Abstract
During anaerobic growth of Escherichia coli an oxygen-sensitive ribonucleoside-triphosphate reductase, different from the aerobic ribonucleoside diphosphate-reductase (EC 1.17.4.1), produces the deoxyribonucleoside triphosphates required for DNA replication. The gene for the anaerobic enzyme has now been cloned and was found to contain a 2136-nucleotide coding region, corresponding to 712 amino acid residues, and an Fnr binding site 228 base pairs upstream of the initiator ATG. The deduced amino acid sequence shows 72% identity to a gene of coliphage T4, sunY, hitherto of unknown function, suggesting that the virus codes for its own anaerobic reductase. The location of an organic free radical formed during activation of the bacterial anaerobic reductase is proposed to be on Gly-681, since the pentapeptide RVCGY at positions 678-682 shows a striking similarity to the C-terminal sequence. RVSGY, of pyruvate formate-lyase. During activation of the anaerobically induced pyruvate formate-lyase, the glycine residue of the pentapeptide becomes an organic radical [Wagner, A. F. V., Frey, M., Neugebauer, F. A., Schäfer, W. & Knappe, J. (1992) Proc. Natl. Acad. Sci. USA 89, 996-1000]. The gene for the anaerobic reductase is located at a position around 96 min on the E. coli genomic map.
Full text
PDF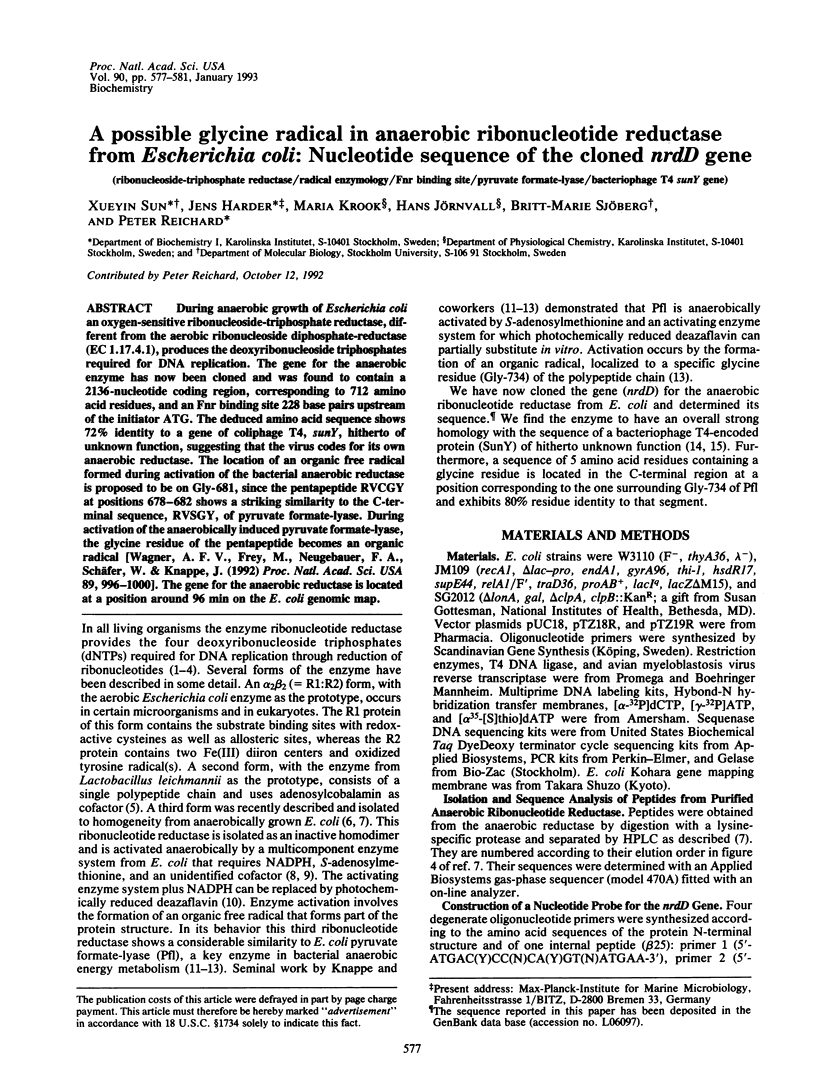
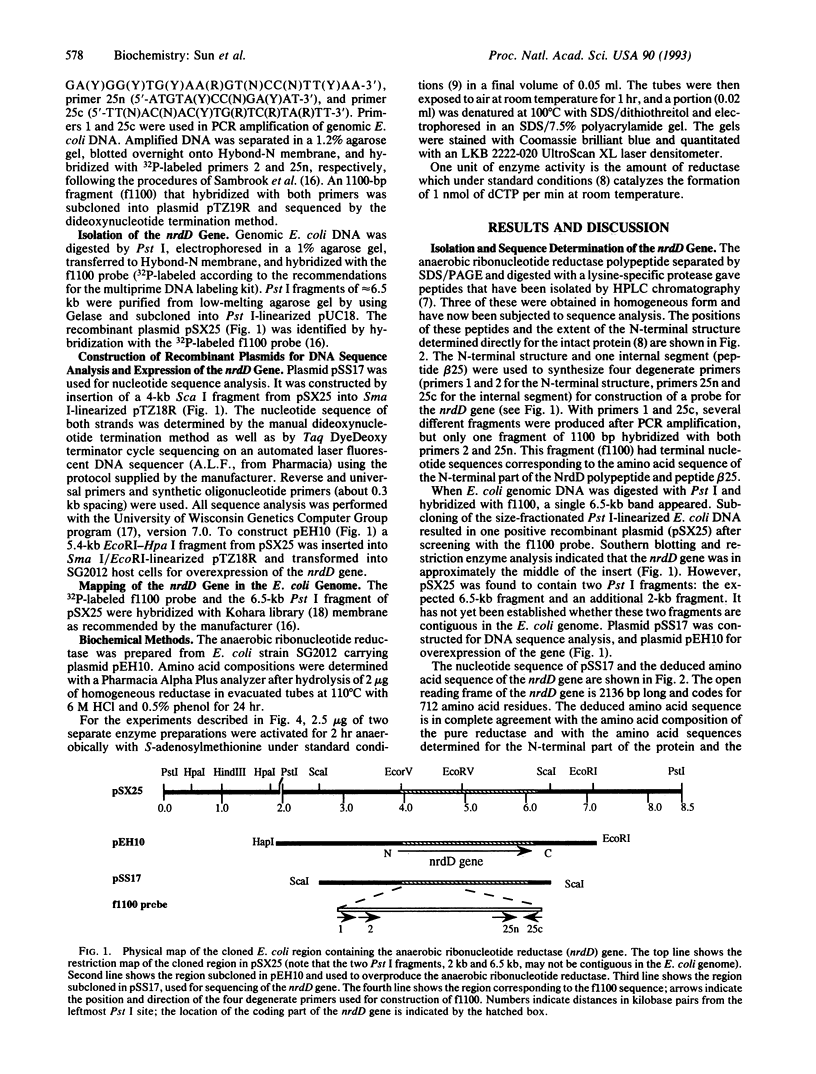
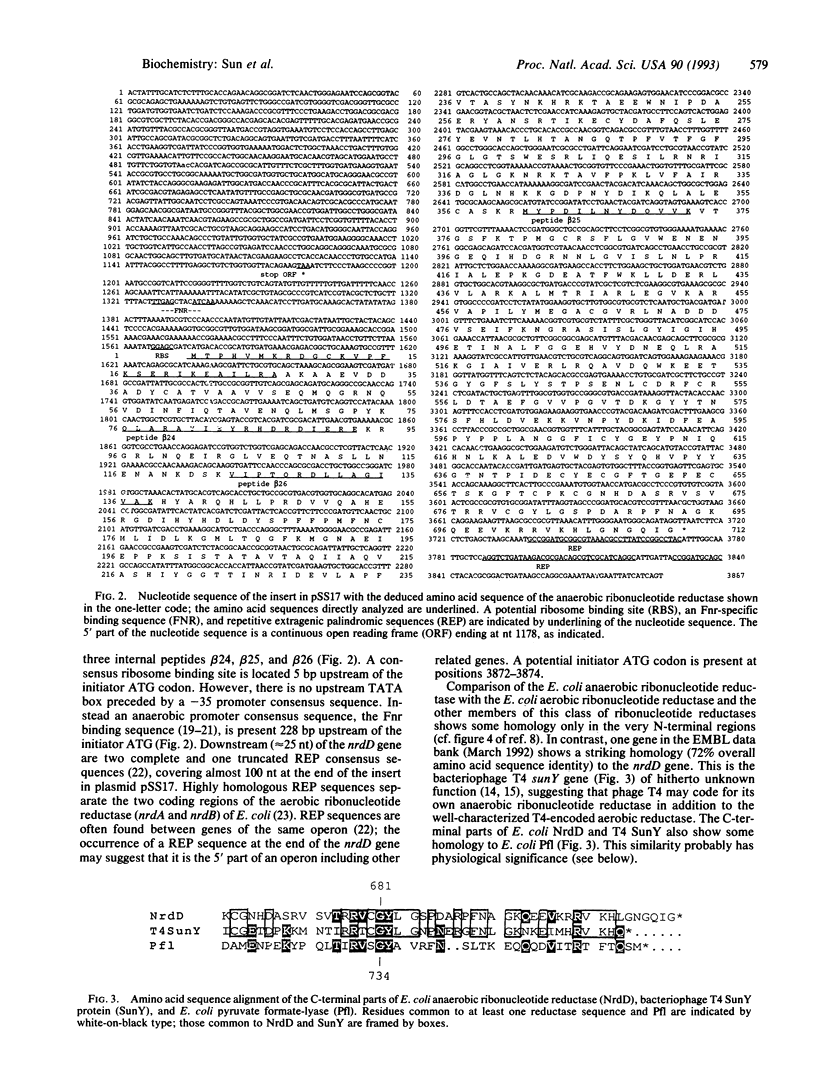
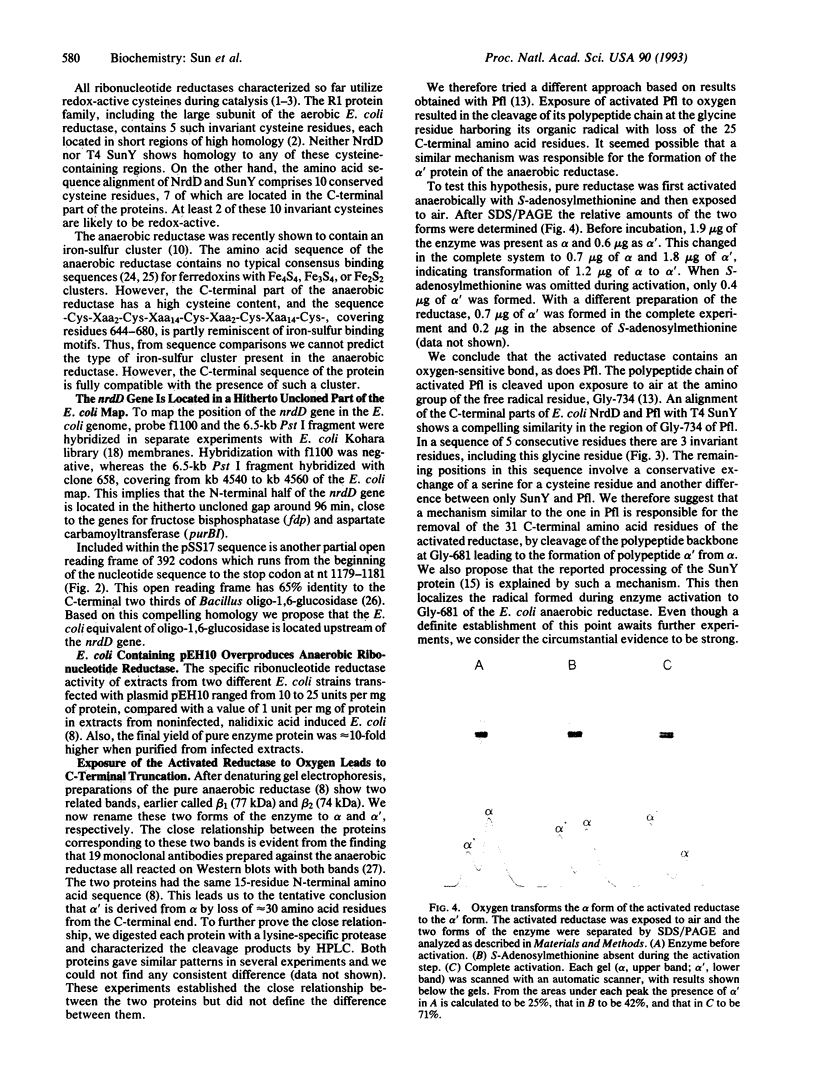
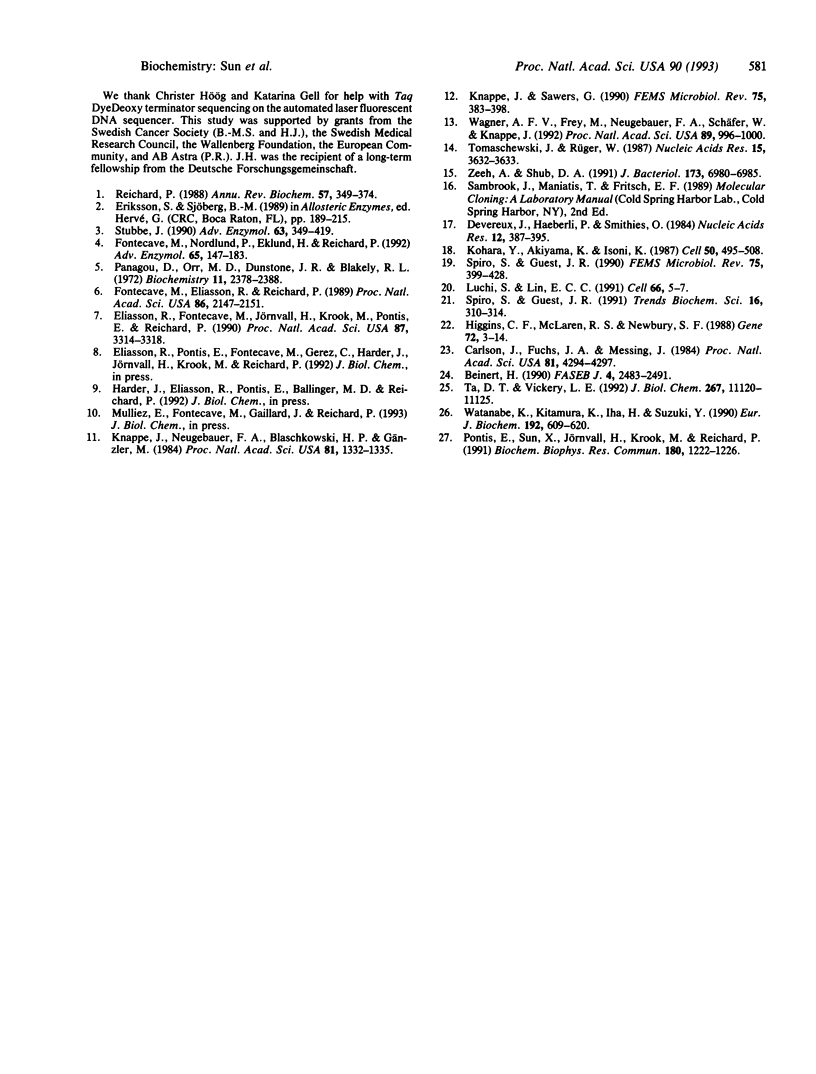
Images in this article
Selected References
These references are in PubMed. This may not be the complete list of references from this article.
- Beinert H. Recent developments in the field of iron-sulfur proteins. FASEB J. 1990 May;4(8):2483–2491. doi: 10.1096/fasebj.4.8.2185975. [DOI] [PubMed] [Google Scholar]
- Carlson J., Fuchs J. A., Messing J. Primary structure of the Escherichia coli ribonucleoside diphosphate reductase operon. Proc Natl Acad Sci U S A. 1984 Jul;81(14):4294–4297. doi: 10.1073/pnas.81.14.4294. [DOI] [PMC free article] [PubMed] [Google Scholar]
- Devereux J., Haeberli P., Smithies O. A comprehensive set of sequence analysis programs for the VAX. Nucleic Acids Res. 1984 Jan 11;12(1 Pt 1):387–395. doi: 10.1093/nar/12.1part1.387. [DOI] [PMC free article] [PubMed] [Google Scholar]
- Eliasson R., Fontecave M., Jörnvall H., Krook M., Pontis E., Reichard P. The anaerobic ribonucleoside triphosphate reductase from Escherichia coli requires S-adenosylmethionine as a cofactor. Proc Natl Acad Sci U S A. 1990 May;87(9):3314–3318. doi: 10.1073/pnas.87.9.3314. [DOI] [PMC free article] [PubMed] [Google Scholar]
- Fontecave M., Eliasson R., Reichard P. Oxygen-sensitive ribonucleoside triphosphate reductase is present in anaerobic Escherichia coli. Proc Natl Acad Sci U S A. 1989 Apr;86(7):2147–2151. doi: 10.1073/pnas.86.7.2147. [DOI] [PMC free article] [PubMed] [Google Scholar]
- Fontecave M., Nordlund P., Eklund H., Reichard P. The redox centers of ribonucleotide reductase of Escherichia coli. Adv Enzymol Relat Areas Mol Biol. 1992;65:147–183. doi: 10.1002/9780470123119.ch4. [DOI] [PubMed] [Google Scholar]
- Higgins C. F., McLaren R. S., Newbury S. F. Repetitive extragenic palindromic sequences, mRNA stability and gene expression: evolution by gene conversion? A review. Gene. 1988 Dec 10;72(1-2):3–14. doi: 10.1016/0378-1119(88)90122-9. [DOI] [PubMed] [Google Scholar]
- Iuchi S., Lin E. C. Adaptation of Escherichia coli to respiratory conditions: regulation of gene expression. Cell. 1991 Jul 12;66(1):5–7. doi: 10.1016/0092-8674(91)90130-q. [DOI] [PubMed] [Google Scholar]
- Knappe J., Neugebauer F. A., Blaschkowski H. P., Gänzler M. Post-translational activation introduces a free radical into pyruvate formate-lyase. Proc Natl Acad Sci U S A. 1984 Mar;81(5):1332–1335. doi: 10.1073/pnas.81.5.1332. [DOI] [PMC free article] [PubMed] [Google Scholar]
- Knappe J., Sawers G. A radical-chemical route to acetyl-CoA: the anaerobically induced pyruvate formate-lyase system of Escherichia coli. FEMS Microbiol Rev. 1990 Aug;6(4):383–398. doi: 10.1111/j.1574-6968.1990.tb04108.x. [DOI] [PubMed] [Google Scholar]
- Kohara Y., Akiyama K., Isono K. The physical map of the whole E. coli chromosome: application of a new strategy for rapid analysis and sorting of a large genomic library. Cell. 1987 Jul 31;50(3):495–508. doi: 10.1016/0092-8674(87)90503-4. [DOI] [PubMed] [Google Scholar]
- Panagou D., Orr M. D., Dunstone J. R., Blakley R. L. A monomeric, allosteric enzyme with a single polypeptide chain. Ribonucleotide reductase of Lactobacillus leichmannii. Biochemistry. 1972 Jun 6;11(12):2378–2388. doi: 10.1021/bi00762a025. [DOI] [PubMed] [Google Scholar]
- Pontis E., Sun X. Y., Jörnvall H., Krook M., Reichard P. ClpB proteins copurify with the anaerobic Escherichia coli reductase. Biochem Biophys Res Commun. 1991 Nov 14;180(3):1222–1226. doi: 10.1016/s0006-291x(05)81326-9. [DOI] [PubMed] [Google Scholar]
- Reichard P. Interactions between deoxyribonucleotide and DNA synthesis. Annu Rev Biochem. 1988;57:349–374. doi: 10.1146/annurev.bi.57.070188.002025. [DOI] [PubMed] [Google Scholar]
- Spiro S., Guest J. R. Adaptive responses to oxygen limitation in Escherichia coli. Trends Biochem Sci. 1991 Aug;16(8):310–314. doi: 10.1016/0968-0004(91)90125-f. [DOI] [PubMed] [Google Scholar]
- Spiro S., Guest J. R. FNR and its role in oxygen-regulated gene expression in Escherichia coli. FEMS Microbiol Rev. 1990 Aug;6(4):399–428. doi: 10.1111/j.1574-6968.1990.tb04109.x. [DOI] [PubMed] [Google Scholar]
- Stubbe J. Ribonucleotide reductases. Adv Enzymol Relat Areas Mol Biol. 1990;63:349–419. doi: 10.1002/9780470123096.ch6. [DOI] [PubMed] [Google Scholar]
- Ta D. T., Vickery L. E. Cloning, sequencing, and overexpression of a [2Fe-2S] ferredoxin gene from Escherichia coli. J Biol Chem. 1992 Jun 5;267(16):11120–11125. [PubMed] [Google Scholar]
- Tomaschewski J., Rüger W. Nucleotide sequence and primary structures of gene products coded for by the T4 genome between map positions 48.266 kb and 39.166 kb. Nucleic Acids Res. 1987 Apr 24;15(8):3632–3633. doi: 10.1093/nar/15.8.3632. [DOI] [PMC free article] [PubMed] [Google Scholar]
- Wagner A. F., Frey M., Neugebauer F. A., Schäfer W., Knappe J. The free radical in pyruvate formate-lyase is located on glycine-734. Proc Natl Acad Sci U S A. 1992 Feb 1;89(3):996–1000. doi: 10.1073/pnas.89.3.996. [DOI] [PMC free article] [PubMed] [Google Scholar]
- Watanabe K., Kitamura K., Iha H., Suzuki Y. Primary structure of the oligo-1,6-glucosidase of Bacillus cereus ATCC7064 deduced from the nucleotide sequence of the cloned gene. Eur J Biochem. 1990 Sep 24;192(3):609–620. doi: 10.1111/j.1432-1033.1990.tb19267.x. [DOI] [PubMed] [Google Scholar]
- Zeeh A., Shub D. A. The product of the split sunY gene of bacteriophage T4 is a processed protein. J Bacteriol. 1991 Nov;173(21):6980–6985. doi: 10.1128/jb.173.21.6980-6985.1991. [DOI] [PMC free article] [PubMed] [Google Scholar]