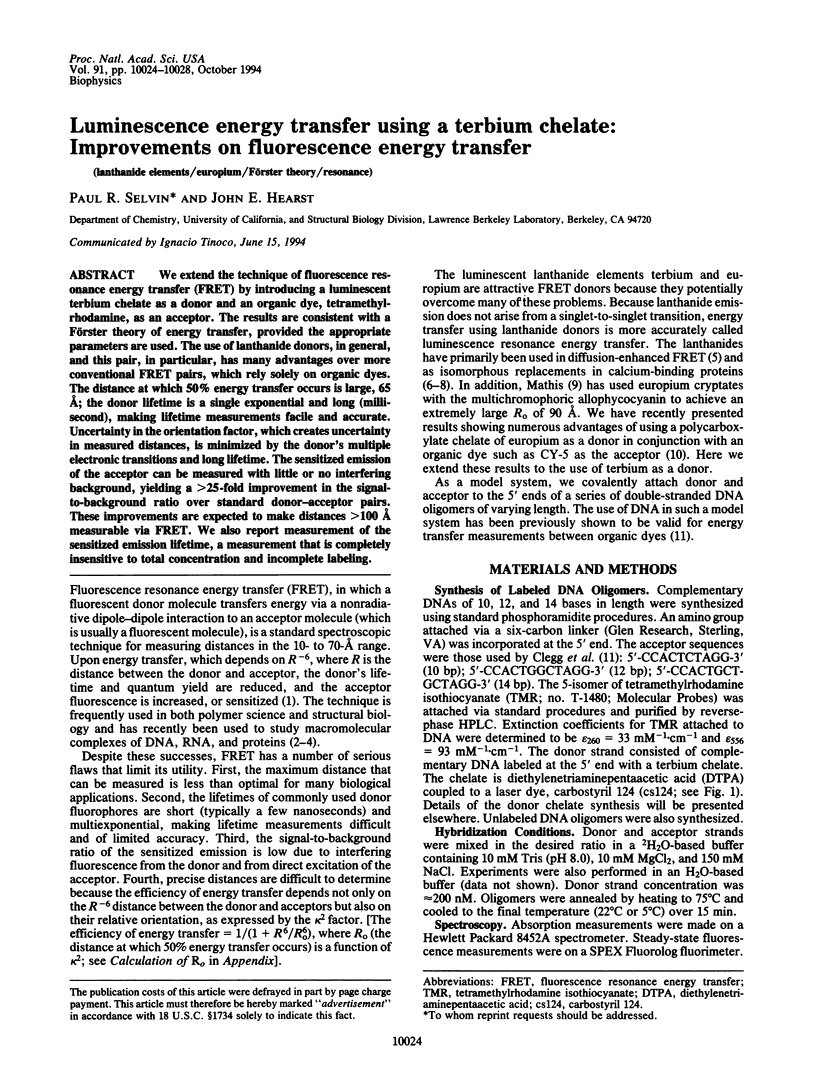
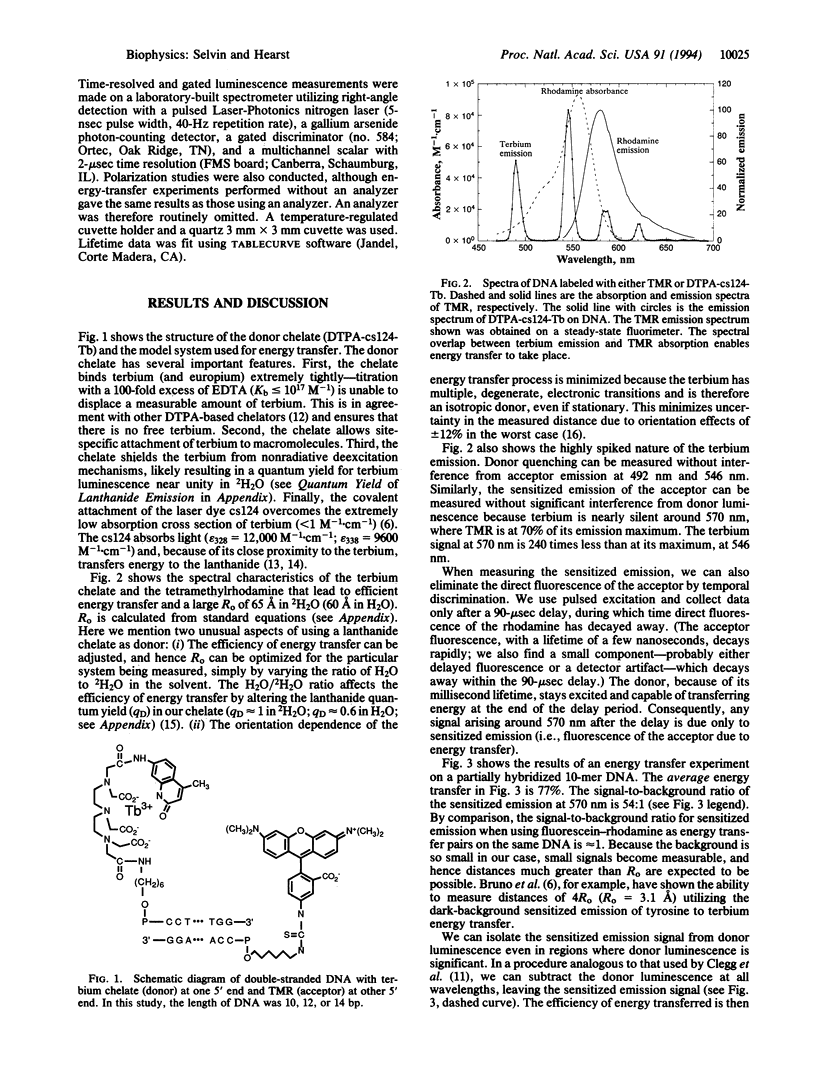
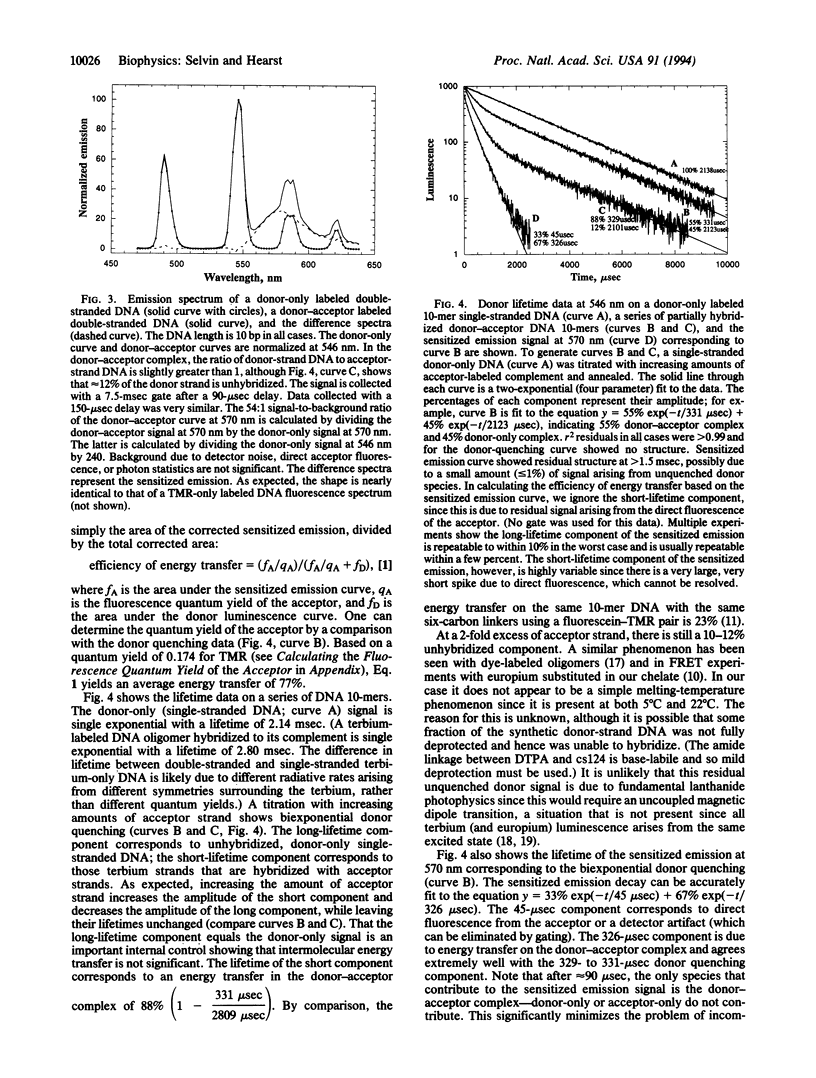
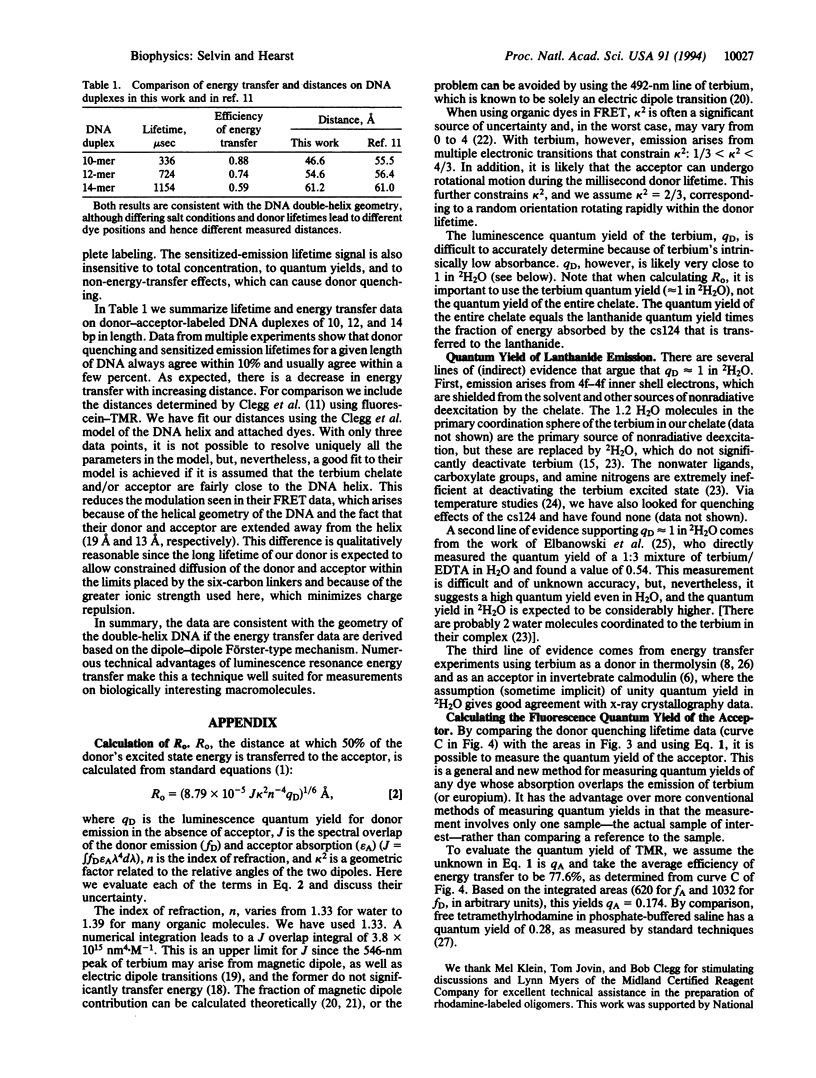
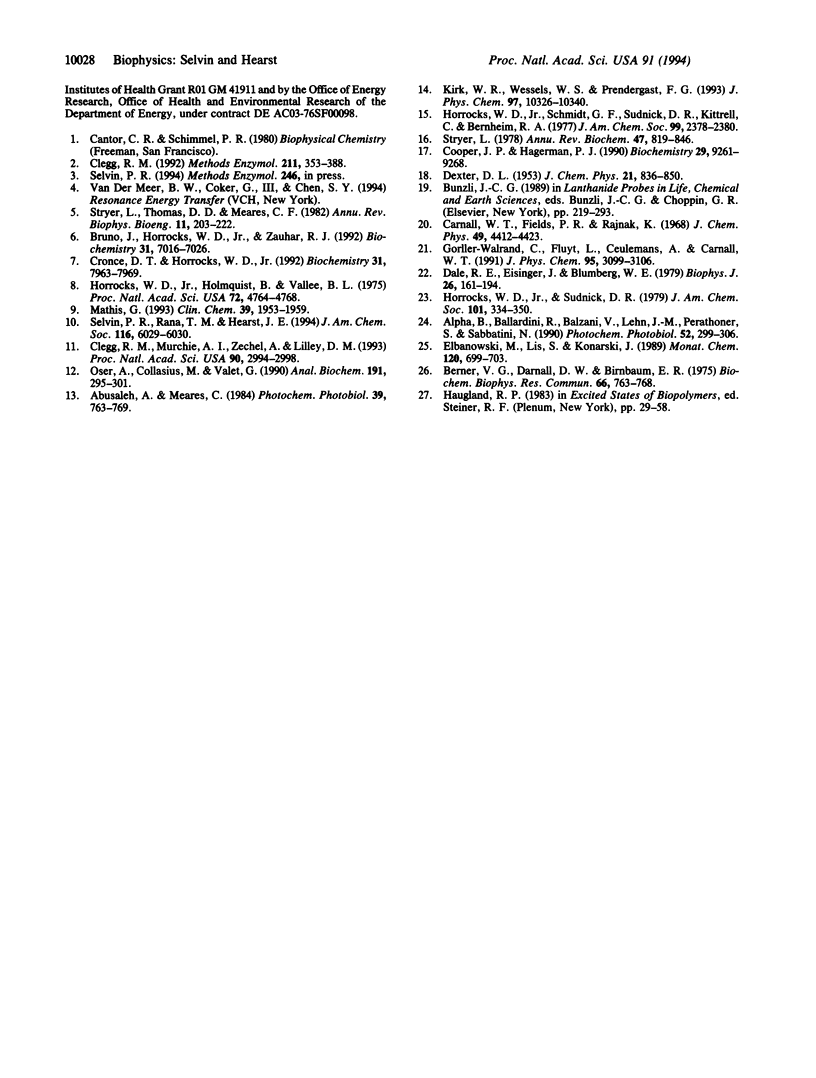
Selected References
These references are in PubMed. This may not be the complete list of references from this article.
- Abusaleh A., Meares C. F. Excitation and de-excitation processes in lanthanide chelates bearing aromatic sidechains. Photochem Photobiol. 1984 Jun;39(6):763–769. doi: 10.1111/j.1751-1097.1984.tb08856.x. [DOI] [PubMed] [Google Scholar]
- Berner V. G., Darnall D. W., Birnbaum E. R. Distance measurements between the metal-binding sites in thermolysin using terbium ion as a fluorescent probe. Biochem Biophys Res Commun. 1975 Sep 16;66(2):763–768. doi: 10.1016/0006-291x(75)90575-6. [DOI] [PubMed] [Google Scholar]
- Bruno J., Horrocks W. D., Jr, Zauhar R. J. Europium(III) luminescence and tyrosine to terbium(III) energy-transfer studies of invertebrate (octopus) calmodulin. Biochemistry. 1992 Aug 11;31(31):7016–7026. doi: 10.1021/bi00146a002. [DOI] [PubMed] [Google Scholar]
- Clegg R. M. Fluorescence resonance energy transfer and nucleic acids. Methods Enzymol. 1992;211:353–388. doi: 10.1016/0076-6879(92)11020-j. [DOI] [PubMed] [Google Scholar]
- Clegg R. M., Murchie A. I., Zechel A., Lilley D. M. Observing the helical geometry of double-stranded DNA in solution by fluorescence resonance energy transfer. Proc Natl Acad Sci U S A. 1993 Apr 1;90(7):2994–2998. doi: 10.1073/pnas.90.7.2994. [DOI] [PMC free article] [PubMed] [Google Scholar]
- Cooper J. P., Hagerman P. J. Analysis of fluorescence energy transfer in duplex and branched DNA molecules. Biochemistry. 1990 Oct 2;29(39):9261–9268. doi: 10.1021/bi00491a022. [DOI] [PubMed] [Google Scholar]
- Cronce D. T., Horrocks W. D., Jr Probing the metal-binding sites of cod parvalbumin using europium(III) ion luminescence and diffusion-enhanced energy transfer. Biochemistry. 1992 Sep 1;31(34):7963–7969. doi: 10.1021/bi00149a030. [DOI] [PubMed] [Google Scholar]
- Dale R. E., Eisinger J., Blumberg W. E. The orientational freedom of molecular probes. The orientation factor in intramolecular energy transfer. Biophys J. 1979 May;26(2):161–193. doi: 10.1016/S0006-3495(79)85243-1. [DOI] [PMC free article] [PubMed] [Google Scholar]
- Horrocks W. D., Jr, Holmquist B., Vallee B. L. Energy transfer between terbium (III) and cobalt (II) in thermolysin: a new class of metal--metal distance probes. Proc Natl Acad Sci U S A. 1975 Dec;72(12):4764–4768. doi: 10.1073/pnas.72.12.4764. [DOI] [PMC free article] [PubMed] [Google Scholar]
- Horrocks W. D., Jr, Schmidt G. F., Sudnick D. R., Kittrell C., Bernheim R. A. Laser-induced lanthanide ion laminescence lifetime measurements by direct excitation of metal ion levels. A new class of structural probe for calcium-binding proteins and nucleic acids. J Am Chem Soc. 1977 Mar 30;99(7):2378–2380. doi: 10.1021/ja00449a079. [DOI] [PubMed] [Google Scholar]
- Mathis G. Rare earth cryptates and homogeneous fluoroimmunoassays with human sera. Clin Chem. 1993 Sep;39(9):1953–1959. [PubMed] [Google Scholar]
- Oser A., Collasius M., Valet G. Multiple end labeling of oligonucleotides with terbium chelate-substituted psoralen for time-resolved fluorescence detection. Anal Biochem. 1990 Dec;191(2):295–301. doi: 10.1016/0003-2697(90)90222-u. [DOI] [PubMed] [Google Scholar]
- Stryer L. Fluorescence energy transfer as a spectroscopic ruler. Annu Rev Biochem. 1978;47:819–846. doi: 10.1146/annurev.bi.47.070178.004131. [DOI] [PubMed] [Google Scholar]
- Stryer L., Thomas D. D., Meares C. F. Diffusion-enhanced fluorescence energy transfer. Annu Rev Biophys Bioeng. 1982;11:203–222. doi: 10.1146/annurev.bb.11.060182.001223. [DOI] [PubMed] [Google Scholar]