Abstract
The membrane-bound complex of bacterial periplasmic permeases consists of two hydrophobic integral membrane proteins and two copies of a hydrophilic ATP-binding protein. The ATP-binding proteins from all periplasmic permeases display a high level of sequence similarity and are referred to as "conserved components." The conserved component from the histidine permease, HisP, has been postulated on the basis of genetic evidence to be accessible at the exterior membrane surface, in contrast to the commonly postulated association with the interior membrane surface as peripheral membrane proteins. We have used proteolysis and biotinylation of membrane vesicles to show that HisP is accessible to these reagents at the external surface and that this orientation depends on the presence of the two hydrophobic components, HisQ and HisM. Several binding-protein-independent hisP mutants are shown to produce HisP proteins that are more susceptible to proteases from the external membrane surface. Since the hydrophilic component is well conserved also in a group of eukaryotic transporters, which together with many prokaryotic systems form the superfamily of traffic ATPases, this insight about its membrane topology has general implications for understanding the molecular mechanism of action of this large superfamily, which includes the cystic fibrosis transmembrane conductance regulator and multidrug-resistance proteins.
Full text
PDF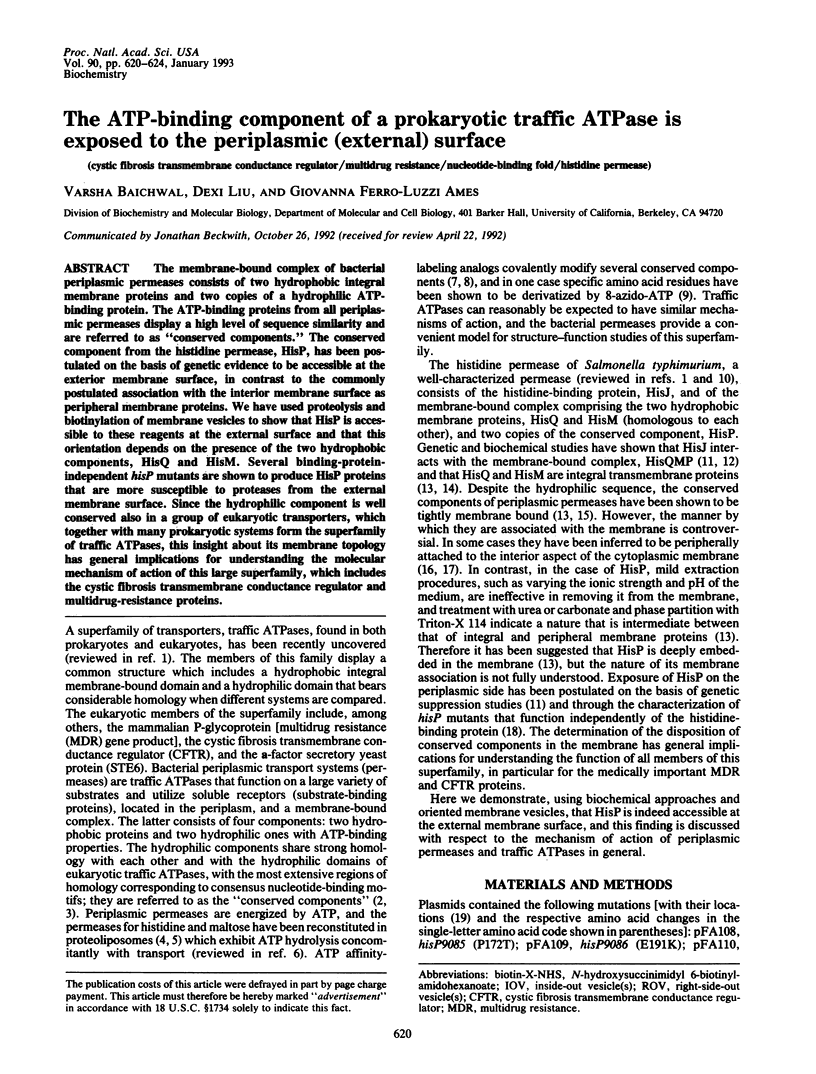
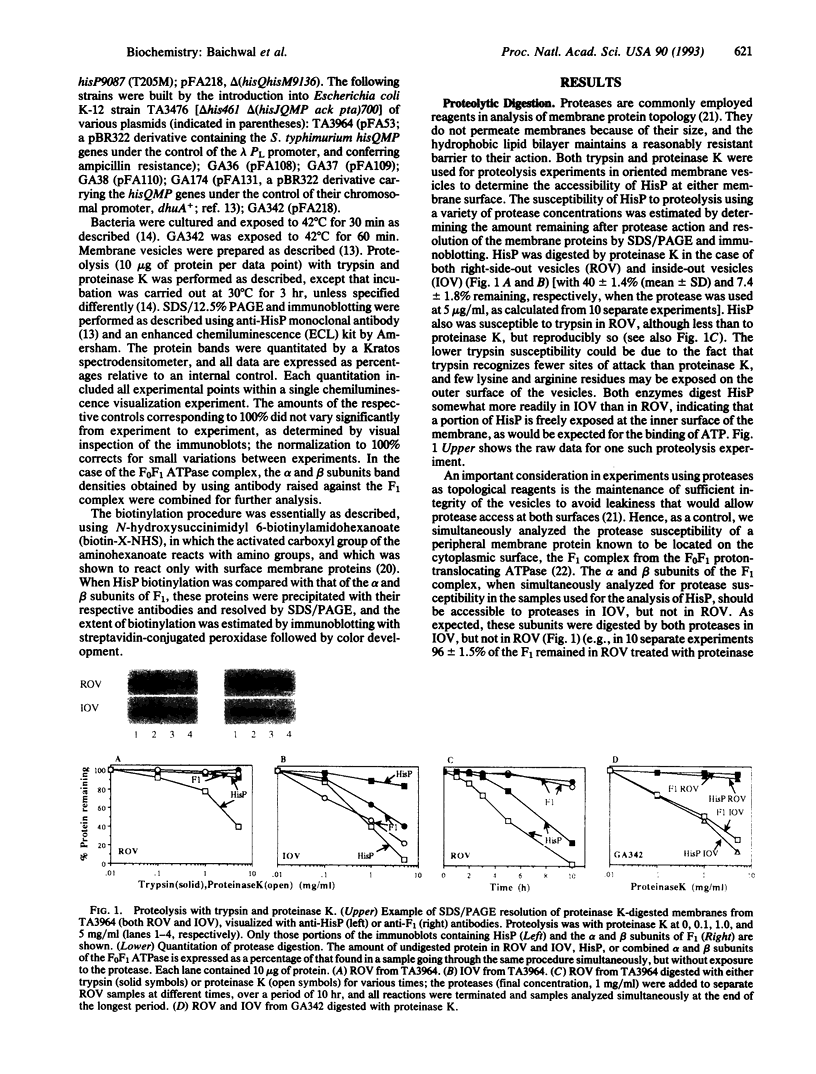
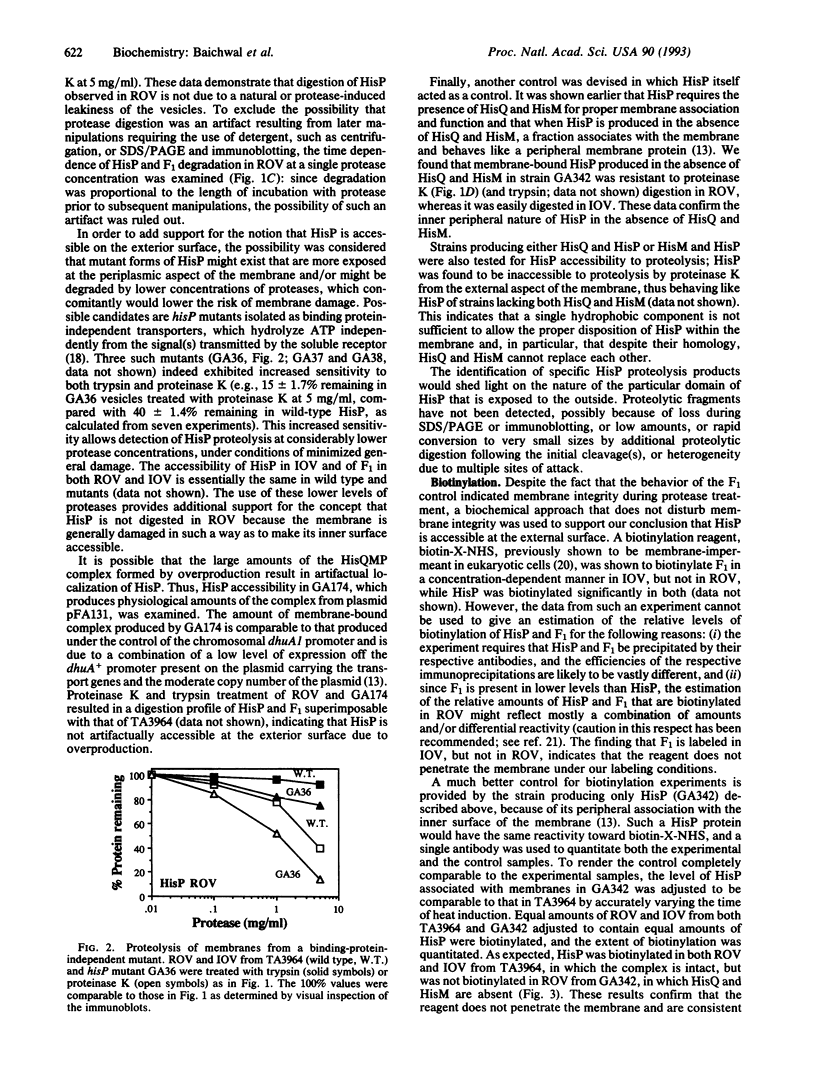
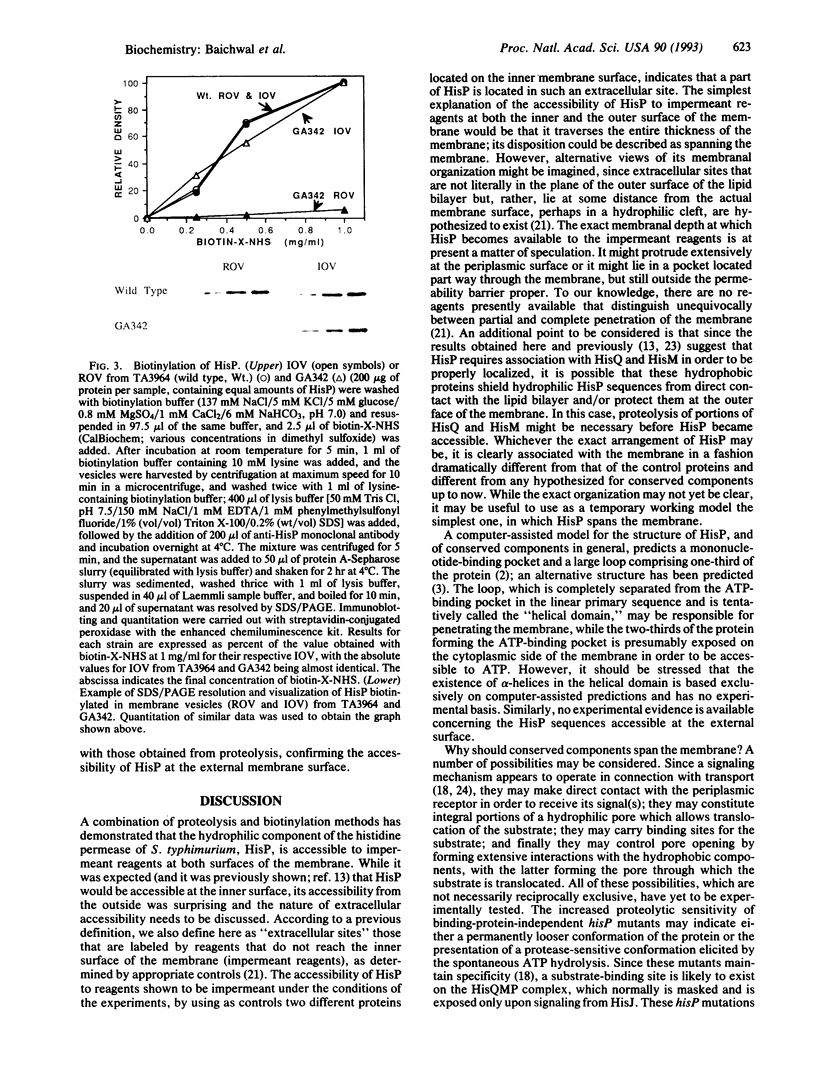
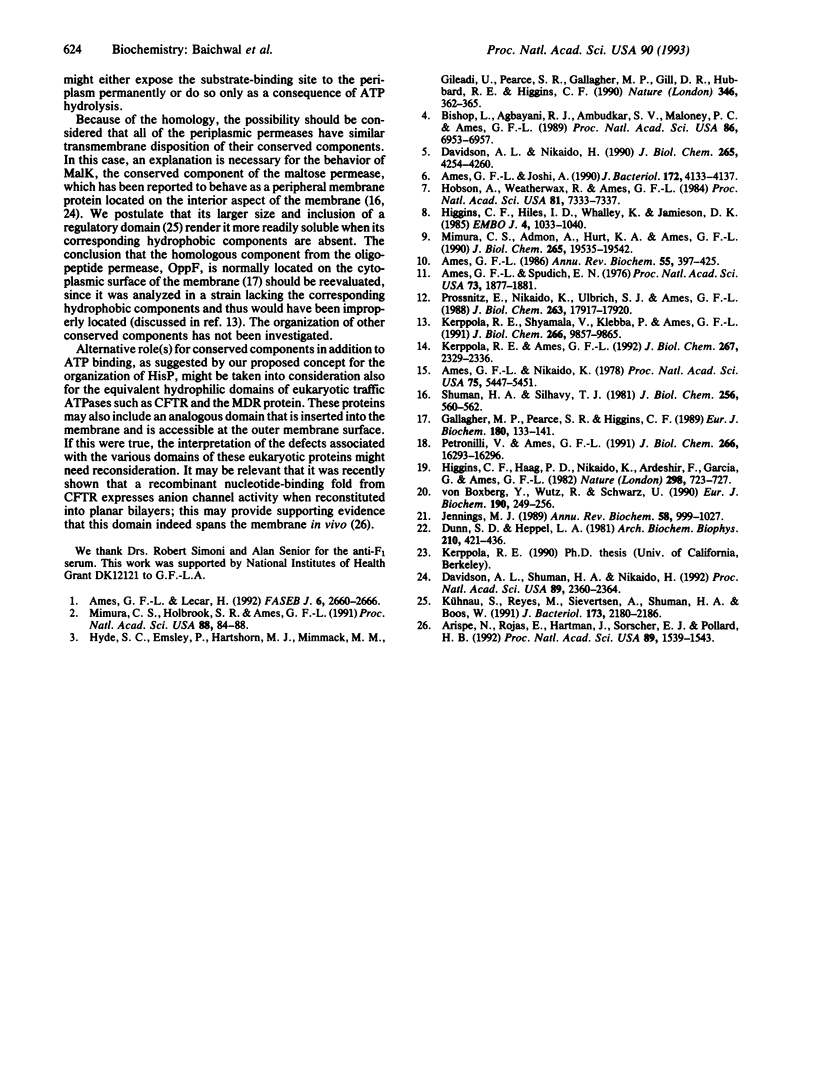
Images in this article
Selected References
These references are in PubMed. This may not be the complete list of references from this article.
- Ames G. F. Bacterial periplasmic transport systems: structure, mechanism, and evolution. Annu Rev Biochem. 1986;55:397–425. doi: 10.1146/annurev.bi.55.070186.002145. [DOI] [PubMed] [Google Scholar]
- Ames G. F., Joshi A. K. Energy coupling in bacterial periplasmic permeases. J Bacteriol. 1990 Aug;172(8):4133–4137. doi: 10.1128/jb.172.8.4133-4137.1990. [DOI] [PMC free article] [PubMed] [Google Scholar]
- Ames G. F., Lecar H. ATP-dependent bacterial transporters and cystic fibrosis: analogy between channels and transporters. FASEB J. 1992 Jun;6(9):2660–2666. doi: 10.1096/fasebj.6.9.1377140. [DOI] [PubMed] [Google Scholar]
- Ames G. F., Nikaido K. Identification of a membrane protein as a histidine transport component in Salmonella typhimurium. Proc Natl Acad Sci U S A. 1978 Nov;75(11):5447–5451. doi: 10.1073/pnas.75.11.5447. [DOI] [PMC free article] [PubMed] [Google Scholar]
- Ames G. F., Spurich E. N. Protein-protein interaction in transport: periplasmic histidine-binding protein J interacts with P protein. Proc Natl Acad Sci U S A. 1976 Jun;73(6):1877–1881. doi: 10.1073/pnas.73.6.1877. [DOI] [PMC free article] [PubMed] [Google Scholar]
- Arispe N., Rojas E., Hartman J., Sorscher E. J., Pollard H. B. Intrinsic anion channel activity of the recombinant first nucleotide binding fold domain of the cystic fibrosis transmembrane regulator protein. Proc Natl Acad Sci U S A. 1992 Mar 1;89(5):1539–1543. doi: 10.1073/pnas.89.5.1539. [DOI] [PMC free article] [PubMed] [Google Scholar]
- Bishop L., Agbayani R., Jr, Ambudkar S. V., Maloney P. C., Ames G. F. Reconstitution of a bacterial periplasmic permease in proteoliposomes and demonstration of ATP hydrolysis concomitant with transport. Proc Natl Acad Sci U S A. 1989 Sep;86(18):6953–6957. doi: 10.1073/pnas.86.18.6953. [DOI] [PMC free article] [PubMed] [Google Scholar]
- Davidson A. L., Nikaido H. Overproduction, solubilization, and reconstitution of the maltose transport system from Escherichia coli. J Biol Chem. 1990 Mar 15;265(8):4254–4260. [PubMed] [Google Scholar]
- Davidson A. L., Shuman H. A., Nikaido H. Mechanism of maltose transport in Escherichia coli: transmembrane signaling by periplasmic binding proteins. Proc Natl Acad Sci U S A. 1992 Mar 15;89(6):2360–2364. doi: 10.1073/pnas.89.6.2360. [DOI] [PMC free article] [PubMed] [Google Scholar]
- Dunn S. D., Heppel L. A. Properties and functions of the subunits of the Escherichia coli coupling factor ATPase. Arch Biochem Biophys. 1981 Sep;210(2):421–436. doi: 10.1016/0003-9861(81)90206-x. [DOI] [PubMed] [Google Scholar]
- Gallagher M. P., Pearce S. R., Higgins C. F. Identification and localization of the membrane-associated, ATP-binding subunit of the oligopeptide permease of Salmonella typhimurium. Eur J Biochem. 1989 Mar 1;180(1):133–141. doi: 10.1111/j.1432-1033.1989.tb14623.x. [DOI] [PubMed] [Google Scholar]
- Higgins C. F., Haag P. D., Nikaido K., Ardeshir F., Garcia G., Ames G. F. Complete nucleotide sequence and identification of membrane components of the histidine transport operon of S. typhimurium. Nature. 1982 Aug 19;298(5876):723–727. doi: 10.1038/298723a0. [DOI] [PubMed] [Google Scholar]
- Higgins C. F., Hiles I. D., Whalley K., Jamieson D. J. Nucleotide binding by membrane components of bacterial periplasmic binding protein-dependent transport systems. EMBO J. 1985 Apr;4(4):1033–1039. doi: 10.1002/j.1460-2075.1985.tb03735.x. [DOI] [PMC free article] [PubMed] [Google Scholar]
- Hobson A. C., Weatherwax R., Ames G. F. ATP-binding sites in the membrane components of histidine permease, a periplasmic transport system. Proc Natl Acad Sci U S A. 1984 Dec;81(23):7333–7337. doi: 10.1073/pnas.81.23.7333. [DOI] [PMC free article] [PubMed] [Google Scholar]
- Hyde S. C., Emsley P., Hartshorn M. J., Mimmack M. M., Gileadi U., Pearce S. R., Gallagher M. P., Gill D. R., Hubbard R. E., Higgins C. F. Structural model of ATP-binding proteins associated with cystic fibrosis, multidrug resistance and bacterial transport. Nature. 1990 Jul 26;346(6282):362–365. doi: 10.1038/346362a0. [DOI] [PubMed] [Google Scholar]
- Jennings M. L. Topography of membrane proteins. Annu Rev Biochem. 1989;58:999–1027. doi: 10.1146/annurev.bi.58.070189.005031. [DOI] [PubMed] [Google Scholar]
- Kerppola R. E., Ames G. F. Topology of the hydrophobic membrane-bound components of the histidine periplasmic permease. Comparison with other members of the family. J Biol Chem. 1992 Feb 5;267(4):2329–2336. [PubMed] [Google Scholar]
- Kerppola R. E., Shyamala V. K., Klebba P., Ames G. F. The membrane-bound proteins of periplasmic permeases form a complex. Identification of the histidine permease HisQMP complex. J Biol Chem. 1991 May 25;266(15):9857–9865. [PubMed] [Google Scholar]
- Kühnau S., Reyes M., Sievertsen A., Shuman H. A., Boos W. The activities of the Escherichia coli MalK protein in maltose transport, regulation, and inducer exclusion can be separated by mutations. J Bacteriol. 1991 Apr;173(7):2180–2186. doi: 10.1128/jb.173.7.2180-2186.1991. [DOI] [PMC free article] [PubMed] [Google Scholar]
- Mimura C. S., Admon A., Hurt K. A., Ames G. F. The nucleotide-binding site of HisP, a membrane protein of the histidine permease. Identification of amino acid residues photoaffinity labeled by 8-azido-ATP. J Biol Chem. 1990 Nov 15;265(32):19535–19542. [PubMed] [Google Scholar]
- Mimura C. S., Holbrook S. R., Ames G. F. Structural model of the nucleotide-binding conserved component of periplasmic permeases. Proc Natl Acad Sci U S A. 1991 Jan 1;88(1):84–88. doi: 10.1073/pnas.88.1.84. [DOI] [PMC free article] [PubMed] [Google Scholar]
- Petronilli V., Ames G. F. Binding protein-independent histidine permease mutants. Uncoupling of ATP hydrolysis from transmembrane signaling. J Biol Chem. 1991 Sep 5;266(25):16293–16296. [PubMed] [Google Scholar]
- Prossnitz E., Nikaido K., Ulbrich S. J., Ames G. F. Formaldehyde and photoactivatable cross-linking of the periplasmic binding protein to a membrane component of the histidine transport system of Salmonella typhimurium. J Biol Chem. 1988 Dec 5;263(34):17917–17920. [PubMed] [Google Scholar]
- Shuman H. A., Silhavy T. J. Identification of the malK gene product. A peripheral membrane component of the Escherichia coli maltose transport system. J Biol Chem. 1981 Jan 25;256(2):560–562. [PubMed] [Google Scholar]
- von Boxberg Y., Wütz R., Schwarz U. Use of the biotin-avidin system for labelling, isolation and characterization of neural cell-surface proteins. Eur J Biochem. 1990 Jun 20;190(2):249–256. doi: 10.1111/j.1432-1033.1990.tb15569.x. [DOI] [PubMed] [Google Scholar]