Abstract
During muscle contraction, work is generated when a myosin cross-bridge attaches to an actin filament and exerts a force on it through some power-stroke distance, h. At the end of this power stroke, attached myosin heads are carried into regions where they exert a negative force on the actin filament (the drag stroke) and where they are released rapidly from actin by ATP binding. Although the length of the power stroke remains controversial, average distance traversed in the drag-stroke region can be determined when one knows both rate of cross-bridge dissociation and filament-sliding velocity. At maximum contraction velocity, the average force exerted in the drag stroke must balance that exerted in the power stroke. We discuss here a simple model of cross-bridge interaction that allows one to calculate the force exerted in the drag stroke and to relate this to the power-stroke distance h traversed by cross-bridges in the positive-force region. Both the rate at which myosin can be dissociated from actin and the velocity at which an actin filament can be translated have been measured for a series of myosin isozymes and for different substrates, producing a wide range of values for each. Nonetheless, we show here that the rate of myosin dissociation from actin correlates well with the velocity of filament sliding, providing support for the simple model presented and suggesting that the power stroke is approximately 10 nm in length.
Full text
PDF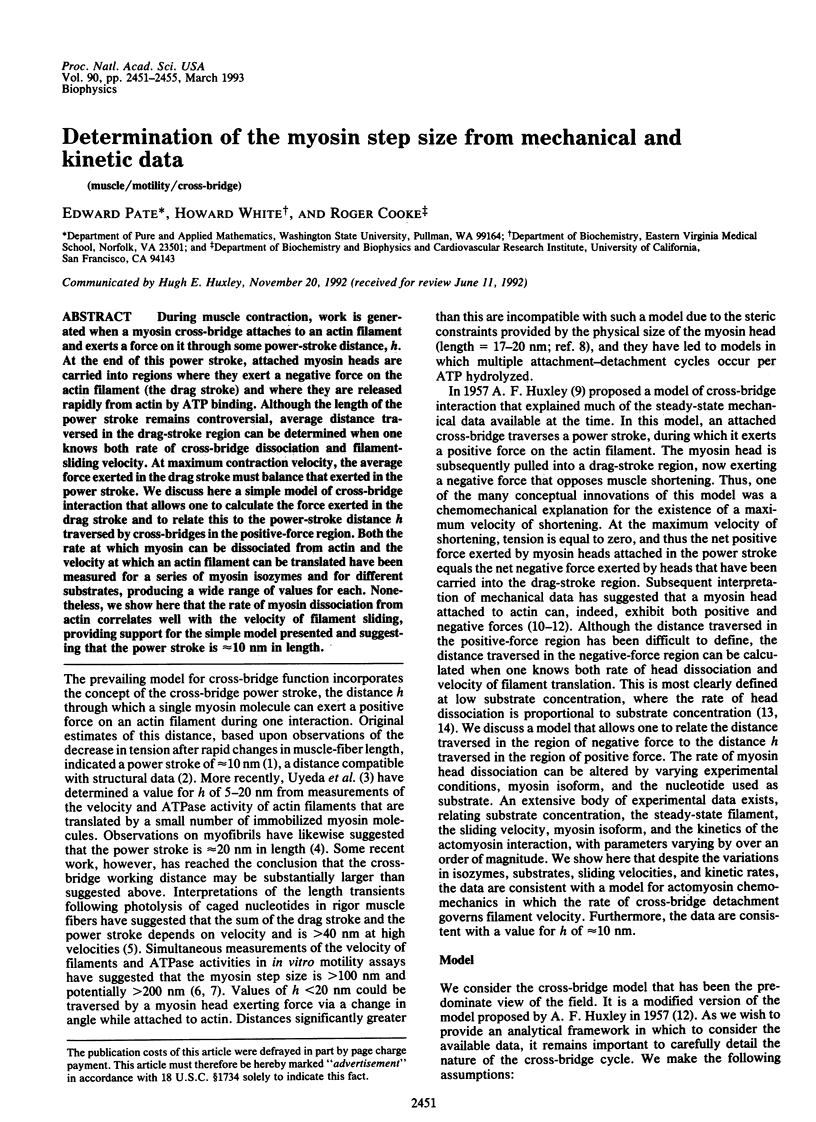
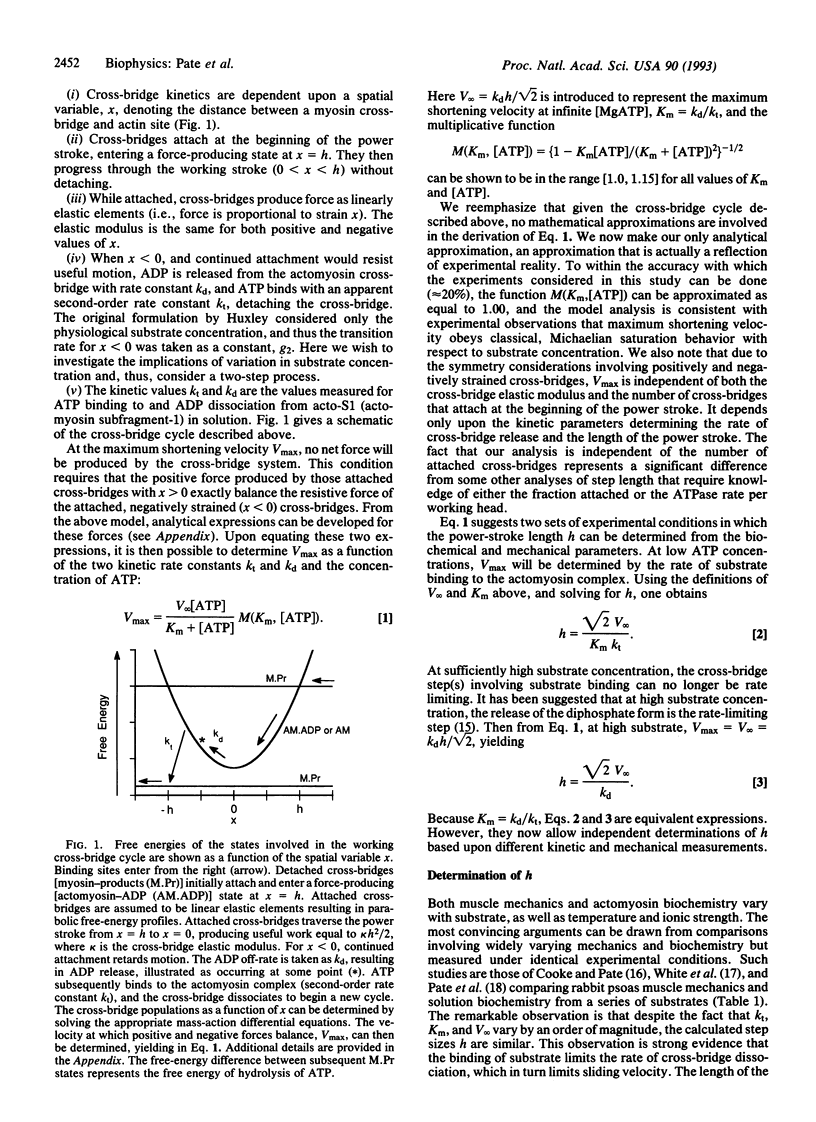
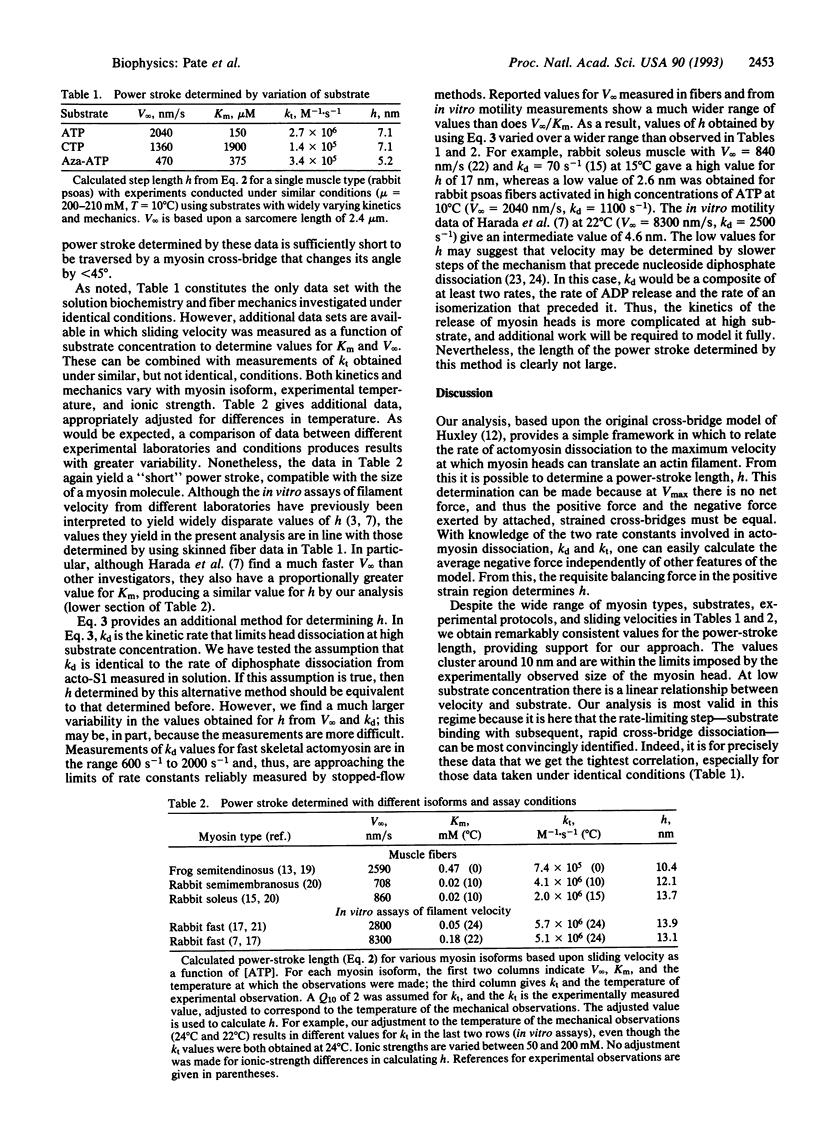
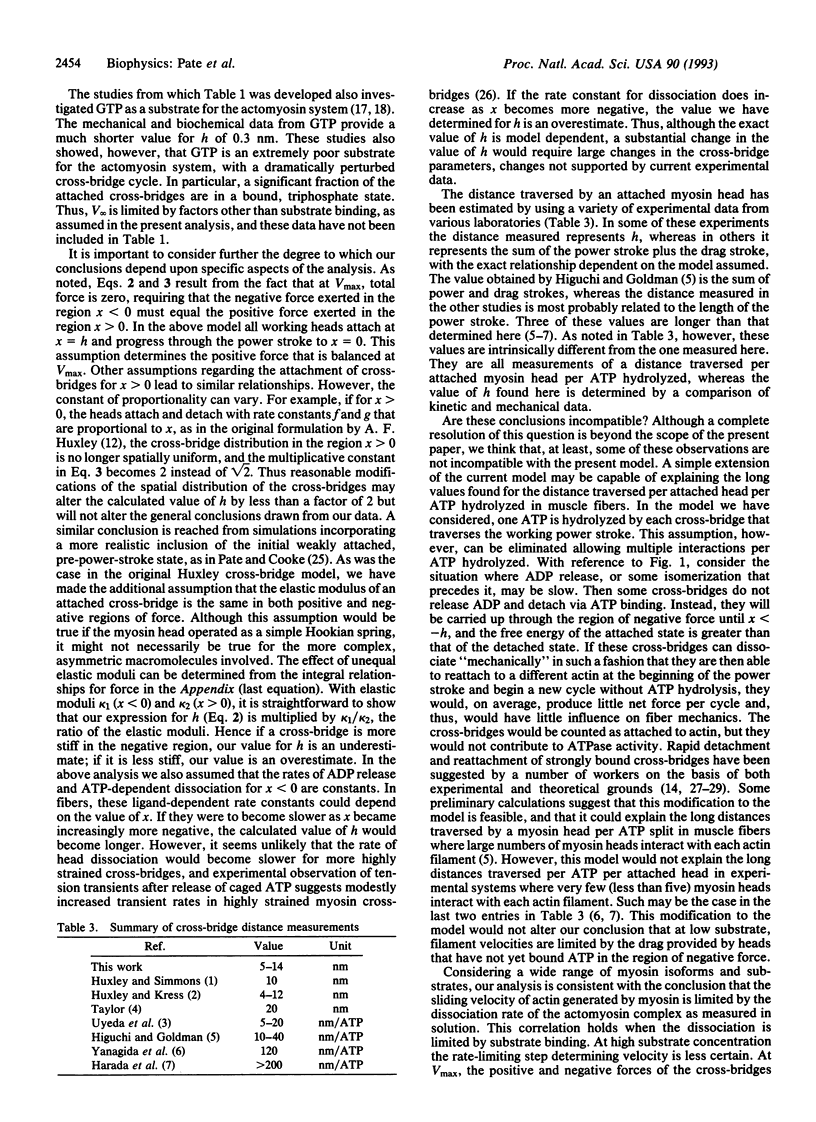
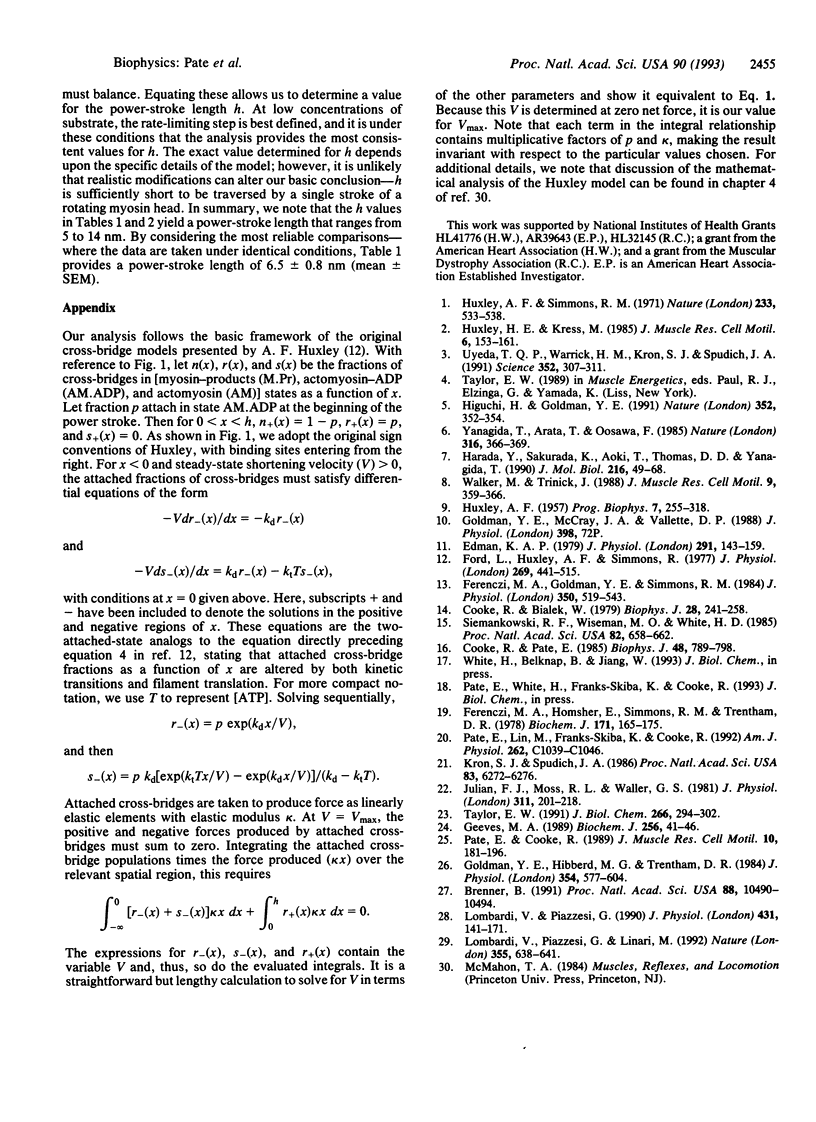
Selected References
These references are in PubMed. This may not be the complete list of references from this article.
- Brenner B. Rapid dissociation and reassociation of actomyosin cross-bridges during force generation: a newly observed facet of cross-bridge action in muscle. Proc Natl Acad Sci U S A. 1991 Dec 1;88(23):10490–10494. doi: 10.1073/pnas.88.23.10490. [DOI] [PMC free article] [PubMed] [Google Scholar]
- Cooke R., Bialek W. Contraction of glycerinated muscle fibers as a function of the ATP concentration. Biophys J. 1979 Nov;28(2):241–258. doi: 10.1016/S0006-3495(79)85174-7. [DOI] [PMC free article] [PubMed] [Google Scholar]
- Cooke R., Pate E. The effects of ADP and phosphate on the contraction of muscle fibers. Biophys J. 1985 Nov;48(5):789–798. doi: 10.1016/S0006-3495(85)83837-6. [DOI] [PMC free article] [PubMed] [Google Scholar]
- Edman K. A. The velocity of unloaded shortening and its relation to sarcomere length and isometric force in vertebrate muscle fibres. J Physiol. 1979 Jun;291:143–159. doi: 10.1113/jphysiol.1979.sp012804. [DOI] [PMC free article] [PubMed] [Google Scholar]
- Ferenczi M. A., Goldman Y. E., Simmons R. M. The dependence of force and shortening velocity on substrate concentration in skinned muscle fibres from Rana temporaria. J Physiol. 1984 May;350:519–543. doi: 10.1113/jphysiol.1984.sp015216. [DOI] [PMC free article] [PubMed] [Google Scholar]
- Ferenczi M. A., Homsher E., Simmons R. M., Trentham D. R. Reaction mechanism of the magnesium ion-dependent adenosine triphosphatase of frog muscle myosin and subfragment 1. Biochem J. 1978 Apr 1;171(1):165–175. doi: 10.1042/bj1710165. [DOI] [PMC free article] [PubMed] [Google Scholar]
- Ford L. E., Huxley A. F., Simmons R. M. Tension responses to sudden length change in stimulated frog muscle fibres near slack length. J Physiol. 1977 Jul;269(2):441–515. doi: 10.1113/jphysiol.1977.sp011911. [DOI] [PMC free article] [PubMed] [Google Scholar]
- Geeves M. A., Jeffries T. E. The effect of nucleotide upon a specific isomerization of actomyosin subfragment 1. Biochem J. 1988 Nov 15;256(1):41–46. doi: 10.1042/bj2560041. [DOI] [PMC free article] [PubMed] [Google Scholar]
- Goldman Y. E., Hibberd M. G., Trentham D. R. Relaxation of rabbit psoas muscle fibres from rigor by photochemical generation of adenosine-5'-triphosphate. J Physiol. 1984 Sep;354:577–604. doi: 10.1113/jphysiol.1984.sp015394. [DOI] [PMC free article] [PubMed] [Google Scholar]
- HUXLEY A. F. Muscle structure and theories of contraction. Prog Biophys Biophys Chem. 1957;7:255–318. [PubMed] [Google Scholar]
- Harada Y., Sakurada K., Aoki T., Thomas D. D., Yanagida T. Mechanochemical coupling in actomyosin energy transduction studied by in vitro movement assay. J Mol Biol. 1990 Nov 5;216(1):49–68. doi: 10.1016/S0022-2836(05)80060-9. [DOI] [PubMed] [Google Scholar]
- Higuchi H., Goldman Y. E. Sliding distance between actin and myosin filaments per ATP molecule hydrolysed in skinned muscle fibres. Nature. 1991 Jul 25;352(6333):352–354. doi: 10.1038/352352a0. [DOI] [PubMed] [Google Scholar]
- Huxley A. F., Simmons R. M. Proposed mechanism of force generation in striated muscle. Nature. 1971 Oct 22;233(5321):533–538. doi: 10.1038/233533a0. [DOI] [PubMed] [Google Scholar]
- Huxley H. E., Kress M. Crossbridge behaviour during muscle contraction. J Muscle Res Cell Motil. 1985 Apr;6(2):153–161. doi: 10.1007/BF00713057. [DOI] [PubMed] [Google Scholar]
- Julian F. J., Moss R. L., Waller G. S. Mechanical properties and myosin light chain composition of skinned muscle fibres from adult and new-born rabbits. J Physiol. 1981 Feb;311:201–218. doi: 10.1113/jphysiol.1981.sp013581. [DOI] [PMC free article] [PubMed] [Google Scholar]
- Kron S. J., Spudich J. A. Fluorescent actin filaments move on myosin fixed to a glass surface. Proc Natl Acad Sci U S A. 1986 Sep;83(17):6272–6276. doi: 10.1073/pnas.83.17.6272. [DOI] [PMC free article] [PubMed] [Google Scholar]
- Lombardi V., Piazzesi G., Linari M. Rapid regeneration of the actin-myosin power stroke in contracting muscle. Nature. 1992 Feb 13;355(6361):638–641. doi: 10.1038/355638a0. [DOI] [PubMed] [Google Scholar]
- Lombardi V., Piazzesi G. The contractile response during steady lengthening of stimulated frog muscle fibres. J Physiol. 1990 Dec;431:141–171. doi: 10.1113/jphysiol.1990.sp018324. [DOI] [PMC free article] [PubMed] [Google Scholar]
- Pate E., Cooke R. A model of crossbridge action: the effects of ATP, ADP and Pi. J Muscle Res Cell Motil. 1989 Jun;10(3):181–196. doi: 10.1007/BF01739809. [DOI] [PubMed] [Google Scholar]
- Pate E., Lin M., Franks-Skiba K., Cooke R. Contraction of glycerinated rabbit slow-twitch muscle fibers as a function of MgATP concentration. Am J Physiol. 1992 Apr;262(4 Pt 1):C1039–C1046. doi: 10.1152/ajpcell.1992.262.4.C1039. [DOI] [PubMed] [Google Scholar]
- Siemankowski R. F., Wiseman M. O., White H. D. ADP dissociation from actomyosin subfragment 1 is sufficiently slow to limit the unloaded shortening velocity in vertebrate muscle. Proc Natl Acad Sci U S A. 1985 Feb;82(3):658–662. doi: 10.1073/pnas.82.3.658. [DOI] [PMC free article] [PubMed] [Google Scholar]
- Taylor E. W. Kinetic studies on the association and dissociation of myosin subfragment 1 and actin. J Biol Chem. 1991 Jan 5;266(1):294–302. [PubMed] [Google Scholar]
- Uyeda T. Q., Warrick H. M., Kron S. J., Spudich J. A. Quantized velocities at low myosin densities in an in vitro motility assay. Nature. 1991 Jul 25;352(6333):307–311. doi: 10.1038/352307a0. [DOI] [PubMed] [Google Scholar]
- Walker M., Trinick J. Visualization of domains in native and nucleotide-trapped myosin heads by negative staining. J Muscle Res Cell Motil. 1988 Aug;9(4):359–366. doi: 10.1007/BF01773879. [DOI] [PubMed] [Google Scholar]
- Yanagida T., Arata T., Oosawa F. Sliding distance of actin filament induced by a myosin crossbridge during one ATP hydrolysis cycle. Nature. 1985 Jul 25;316(6026):366–369. doi: 10.1038/316366a0. [DOI] [PubMed] [Google Scholar]