Abstract
In addition to ion-pumping ATPases, most plasma membranes of animal cells contain a Mg2+ ATPase activity, the function of which is unknown. This enzyme, of apparent molecular mass 110 kDa, was purified from human erythrocyte membranes by a series of column chromatographic procedures after solubilization in Triton X-100. When reincorporated into artificial bilayers formed from phosphatidylcholine, it was able to transport a spin-labeled phosphatidylserine analogue from the inner to the outer membrane leaflet provided Mg2+ ATP was present in the incubation mixture. The ATP-dependent transport of the phosphatidylethanolamine analogue required the presence of an anionic phospholipid (e.g., phosphatidylinositol) in the outer membrane leaflet. In contrast the transmembrane distribution of spin-labeled phosphatidylcholine was unaffected in the same experimental conditions. This transmembrane movement of aminophospholipid analogues was inhibited by treatment of the proteoliposomes with a sulfhydryl reagent. We conclude that the Mg2+ ATPase is sufficient for the biochemical expression of the aminophospholipid translocase activity, which is responsible for the inward transport of phosphatidylserine and phosphatidylethanolamine within the erythrocyte membrane. The presence of this transport activity in many animal cell plasma membranes provides a function for the Mg2+ ATPase borne by these membranes.
Full text
PDF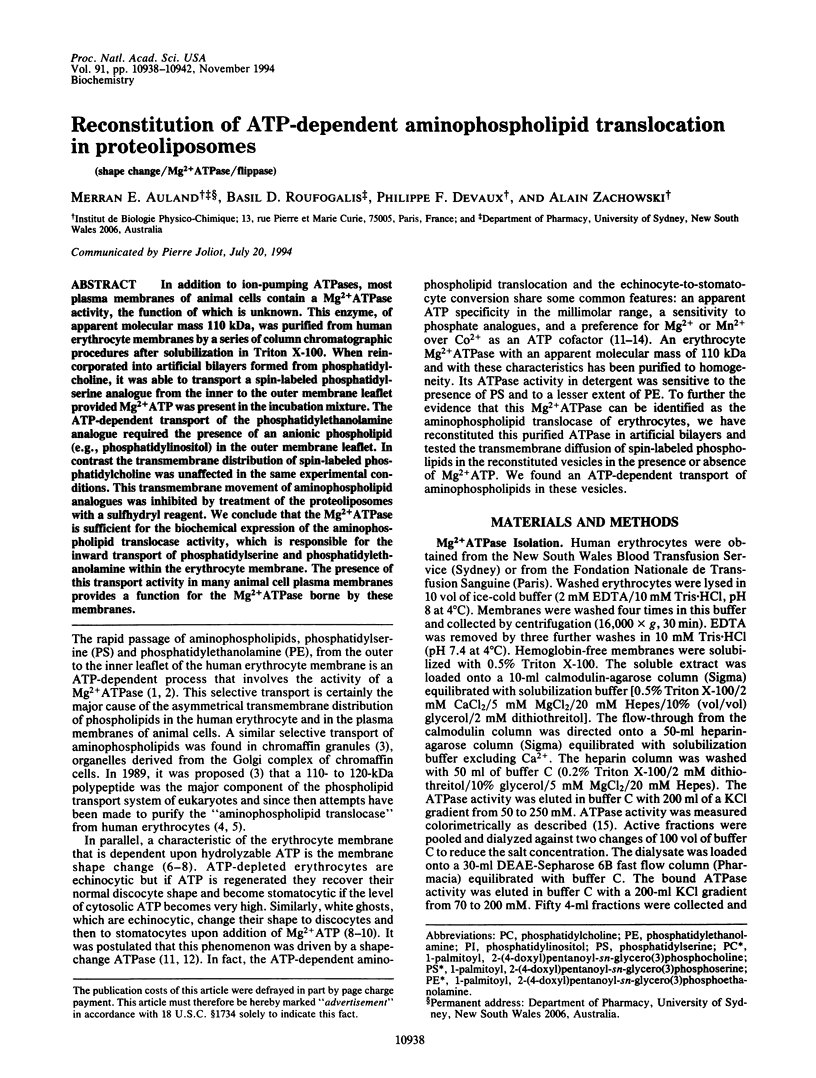
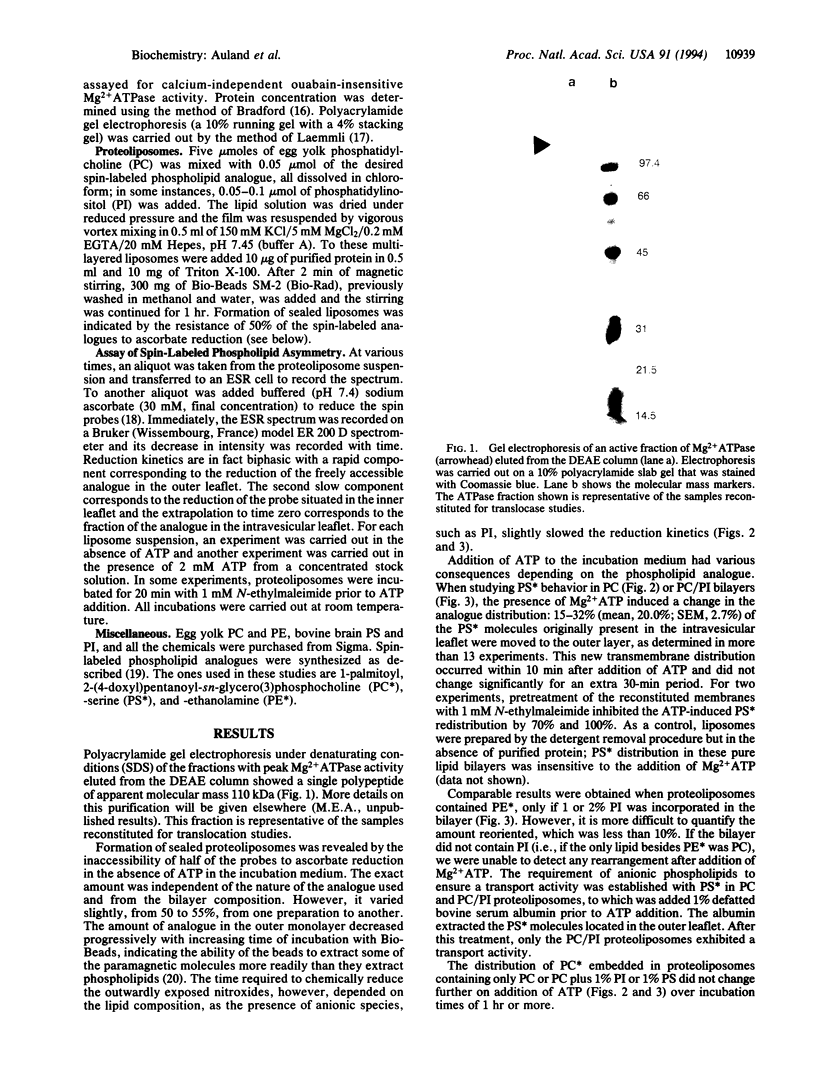
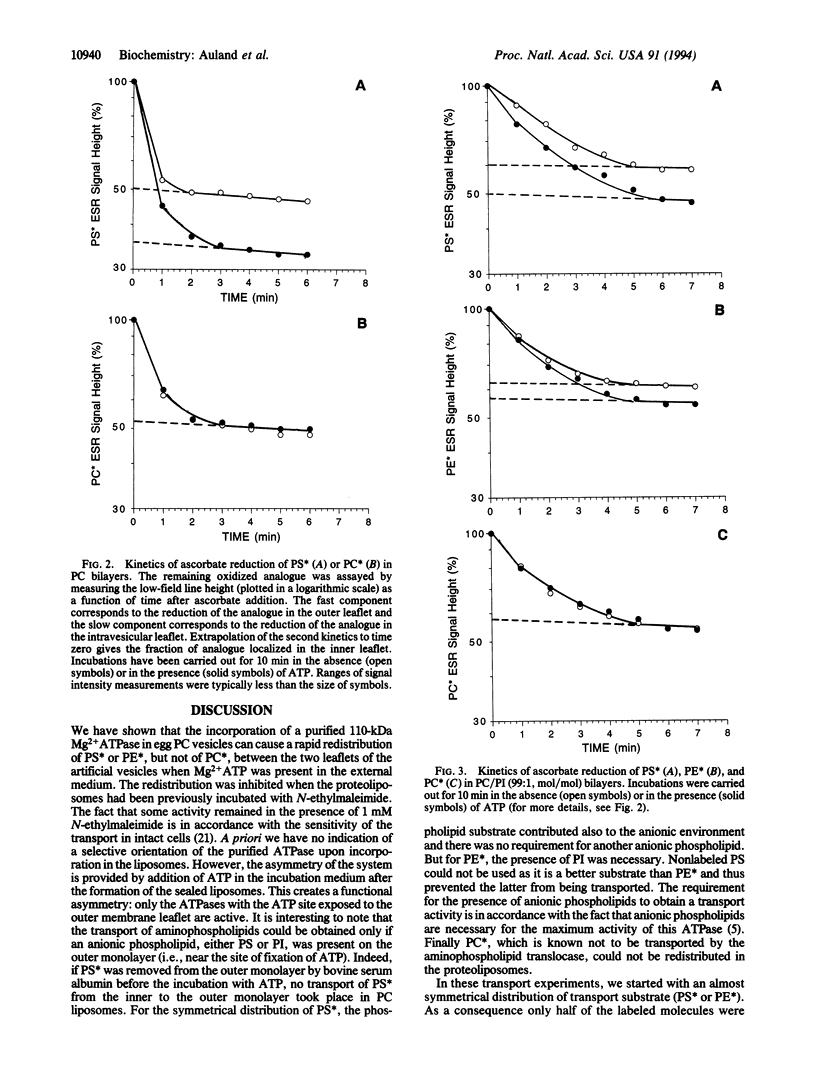
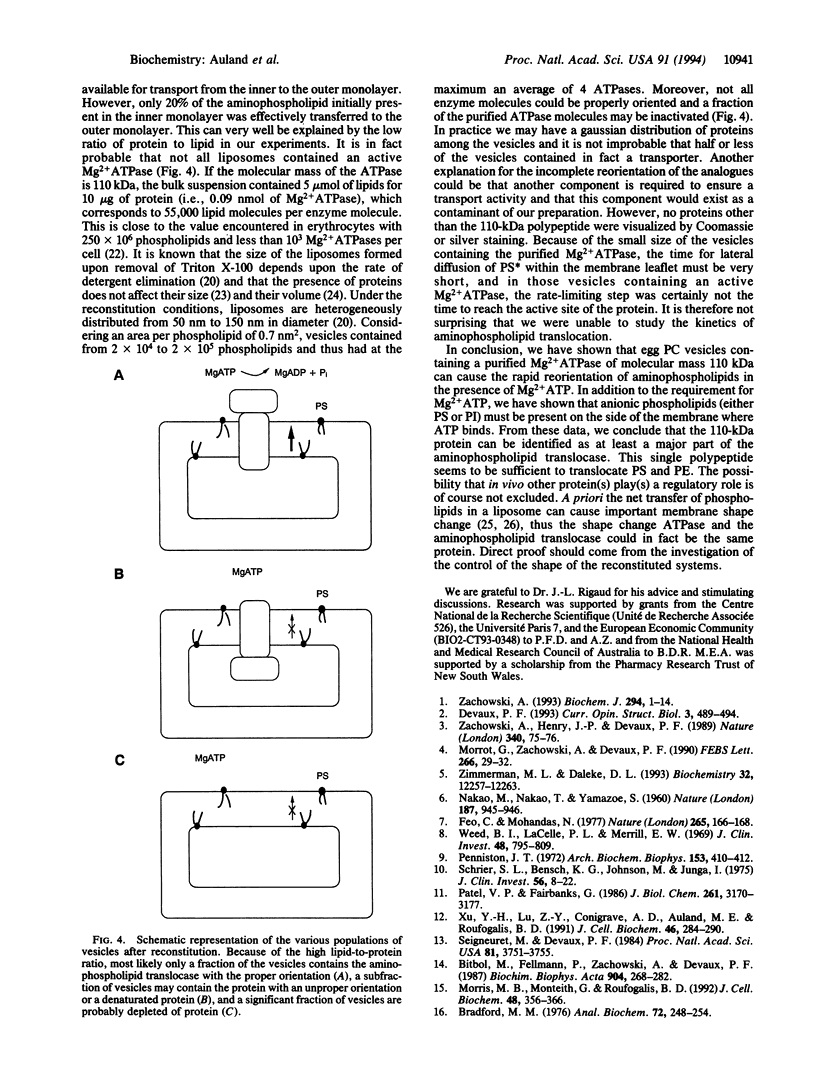
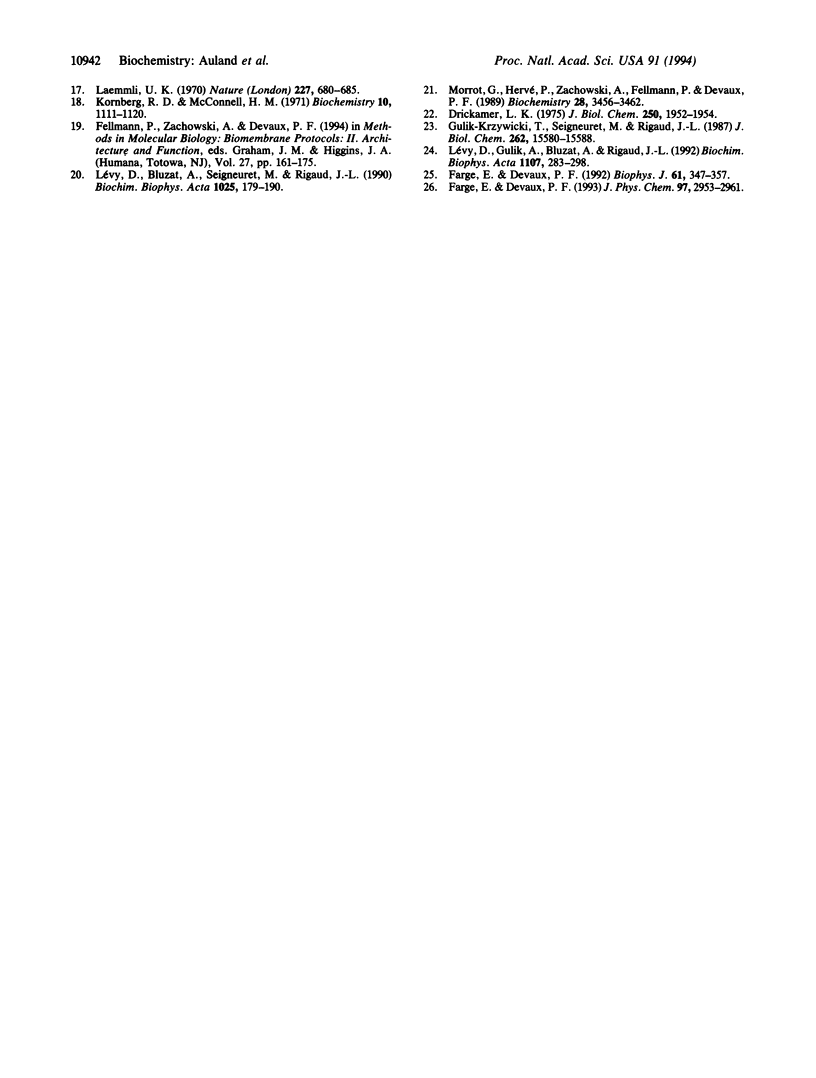
Images in this article
Selected References
These references are in PubMed. This may not be the complete list of references from this article.
- Bitbol M., Fellmann P., Zachowski A., Devaux P. F. Ion regulation of phosphatidylserine and phosphatidylethanolamine outside-inside translocation in human erythrocytes. Biochim Biophys Acta. 1987 Nov 13;904(2):268–282. doi: 10.1016/0005-2736(87)90376-2. [DOI] [PubMed] [Google Scholar]
- Bradford M. M. A rapid and sensitive method for the quantitation of microgram quantities of protein utilizing the principle of protein-dye binding. Anal Biochem. 1976 May 7;72:248–254. doi: 10.1006/abio.1976.9999. [DOI] [PubMed] [Google Scholar]
- Drickamer L. K. The red cell membrane contains three different adenosine triphophatases. J Biol Chem. 1975 Mar 10;250(5):1952–1954. [PubMed] [Google Scholar]
- Farge E., Devaux P. F. Shape changes of giant liposomes induced by an asymmetric transmembrane distribution of phospholipids. Biophys J. 1992 Feb;61(2):347–357. doi: 10.1016/S0006-3495(92)81841-6. [DOI] [PMC free article] [PubMed] [Google Scholar]
- Feo C., Mohandas N. Clarification of role of ATP in red-cell morphology and function. Nature. 1977 Jan 13;265(5590):166–168. doi: 10.1038/265166a0. [DOI] [PubMed] [Google Scholar]
- Gulik-Krzywicki T., Seigneuret M., Rigaud J. L. Monomer-oligomer equilibrium of bacteriorhodopsin in reconstituted proteoliposomes. A freeze-fracture electron microscope study. J Biol Chem. 1987 Nov 15;262(32):15580–15588. [PubMed] [Google Scholar]
- Kornberg R. D., McConnell H. M. Inside-outside transitions of phospholipids in vesicle membranes. Biochemistry. 1971 Mar 30;10(7):1111–1120. doi: 10.1021/bi00783a003. [DOI] [PubMed] [Google Scholar]
- Laemmli U. K. Cleavage of structural proteins during the assembly of the head of bacteriophage T4. Nature. 1970 Aug 15;227(5259):680–685. doi: 10.1038/227680a0. [DOI] [PubMed] [Google Scholar]
- Lévy D., Bluzat A., Seigneuret M., Rigaud J. L. A systematic study of liposome and proteoliposome reconstitution involving Bio-Bead-mediated Triton X-100 removal. Biochim Biophys Acta. 1990 Jun 27;1025(2):179–190. doi: 10.1016/0005-2736(90)90096-7. [DOI] [PubMed] [Google Scholar]
- Lévy D., Gulik A., Bluzat A., Rigaud J. L. Reconstitution of the sarcoplasmic reticulum Ca(2+)-ATPase: mechanisms of membrane protein insertion into liposomes during reconstitution procedures involving the use of detergents. Biochim Biophys Acta. 1992 Jun 30;1107(2):283–298. doi: 10.1016/0005-2736(92)90415-i. [DOI] [PubMed] [Google Scholar]
- Morris M. B., Monteith G., Roufogalis B. D. The inhibition of ATP-dependent shape change of human erythrocyte ghosts correlates with an inhibition of Mg(2+)-ATPase activity by fluoride and aluminofluoride complexes. J Cell Biochem. 1992 Apr;48(4):356–366. doi: 10.1002/jcb.240480404. [DOI] [PubMed] [Google Scholar]
- Morrot G., Hervé P., Zachowski A., Fellmann P., Devaux P. F. Aminophospholipid translocase of human erythrocytes: phospholipid substrate specificity and effect of cholesterol. Biochemistry. 1989 Apr 18;28(8):3456–3462. doi: 10.1021/bi00434a046. [DOI] [PubMed] [Google Scholar]
- Morrot G., Zachowski A., Devaux P. F. Partial purification and characterization of the human erythrocyte Mg2(+)-ATPase. A candidate aminophospholipid translocase. FEBS Lett. 1990 Jun 18;266(1-2):29–32. doi: 10.1016/0014-5793(90)81498-d. [DOI] [PubMed] [Google Scholar]
- NAKAO M., NAKAO T., YAMAZOE S. Adenosine triphosphate and maintenance of shape of the human red cells. Nature. 1960 Sep 10;187:945–946. doi: 10.1038/187945a0. [DOI] [PubMed] [Google Scholar]
- Patel V. P., Fairbanks G. Relationship of major phosphorylation reactions and MgATPase activities to ATP-dependent shape change of human erythrocyte membranes. J Biol Chem. 1986 Mar 5;261(7):3170–3177. [PubMed] [Google Scholar]
- Penniston J. T. Endocytosis by erythrocyte ghosts; dependence upon ATP hydrolysis. Arch Biochem Biophys. 1972 Nov;153(1):410–412. doi: 10.1016/0003-9861(72)90463-8. [DOI] [PubMed] [Google Scholar]
- Schriei S. L., Bensch K. G., Johnson M., Junga I. Energized endocytosis in human erythrocyte ghosts. J Clin Invest. 1975 Jul;56(1):8–22. doi: 10.1172/JCI108083. [DOI] [PMC free article] [PubMed] [Google Scholar]
- Seigneuret M., Devaux P. F. ATP-dependent asymmetric distribution of spin-labeled phospholipids in the erythrocyte membrane: relation to shape changes. Proc Natl Acad Sci U S A. 1984 Jun;81(12):3751–3755. doi: 10.1073/pnas.81.12.3751. [DOI] [PMC free article] [PubMed] [Google Scholar]
- Weed R. I., LaCelle P. L., Merrill E. W. Metabolic dependence of red cell deformability. J Clin Invest. 1969 May;48(5):795–809. doi: 10.1172/JCI106038. [DOI] [PMC free article] [PubMed] [Google Scholar]
- Xu Y. H., Lu Z. Y., Conigrave A. D., Auland M. E., Roufogalis B. D. Association of vanadate-sensitive Mg(2+)-ATPase and shape change in intact red blood cells. J Cell Biochem. 1991 Aug;46(4):284–290. doi: 10.1002/jcb.240460403. [DOI] [PubMed] [Google Scholar]
- Zachowski A., Henry J. P., Devaux P. F. Control of transmembrane lipid asymmetry in chromaffin granules by an ATP-dependent protein. Nature. 1989 Jul 6;340(6228):75–76. doi: 10.1038/340075a0. [DOI] [PubMed] [Google Scholar]
- Zachowski A. Phospholipids in animal eukaryotic membranes: transverse asymmetry and movement. Biochem J. 1993 Aug 15;294(Pt 1):1–14. doi: 10.1042/bj2940001. [DOI] [PMC free article] [PubMed] [Google Scholar]
- Zimmerman M. L., Daleke D. L. Regulation of a candidate aminophospholipid-transporting ATPase by lipid. Biochemistry. 1993 Nov 16;32(45):12257–12263. doi: 10.1021/bi00096a040. [DOI] [PubMed] [Google Scholar]