Abstract
How does a protein find its native state without a globally exhaustive search? We propose the "HZ" (hydrophobic zipper) hypothesis: hydrophobic contacts act as constraints that bring other contacts into spatial proximity, which then further constrain and zip up the next contacts, etc. In contrast to helix-coil cooperativity, HZ-heteropolymer collapse cooperativity is driven by nonlocal interactions, causes sheet and irregular conformations in addition to helices, leads to secondary structures concurrently with early hydrophobic core formation, is much more sequence dependent than helix-coil processes, and involves compact intermediate states that have much secondary--but little tertiary--structure. Hydrophobic contacts in the 1992 Protein Data Bank have the type of "topological localness" predicted by the hypothesis. The HZ paths for amino acid sequences that mimic crambin and bovine pancreatic trypsin inhibitor are quickly found by computer; the best configurations thus reached have single hydrophobic cores that are within about 3 kcal/mol of the global minimum. This hypothesis shows how proteins could find globally optimal states without exhaustive search.
Full text
PDF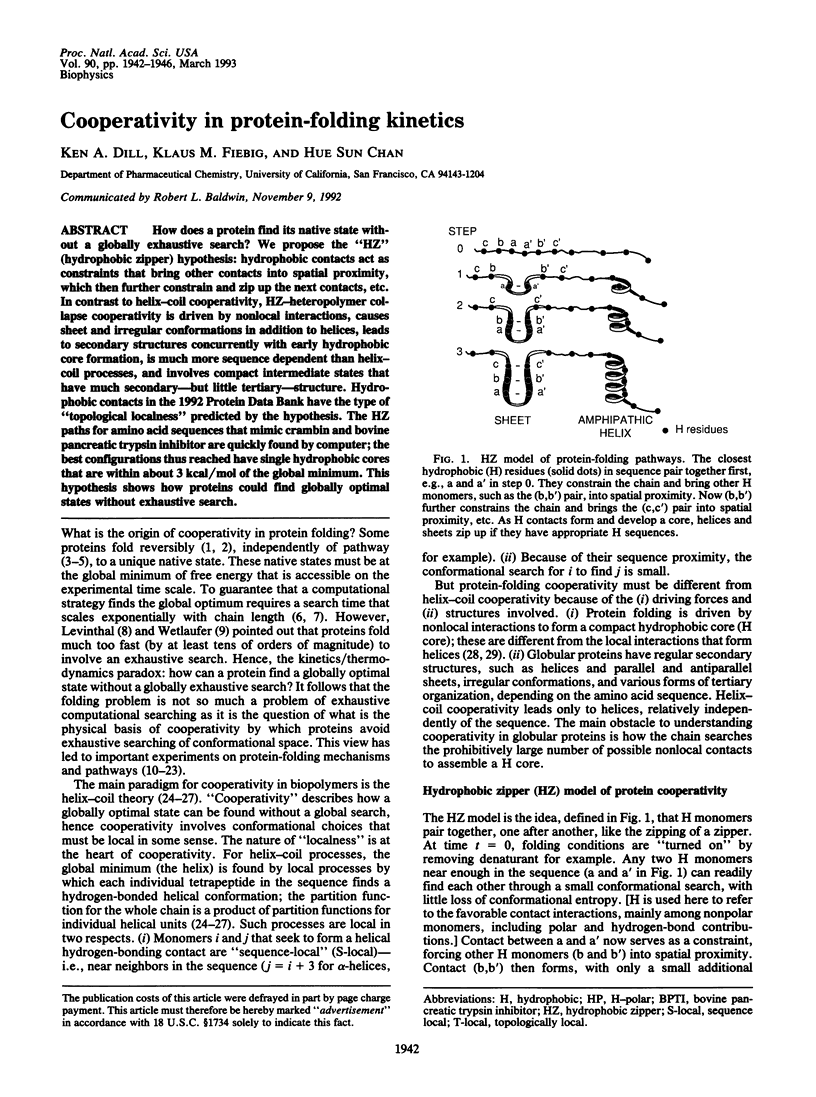
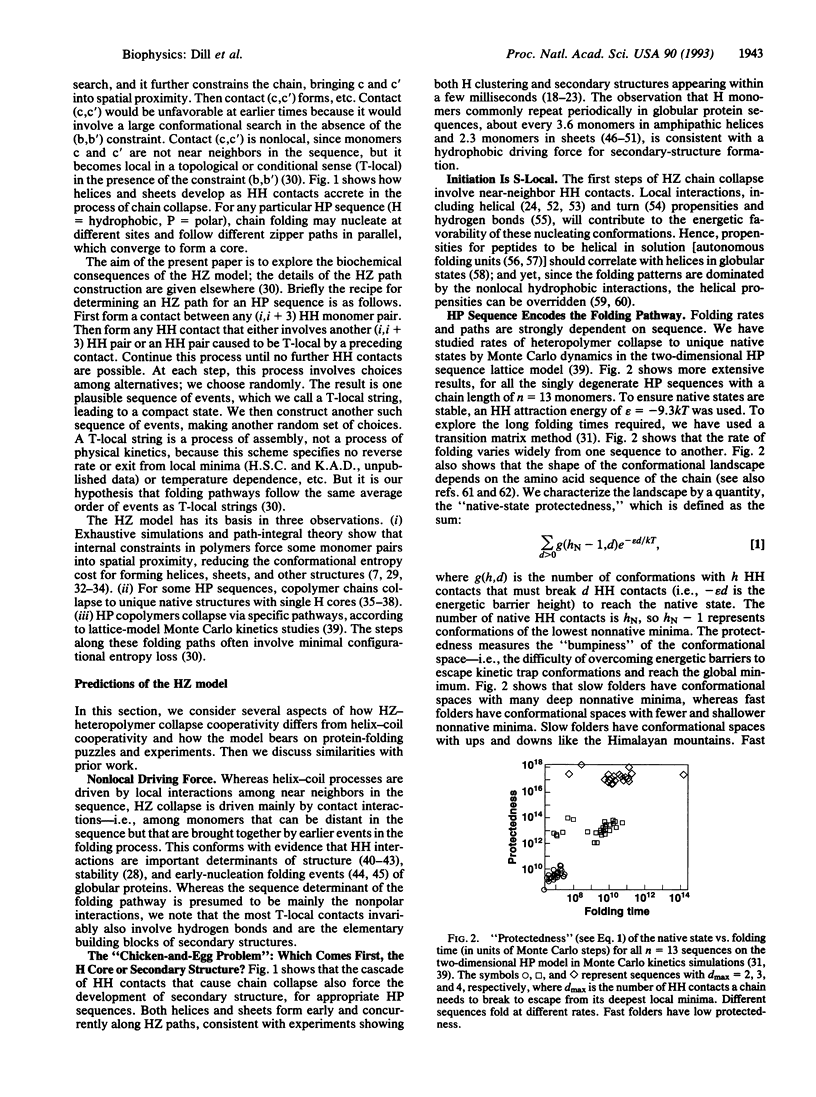
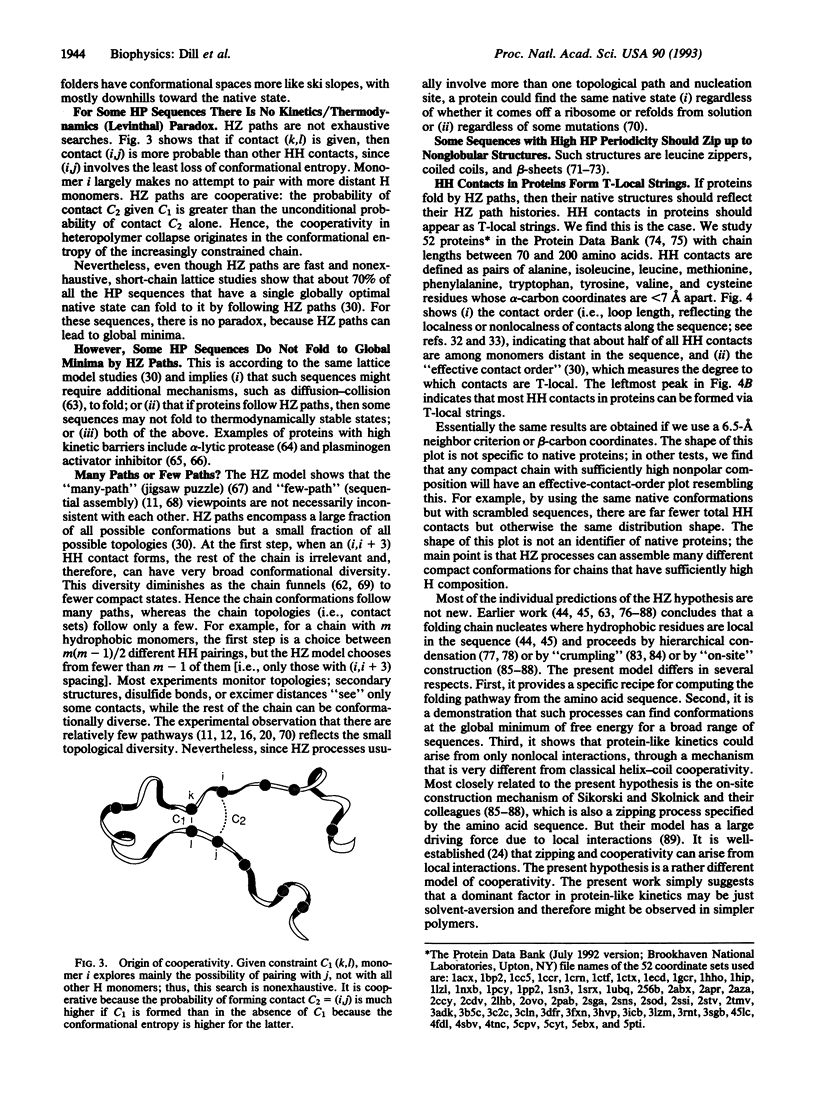
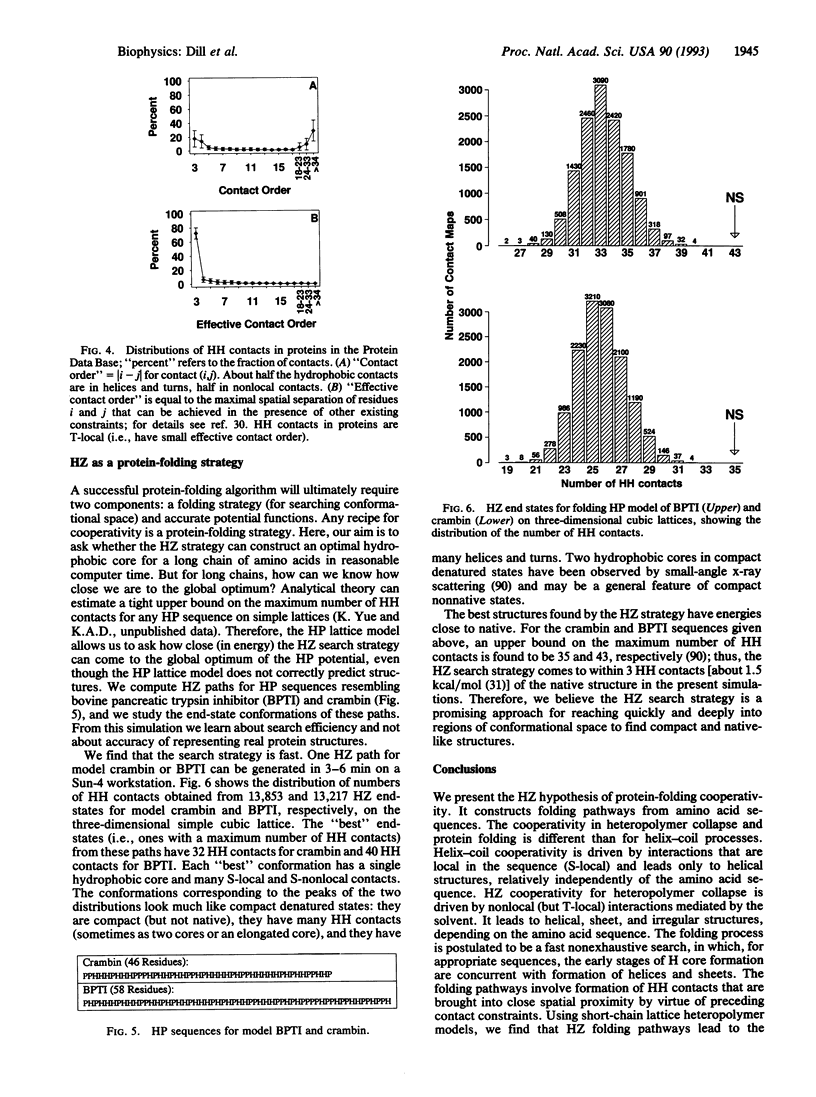
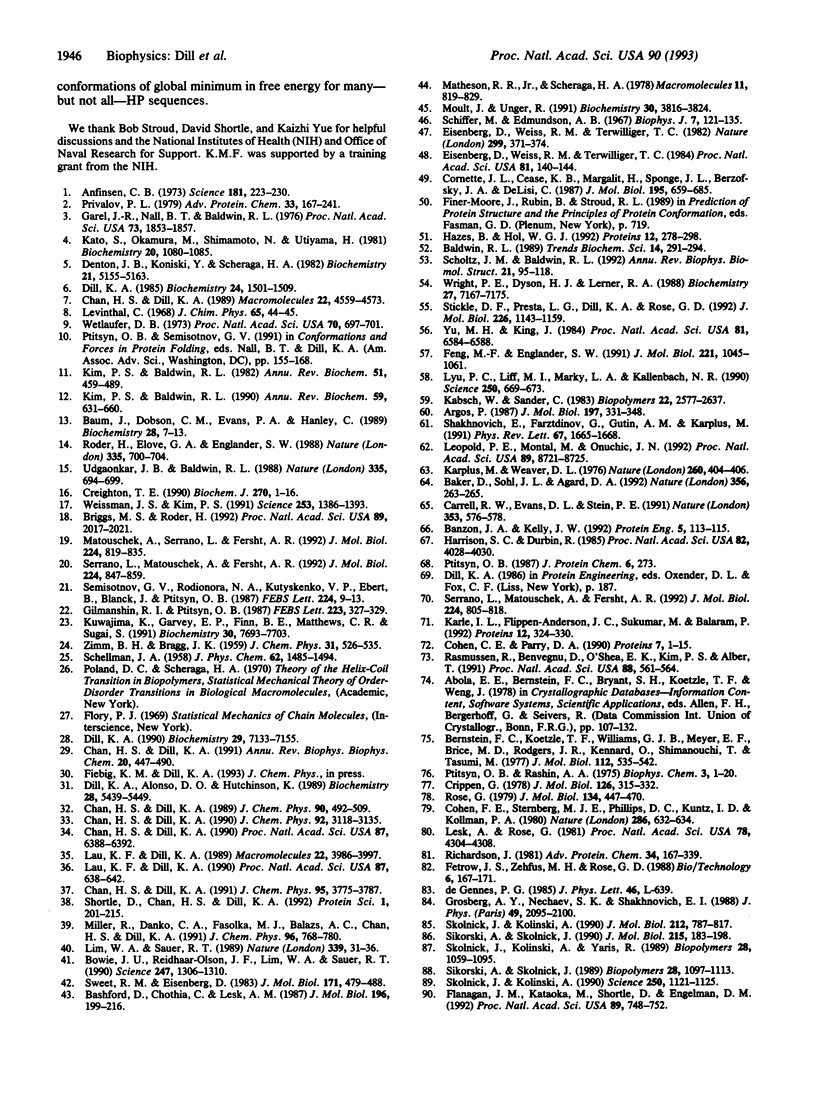
Images in this article
Selected References
These references are in PubMed. This may not be the complete list of references from this article.
- Anfinsen C. B. Principles that govern the folding of protein chains. Science. 1973 Jul 20;181(4096):223–230. doi: 10.1126/science.181.4096.223. [DOI] [PubMed] [Google Scholar]
- Argos P. Analysis of sequence-similar pentapeptides in unrelated protein tertiary structures. Strategies for protein folding and a guide for site-directed mutagenesis. J Mol Biol. 1987 Sep 20;197(2):331–348. doi: 10.1016/0022-2836(87)90127-6. [DOI] [PubMed] [Google Scholar]
- Baker D., Sohl J. L., Agard D. A. A protein-folding reaction under kinetic control. Nature. 1992 Mar 19;356(6366):263–265. doi: 10.1038/356263a0. [DOI] [PubMed] [Google Scholar]
- Baldwin R. L. How does protein folding get started? Trends Biochem Sci. 1989 Jul;14(7):291–294. doi: 10.1016/0968-0004(89)90067-4. [DOI] [PubMed] [Google Scholar]
- Banzon J. A., Kelly J. W. Beta-sheet rearrangements: serpins and beyond. Protein Eng. 1992 Mar;5(2):113–115. doi: 10.1093/protein/5.2.113. [DOI] [PubMed] [Google Scholar]
- Bashford D., Chothia C., Lesk A. M. Determinants of a protein fold. Unique features of the globin amino acid sequences. J Mol Biol. 1987 Jul 5;196(1):199–216. doi: 10.1016/0022-2836(87)90521-3. [DOI] [PubMed] [Google Scholar]
- Baum J., Dobson C. M., Evans P. A., Hanley C. Characterization of a partly folded protein by NMR methods: studies on the molten globule state of guinea pig alpha-lactalbumin. Biochemistry. 1989 Jan 10;28(1):7–13. doi: 10.1021/bi00427a002. [DOI] [PubMed] [Google Scholar]
- Bernstein F. C., Koetzle T. F., Williams G. J., Meyer E. F., Jr, Brice M. D., Rodgers J. R., Kennard O., Shimanouchi T., Tasumi M. The Protein Data Bank: a computer-based archival file for macromolecular structures. J Mol Biol. 1977 May 25;112(3):535–542. doi: 10.1016/s0022-2836(77)80200-3. [DOI] [PubMed] [Google Scholar]
- Bowie J. U., Reidhaar-Olson J. F., Lim W. A., Sauer R. T. Deciphering the message in protein sequences: tolerance to amino acid substitutions. Science. 1990 Mar 16;247(4948):1306–1310. doi: 10.1126/science.2315699. [DOI] [PubMed] [Google Scholar]
- Briggs M. S., Roder H. Early hydrogen-bonding events in the folding reaction of ubiquitin. Proc Natl Acad Sci U S A. 1992 Mar 15;89(6):2017–2021. doi: 10.1073/pnas.89.6.2017. [DOI] [PMC free article] [PubMed] [Google Scholar]
- Carrell R. W., Evans D. L., Stein P. E. Mobile reactive centre of serpins and the control of thrombosis. Nature. 1991 Oct 10;353(6344):576–578. doi: 10.1038/353576a0. [DOI] [PubMed] [Google Scholar]
- Chan H. S., Dill K. A. Origins of structure in globular proteins. Proc Natl Acad Sci U S A. 1990 Aug;87(16):6388–6392. doi: 10.1073/pnas.87.16.6388. [DOI] [PMC free article] [PubMed] [Google Scholar]
- Chan H. S., Dill K. A. Polymer principles in protein structure and stability. Annu Rev Biophys Biophys Chem. 1991;20:447–490. doi: 10.1146/annurev.bb.20.060191.002311. [DOI] [PubMed] [Google Scholar]
- Cohen C., Parry D. A. Alpha-helical coiled coils and bundles: how to design an alpha-helical protein. Proteins. 1990;7(1):1–15. doi: 10.1002/prot.340070102. [DOI] [PubMed] [Google Scholar]
- Cohen F. E., Sternberg M. J., Phillips D. C., Kuntz I. D., Kollman P. A. A diffusion--collision--adhesion model for the kinetics of myoglobin refolding. Nature. 1980 Aug 7;286(5773):632–634. doi: 10.1038/286632a0. [DOI] [PubMed] [Google Scholar]
- Cornette J. L., Cease K. B., Margalit H., Spouge J. L., Berzofsky J. A., DeLisi C. Hydrophobicity scales and computational techniques for detecting amphipathic structures in proteins. J Mol Biol. 1987 Jun 5;195(3):659–685. doi: 10.1016/0022-2836(87)90189-6. [DOI] [PubMed] [Google Scholar]
- Creighton T. E. Protein folding. Biochem J. 1990 Aug 15;270(1):1–16. doi: 10.1042/bj2700001. [DOI] [PMC free article] [PubMed] [Google Scholar]
- Crippen G. M. The tree structural organization of proteins. J Mol Biol. 1978 Dec 15;126(3):315–332. doi: 10.1016/0022-2836(78)90043-8. [DOI] [PubMed] [Google Scholar]
- Denton J. B., Konishi Y., Scheraga H. A. Folding of ribonuclease A from a partially disordered conformation. Kinetic study under folding conditions. Biochemistry. 1982 Oct 12;21(21):5155–5163. doi: 10.1021/bi00264a008. [DOI] [PubMed] [Google Scholar]
- Dill K. A., Alonso D. O., Hutchinson K. Thermal stabilities of globular proteins. Biochemistry. 1989 Jun 27;28(13):5439–5449. doi: 10.1021/bi00439a019. [DOI] [PubMed] [Google Scholar]
- Dill K. A. Dominant forces in protein folding. Biochemistry. 1990 Aug 7;29(31):7133–7155. doi: 10.1021/bi00483a001. [DOI] [PubMed] [Google Scholar]
- Dill K. A. Theory for the folding and stability of globular proteins. Biochemistry. 1985 Mar 12;24(6):1501–1509. doi: 10.1021/bi00327a032. [DOI] [PubMed] [Google Scholar]
- Eisenberg D., Weiss R. M., Terwilliger T. C. The helical hydrophobic moment: a measure of the amphiphilicity of a helix. Nature. 1982 Sep 23;299(5881):371–374. doi: 10.1038/299371a0. [DOI] [PubMed] [Google Scholar]
- Eisenberg D., Weiss R. M., Terwilliger T. C. The hydrophobic moment detects periodicity in protein hydrophobicity. Proc Natl Acad Sci U S A. 1984 Jan;81(1):140–144. doi: 10.1073/pnas.81.1.140. [DOI] [PMC free article] [PubMed] [Google Scholar]
- Flanagan J. M., Kataoka M., Shortle D., Engelman D. M. Truncated staphylococcal nuclease is compact but disordered. Proc Natl Acad Sci U S A. 1992 Jan 15;89(2):748–752. doi: 10.1073/pnas.89.2.748. [DOI] [PMC free article] [PubMed] [Google Scholar]
- Garel J. R., Nall B. T., Baldwin R. L. Guanidine-unfolded state of ribonuclease A contains both fast- and slow-refolding species. Proc Natl Acad Sci U S A. 1976 Jun;73(6):1853–1857. doi: 10.1073/pnas.73.6.1853. [DOI] [PMC free article] [PubMed] [Google Scholar]
- Gilmanshin R. I., Ptitsyn O. B. An early intermediate of refolding alpha-lactalbumin forms within 20 ms. FEBS Lett. 1987 Nov 2;223(2):327–329. doi: 10.1016/0014-5793(87)80313-7. [DOI] [PubMed] [Google Scholar]
- Harrison S. C., Durbin R. Is there a single pathway for the folding of a polypeptide chain? Proc Natl Acad Sci U S A. 1985 Jun;82(12):4028–4030. doi: 10.1073/pnas.82.12.4028. [DOI] [PMC free article] [PubMed] [Google Scholar]
- Hazes B., Hol W. G. Comparison of the hemocyanin beta-barrel with other Greek key beta-barrels: possible importance of the "beta-zipper" in protein structure and folding. Proteins. 1992 Mar;12(3):278–298. doi: 10.1002/prot.340120306. [DOI] [PubMed] [Google Scholar]
- Jeng M. F., Englander S. W. Stable submolecular folding units in a non-compact form of cytochrome c. J Mol Biol. 1991 Oct 5;221(3):1045–1061. doi: 10.1016/0022-2836(91)80191-v. [DOI] [PubMed] [Google Scholar]
- Kabsch W., Sander C. Dictionary of protein secondary structure: pattern recognition of hydrogen-bonded and geometrical features. Biopolymers. 1983 Dec;22(12):2577–2637. doi: 10.1002/bip.360221211. [DOI] [PubMed] [Google Scholar]
- Karle I. L., Flippen-Anderson J. L., Sukumar M., Balaram P. Helix packing of leucine-rich peptides: a parallel leucine ladder in the structure of Boc-Aib-Leu-Aib-Aib-Leu-Leu-Leu-Aib-Leu-Aib-OMe. Proteins. 1992 Apr;12(4):324–330. doi: 10.1002/prot.340120404. [DOI] [PubMed] [Google Scholar]
- Karplus M., Weaver D. L. Protein-folding dynamics. Nature. 1976 Apr 1;260(5550):404–406. doi: 10.1038/260404a0. [DOI] [PubMed] [Google Scholar]
- Kato S., Okamura M., Shimamoto N., Utiyama H. Spectral evidence for a rapidly formed structural intermediate in the refolding kinetics of hen egg-white lysozyme. Biochemistry. 1981 Mar 3;20(5):1080–1085. doi: 10.1021/bi00508a006. [DOI] [PubMed] [Google Scholar]
- Kim P. S., Baldwin R. L. Intermediates in the folding reactions of small proteins. Annu Rev Biochem. 1990;59:631–660. doi: 10.1146/annurev.bi.59.070190.003215. [DOI] [PubMed] [Google Scholar]
- Kim P. S., Baldwin R. L. Specific intermediates in the folding reactions of small proteins and the mechanism of protein folding. Annu Rev Biochem. 1982;51:459–489. doi: 10.1146/annurev.bi.51.070182.002331. [DOI] [PubMed] [Google Scholar]
- Kuwajima K., Garvey E. P., Finn B. E., Matthews C. R., Sugai S. Transient intermediates in the folding of dihydrofolate reductase as detected by far-ultraviolet circular dichroism spectroscopy. Biochemistry. 1991 Aug 6;30(31):7693–7703. doi: 10.1021/bi00245a005. [DOI] [PubMed] [Google Scholar]
- Lau K. F., Dill K. A. Theory for protein mutability and biogenesis. Proc Natl Acad Sci U S A. 1990 Jan;87(2):638–642. doi: 10.1073/pnas.87.2.638. [DOI] [PMC free article] [PubMed] [Google Scholar]
- Leopold P. E., Montal M., Onuchic J. N. Protein folding funnels: a kinetic approach to the sequence-structure relationship. Proc Natl Acad Sci U S A. 1992 Sep 15;89(18):8721–8725. doi: 10.1073/pnas.89.18.8721. [DOI] [PMC free article] [PubMed] [Google Scholar]
- Lesk A. M., Rose G. D. Folding units in globular proteins. Proc Natl Acad Sci U S A. 1981 Jul;78(7):4304–4308. doi: 10.1073/pnas.78.7.4304. [DOI] [PMC free article] [PubMed] [Google Scholar]
- Lim W. A., Sauer R. T. Alternative packing arrangements in the hydrophobic core of lambda repressor. Nature. 1989 May 4;339(6219):31–36. doi: 10.1038/339031a0. [DOI] [PubMed] [Google Scholar]
- Lyu P. C., Liff M. I., Marky L. A., Kallenbach N. R. Side chain contributions to the stability of alpha-helical structure in peptides. Science. 1990 Nov 2;250(4981):669–673. doi: 10.1126/science.2237416. [DOI] [PubMed] [Google Scholar]
- Matouschek A., Serrano L., Fersht A. R. The folding of an enzyme. IV. Structure of an intermediate in the refolding of barnase analysed by a protein engineering procedure. J Mol Biol. 1992 Apr 5;224(3):819–835. doi: 10.1016/0022-2836(92)90564-z. [DOI] [PubMed] [Google Scholar]
- Moult J., Unger R. An analysis of protein folding pathways. Biochemistry. 1991 Apr 23;30(16):3816–3824. doi: 10.1021/bi00230a003. [DOI] [PubMed] [Google Scholar]
- Privalov P. L. Stability of proteins: small globular proteins. Adv Protein Chem. 1979;33:167–241. doi: 10.1016/s0065-3233(08)60460-x. [DOI] [PubMed] [Google Scholar]
- Ptitsyn O. B., Rashin A. A. A model of myoglobin self-organization. Biophys Chem. 1975 Feb;3(1):1–20. doi: 10.1016/0301-4622(75)80033-0. [DOI] [PubMed] [Google Scholar]
- Rasmussen R., Benvegnu D., O'Shea E. K., Kim P. S., Alber T. X-ray scattering indicates that the leucine zipper is a coiled coil. Proc Natl Acad Sci U S A. 1991 Jan 15;88(2):561–564. doi: 10.1073/pnas.88.2.561. [DOI] [PMC free article] [PubMed] [Google Scholar]
- Richardson J. S. The anatomy and taxonomy of protein structure. Adv Protein Chem. 1981;34:167–339. doi: 10.1016/s0065-3233(08)60520-3. [DOI] [PubMed] [Google Scholar]
- Roder H., Elöve G. A., Englander S. W. Structural characterization of folding intermediates in cytochrome c by H-exchange labelling and proton NMR. Nature. 1988 Oct 20;335(6192):700–704. doi: 10.1038/335700a0. [DOI] [PMC free article] [PubMed] [Google Scholar]
- Rose G. D. Hierarchic organization of domains in globular proteins. J Mol Biol. 1979 Nov 5;134(3):447–470. doi: 10.1016/0022-2836(79)90363-2. [DOI] [PubMed] [Google Scholar]
- Schiffer M., Edmundson A. B. Use of helical wheels to represent the structures of proteins and to identify segments with helical potential. Biophys J. 1967 Mar;7(2):121–135. doi: 10.1016/S0006-3495(67)86579-2. [DOI] [PMC free article] [PubMed] [Google Scholar]
- Scholtz J. M., Baldwin R. L. The mechanism of alpha-helix formation by peptides. Annu Rev Biophys Biomol Struct. 1992;21:95–118. doi: 10.1146/annurev.bb.21.060192.000523. [DOI] [PubMed] [Google Scholar]
- Semisotnov G. V., Rodionova N. A., Kutyshenko V. P., Ebert B., Blanck J., Ptitsyn O. B. Sequential mechanism of refolding of carbonic anhydrase B. FEBS Lett. 1987 Nov 16;224(1):9–13. doi: 10.1016/0014-5793(87)80412-x. [DOI] [PubMed] [Google Scholar]
- Serrano L., Matouschek A., Fersht A. R. The folding of an enzyme. III. Structure of the transition state for unfolding of barnase analysed by a protein engineering procedure. J Mol Biol. 1992 Apr 5;224(3):805–818. doi: 10.1016/0022-2836(92)90563-y. [DOI] [PubMed] [Google Scholar]
- Serrano L., Matouschek A., Fersht A. R. The folding of an enzyme. VI. The folding pathway of barnase: comparison with theoretical models. J Mol Biol. 1992 Apr 5;224(3):847–859. doi: 10.1016/0022-2836(92)90566-3. [DOI] [PubMed] [Google Scholar]
- Shakhnovich E, Farztdinov G, Gutin AM, Karplus M. Protein folding bottlenecks: A lattice Monte Carlo simulation. Phys Rev Lett. 1991 Sep 16;67(12):1665–1668. doi: 10.1103/PhysRevLett.67.1665. [DOI] [PubMed] [Google Scholar]
- Shortle D., Chan H. S., Dill K. A. Modeling the effects of mutations on the denatured states of proteins. Protein Sci. 1992 Feb;1(2):201–215. doi: 10.1002/pro.5560010202. [DOI] [PMC free article] [PubMed] [Google Scholar]
- Sikorski A., Skolnick J. Dynamic Monte Carlo simulations of globular protein folding. Model studies of in vivo assembly of four helix bundles and four member beta-barrels. J Mol Biol. 1990 Sep 5;215(1):183–198. doi: 10.1016/s0022-2836(05)80103-2. [DOI] [PubMed] [Google Scholar]
- Sikorski A., Skolnick J. Monte Carlo studies on equilibrium globular protein folding. III. The four helix bundle. Biopolymers. 1989 Jun;28(6):1097–1113. doi: 10.1002/bip.360280605. [DOI] [PubMed] [Google Scholar]
- Skolnick J., Kolinski A. Dynamic Monte Carlo simulations of globular protein folding/unfolding pathways. I. Six-member, Greek key beta-barrel proteins. J Mol Biol. 1990 Apr 20;212(4):787–817. doi: 10.1016/0022-2836(90)90237-g. [DOI] [PubMed] [Google Scholar]
- Skolnick J., Kolinski A. Simulations of the folding of a globular protein. Science. 1990 Nov 23;250(4984):1121–1125. doi: 10.1126/science.250.4984.1121. [DOI] [PubMed] [Google Scholar]
- Skolnick J., Kolinski A., Yaris R. Monte Carlo studies on equilibrium globular protein folding. II. Beta-barrel globular protein models. Biopolymers. 1989 Jun;28(6):1059–1095. doi: 10.1002/bip.360280604. [DOI] [PubMed] [Google Scholar]
- Stickle D. F., Presta L. G., Dill K. A., Rose G. D. Hydrogen bonding in globular proteins. J Mol Biol. 1992 Aug 20;226(4):1143–1159. doi: 10.1016/0022-2836(92)91058-w. [DOI] [PubMed] [Google Scholar]
- Sweet R. M., Eisenberg D. Correlation of sequence hydrophobicities measures similarity in three-dimensional protein structure. J Mol Biol. 1983 Dec 25;171(4):479–488. doi: 10.1016/0022-2836(83)90041-4. [DOI] [PubMed] [Google Scholar]
- Udgaonkar J. B., Baldwin R. L. NMR evidence for an early framework intermediate on the folding pathway of ribonuclease A. Nature. 1988 Oct 20;335(6192):694–699. doi: 10.1038/335694a0. [DOI] [PubMed] [Google Scholar]
- Weissman J. S., Kim P. S. Reexamination of the folding of BPTI: predominance of native intermediates. Science. 1991 Sep 20;253(5026):1386–1393. doi: 10.1126/science.1716783. [DOI] [PubMed] [Google Scholar]
- Wetlaufer D. B. Nucleation, rapid folding, and globular intrachain regions in proteins. Proc Natl Acad Sci U S A. 1973 Mar;70(3):697–701. doi: 10.1073/pnas.70.3.697. [DOI] [PMC free article] [PubMed] [Google Scholar]
- Wright P. E., Dyson H. J., Lerner R. A. Conformation of peptide fragments of proteins in aqueous solution: implications for initiation of protein folding. Biochemistry. 1988 Sep 20;27(19):7167–7175. doi: 10.1021/bi00419a001. [DOI] [PubMed] [Google Scholar]
- Yu M. H., King J. Single amino acid substitutions influencing the folding pathway of the phage P22 tail spike endorhamnosidase. Proc Natl Acad Sci U S A. 1984 Nov;81(21):6584–6588. doi: 10.1073/pnas.81.21.6584. [DOI] [PMC free article] [PubMed] [Google Scholar]