Abstract
Myosin, a molecular motor that is responsible for muscle contraction, is composed of two heavy chains each with two light chains. The crystal structure of subfragment 1 indicates that both the regulatory light chains (RLCs) and the essential light chains (ELCs) stabilize an extended alpha-helical segment of the heavy chain. It has recently been shown in a motility assay that removal of either light chain markedly reduces actin filament sliding velocity without a significant loss in actin-activated ATPase activity. Here we demonstrate by single actin filament force measurements that RLC removal has little effect on isometric force, whereas ELC removal reduces isometric force by over 50%. These data are interpreted with a simple mechanical model where subfragment 1 behaves as a torque motor whose leyer arm length is sensitive to light-chain removal. Although the effect of removing RLCs fits within the confines of this model, altered crossbridge kinetics, as reflected in a reduced unloaded duty cycle, probably contributes to the reduced velocity and force production of ELC-deficient myosins.
Full text
PDF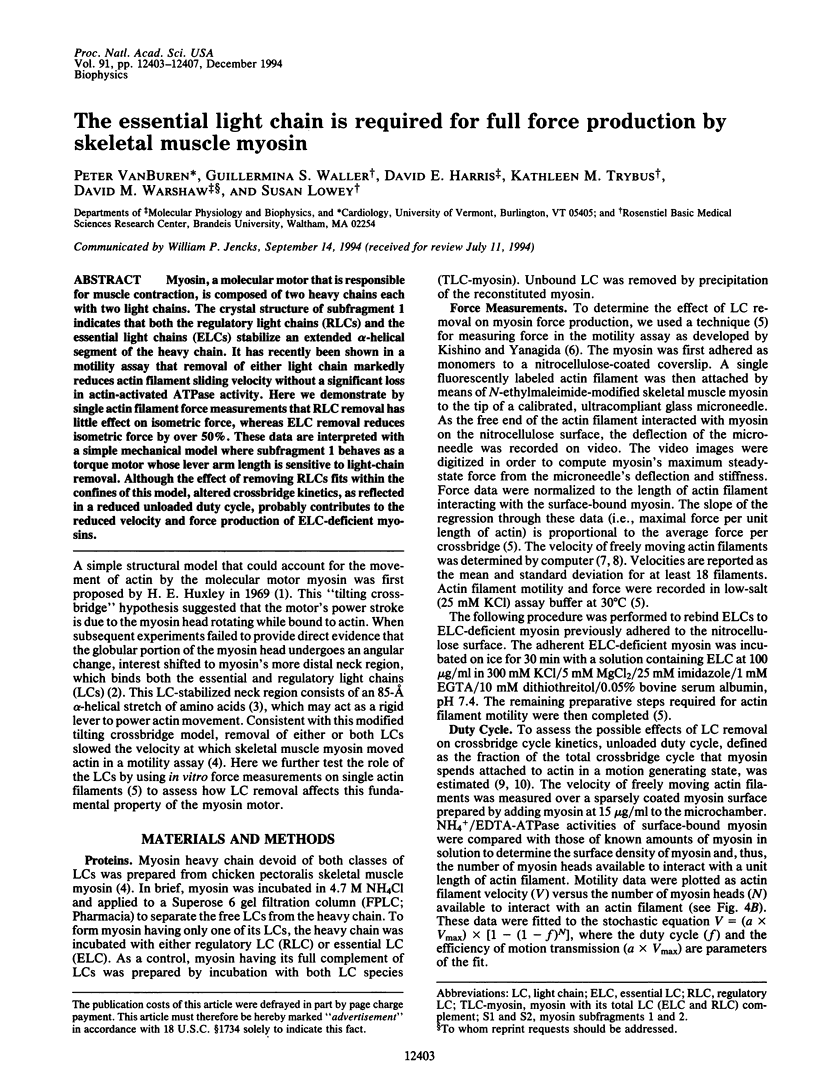
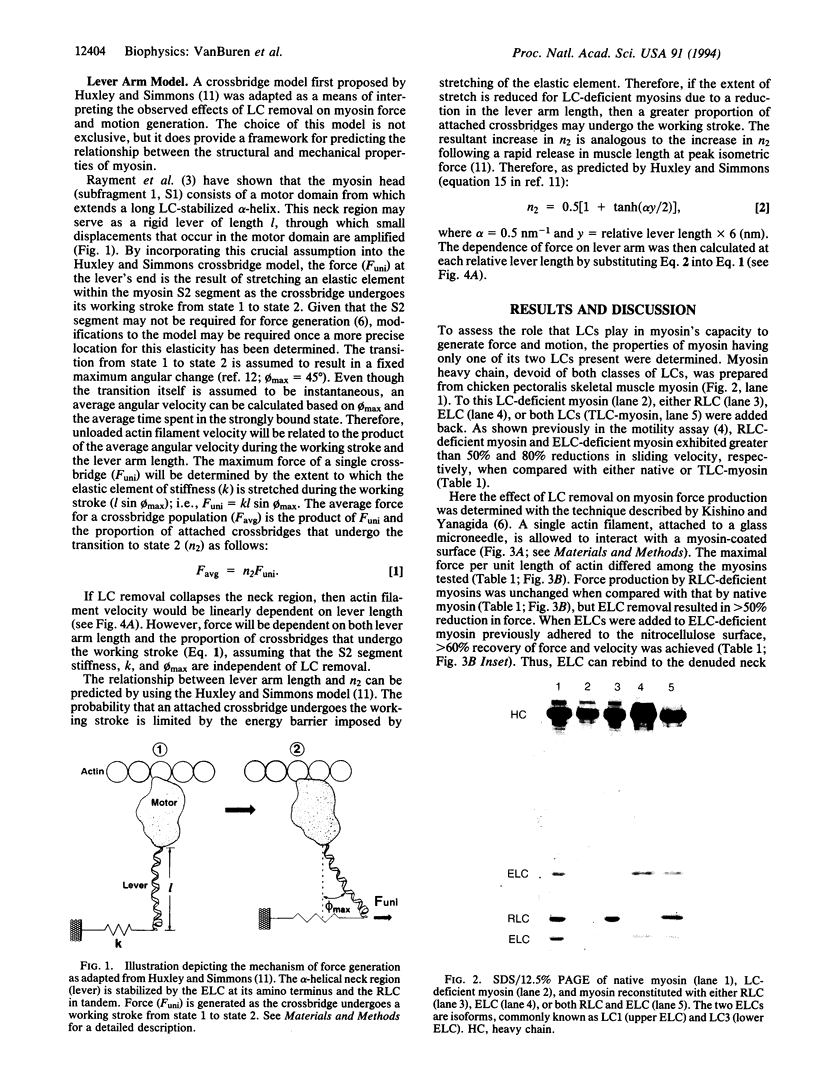
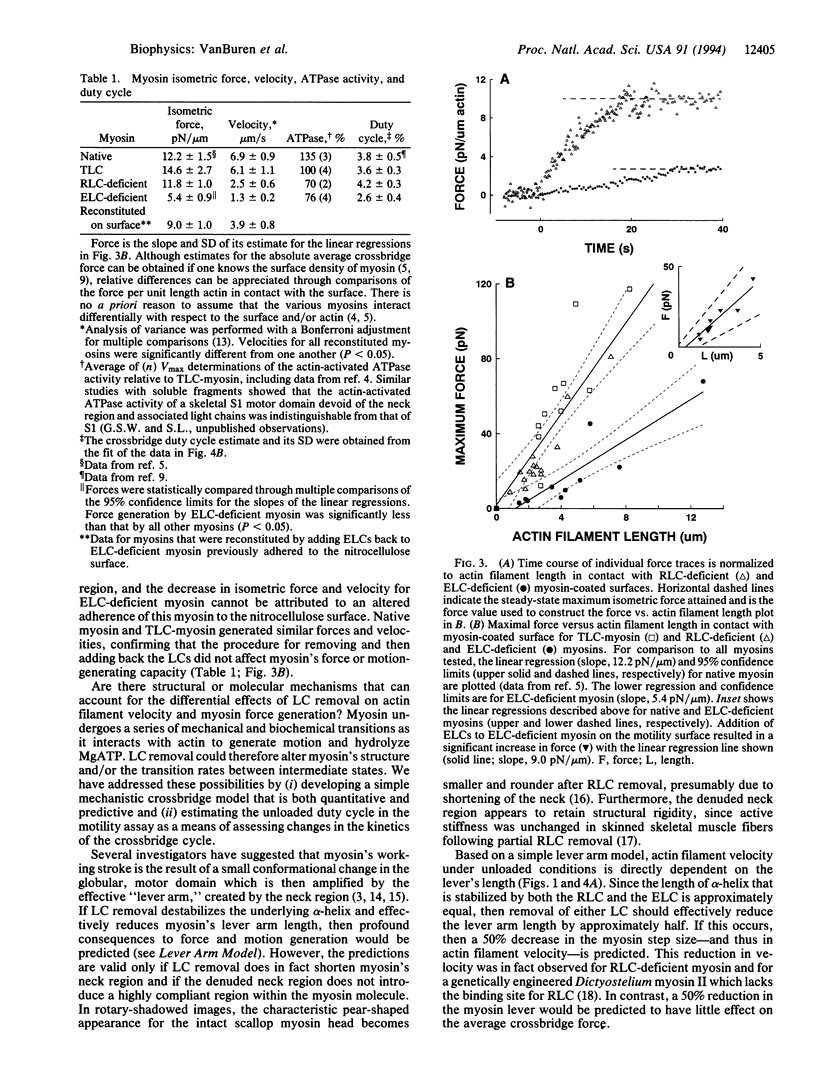
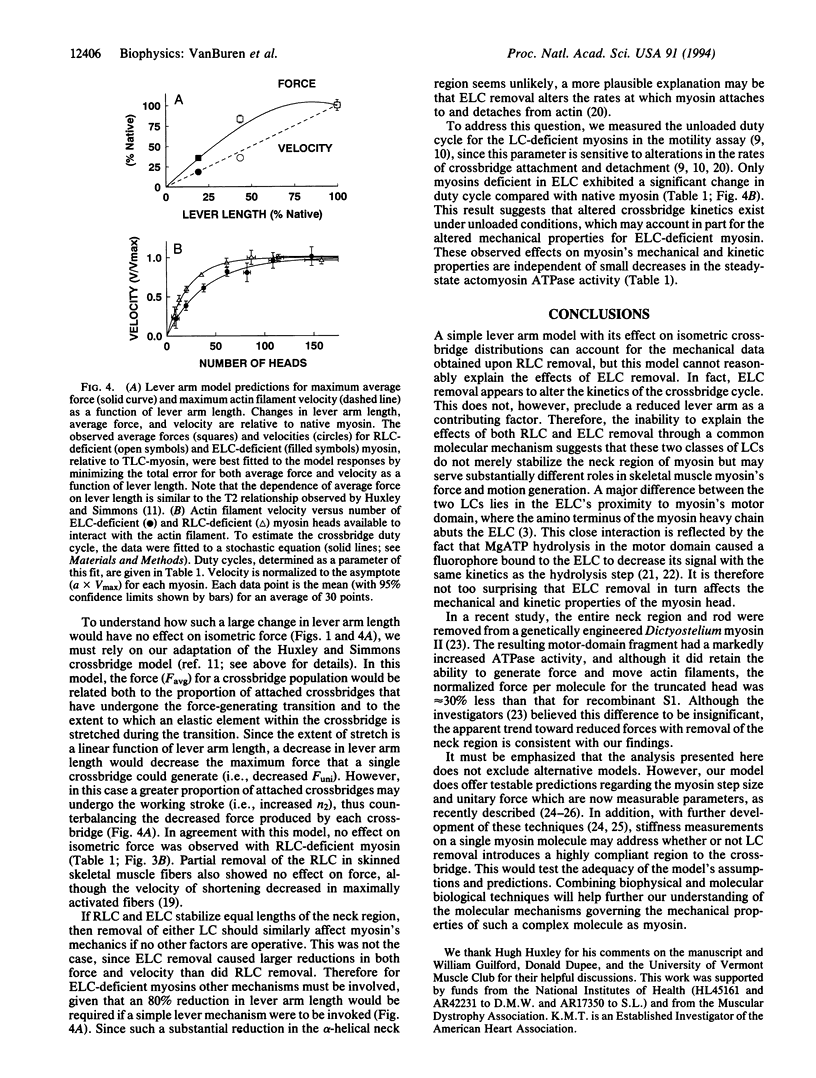
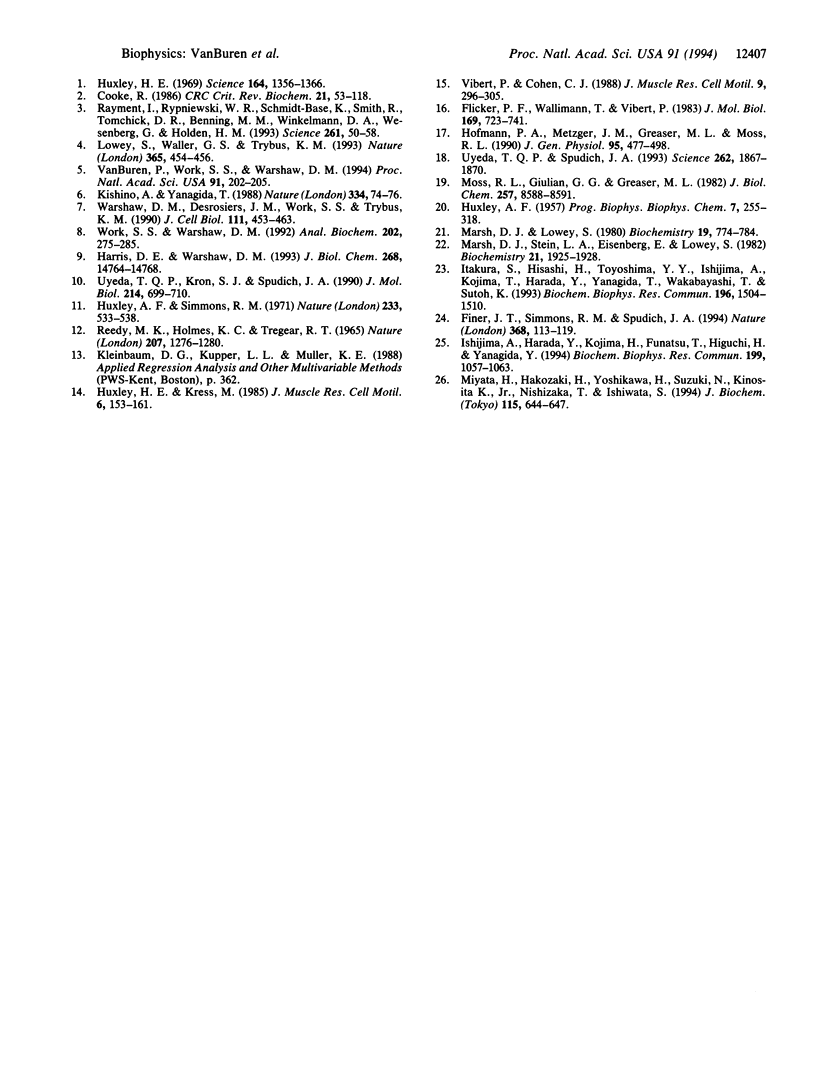
Images in this article
Selected References
These references are in PubMed. This may not be the complete list of references from this article.
- Cooke R. The mechanism of muscle contraction. CRC Crit Rev Biochem. 1986;21(1):53–118. doi: 10.3109/10409238609113609. [DOI] [PubMed] [Google Scholar]
- Finer J. T., Simmons R. M., Spudich J. A. Single myosin molecule mechanics: piconewton forces and nanometre steps. Nature. 1994 Mar 10;368(6467):113–119. doi: 10.1038/368113a0. [DOI] [PubMed] [Google Scholar]
- Flicker P. F., Wallimann T., Vibert P. Electron microscopy of scallop myosin. Location of regulatory light chains. J Mol Biol. 1983 Sep 25;169(3):723–741. doi: 10.1016/s0022-2836(83)80167-3. [DOI] [PubMed] [Google Scholar]
- HUXLEY A. F. Muscle structure and theories of contraction. Prog Biophys Biophys Chem. 1957;7:255–318. [PubMed] [Google Scholar]
- Harris D. E., Warshaw D. M. Smooth and skeletal muscle myosin both exhibit low duty cycles at zero load in vitro. J Biol Chem. 1993 Jul 15;268(20):14764–14768. [PubMed] [Google Scholar]
- Hofmann P. A., Metzger J. M., Greaser M. L., Moss R. L. Effects of partial extraction of light chain 2 on the Ca2+ sensitivities of isometric tension, stiffness, and velocity of shortening in skinned skeletal muscle fibers. J Gen Physiol. 1990 Mar;95(3):477–498. doi: 10.1085/jgp.95.3.477. [DOI] [PMC free article] [PubMed] [Google Scholar]
- Huxley A. F., Simmons R. M. Proposed mechanism of force generation in striated muscle. Nature. 1971 Oct 22;233(5321):533–538. doi: 10.1038/233533a0. [DOI] [PubMed] [Google Scholar]
- Huxley H. E., Kress M. Crossbridge behaviour during muscle contraction. J Muscle Res Cell Motil. 1985 Apr;6(2):153–161. doi: 10.1007/BF00713057. [DOI] [PubMed] [Google Scholar]
- Huxley H. E. The mechanism of muscular contraction. Science. 1969 Jun 20;164(3886):1356–1365. doi: 10.1126/science.164.3886.1356. [DOI] [PubMed] [Google Scholar]
- Ishijima A., Harada Y., Kojima H., Funatsu T., Higuchi H., Yanagida T. Single-molecule analysis of the actomyosin motor using nano-manipulation. Biochem Biophys Res Commun. 1994 Mar 15;199(2):1057–1063. doi: 10.1006/bbrc.1994.1336. [DOI] [PubMed] [Google Scholar]
- Itakura S., Yamakawa H., Toyoshima Y. Y., Ishijima A., Kojima T., Harada Y., Yanagida T., Wakabayashi T., Sutoh K. Force-generating domain of myosin motor. Biochem Biophys Res Commun. 1993 Nov 15;196(3):1504–1510. doi: 10.1006/bbrc.1993.2422. [DOI] [PubMed] [Google Scholar]
- Kishino A., Yanagida T. Force measurements by micromanipulation of a single actin filament by glass needles. Nature. 1988 Jul 7;334(6177):74–76. doi: 10.1038/334074a0. [DOI] [PubMed] [Google Scholar]
- Lowey S., Waller G. S., Trybus K. M. Skeletal muscle myosin light chains are essential for physiological speeds of shortening. Nature. 1993 Sep 30;365(6445):454–456. doi: 10.1038/365454a0. [DOI] [PubMed] [Google Scholar]
- Marsh D. J., Lowey S. Fluorescence energey transfer in myosin subfragment-1. Biochemistry. 1980 Feb 19;19(4):774–784. doi: 10.1021/bi00545a025. [DOI] [PubMed] [Google Scholar]
- Marsh D. J., Stein L. A., Eisenberg E., Lowey S. Fluorescently labeled myosin subfragment 1: identification of the kinetic step associated with the adenosine 5'-triphosphate induced fluorescence decrease. Biochemistry. 1982 Apr 13;21(8):1925–1928. doi: 10.1021/bi00537a035. [DOI] [PubMed] [Google Scholar]
- Miyata H., Hakozaki H., Yoshikawa H., Suzuki N., Kinosita K., Jr, Nishizaka T., Ishiwata S. Stepwise motion of an actin filament over a small number of heavy meromyosin molecules is revealed in an in vitro motility assay. J Biochem. 1994 Apr;115(4):644–647. doi: 10.1093/oxfordjournals.jbchem.a124389. [DOI] [PubMed] [Google Scholar]
- Moss R. L., Giulian G. G., Greaser M. L. Physiological effects accompanying the removal of myosin LC2 from skinned skeletal muscle fibers. J Biol Chem. 1982 Aug 10;257(15):8588–8591. [PubMed] [Google Scholar]
- Rayment I., Rypniewski W. R., Schmidt-Bäse K., Smith R., Tomchick D. R., Benning M. M., Winkelmann D. A., Wesenberg G., Holden H. M. Three-dimensional structure of myosin subfragment-1: a molecular motor. Science. 1993 Jul 2;261(5117):50–58. doi: 10.1126/science.8316857. [DOI] [PubMed] [Google Scholar]
- Reedy M. K., Holmes K. C., Tregear R. T. Induced changes in orientation of the cross-bridges of glycerinated insect flight muscle. Nature. 1965 Sep 18;207(5003):1276–1280. doi: 10.1038/2071276a0. [DOI] [PubMed] [Google Scholar]
- Uyeda T. Q., Kron S. J., Spudich J. A. Myosin step size. Estimation from slow sliding movement of actin over low densities of heavy meromyosin. J Mol Biol. 1990 Aug 5;214(3):699–710. doi: 10.1016/0022-2836(90)90287-V. [DOI] [PubMed] [Google Scholar]
- Uyeda T. Q., Spudich J. A. A functional recombinant myosin II lacking a regulatory light chain-binding site. Science. 1993 Dec 17;262(5141):1867–1870. doi: 10.1126/science.8266074. [DOI] [PubMed] [Google Scholar]
- VanBuren P., Work S. S., Warshaw D. M. Enhanced force generation by smooth muscle myosin in vitro. Proc Natl Acad Sci U S A. 1994 Jan 4;91(1):202–205. doi: 10.1073/pnas.91.1.202. [DOI] [PMC free article] [PubMed] [Google Scholar]
- Vibert P., Cohen C. Domains, motions and regulation in the myosin head. J Muscle Res Cell Motil. 1988 Aug;9(4):296–305. doi: 10.1007/BF01773873. [DOI] [PubMed] [Google Scholar]
- Warshaw D. M., Desrosiers J. M., Work S. S., Trybus K. M. Smooth muscle myosin cross-bridge interactions modulate actin filament sliding velocity in vitro. J Cell Biol. 1990 Aug;111(2):453–463. doi: 10.1083/jcb.111.2.453. [DOI] [PMC free article] [PubMed] [Google Scholar]
- Work S. S., Warshaw D. M. Computer-assisted tracking of actin filament motility. Anal Biochem. 1992 May 1;202(2):275–285. doi: 10.1016/0003-2697(92)90106-h. [DOI] [PubMed] [Google Scholar]