Abstract
The efficiency of fluorescence resonance energy transfer (FRET) between fluorescein and rhodamine covalently attached to both 5' termini of a series of double-stranded DNA species (ranging from 8 to 20 bp) was measured. FRET efficiency varied with a dependence compatible with dye-to-dye distances (R) calculated on the basis of double-stranded B-DNA structure; the helical geometry of double-stranded DNA in solution is clearly evident. The experimental data were consistent with a 1/[1 + (R/R0)6] dependence of FRET efficiency characteristic for the Förster dipole-dipole mechanism. The thermal dissociation of the strands of the duplex DNA species can be followed by using FRET, and from these data we have been able to obtain enthalpies of duplex formation in good agreement with earlier measurements using alternative techniques. FRET measurements at very different salt concentrations can be accurately compared. We conclude that FRET is a reliable and valuable method for studying structure and conformational transitions in nucleic acids.
Full text
PDF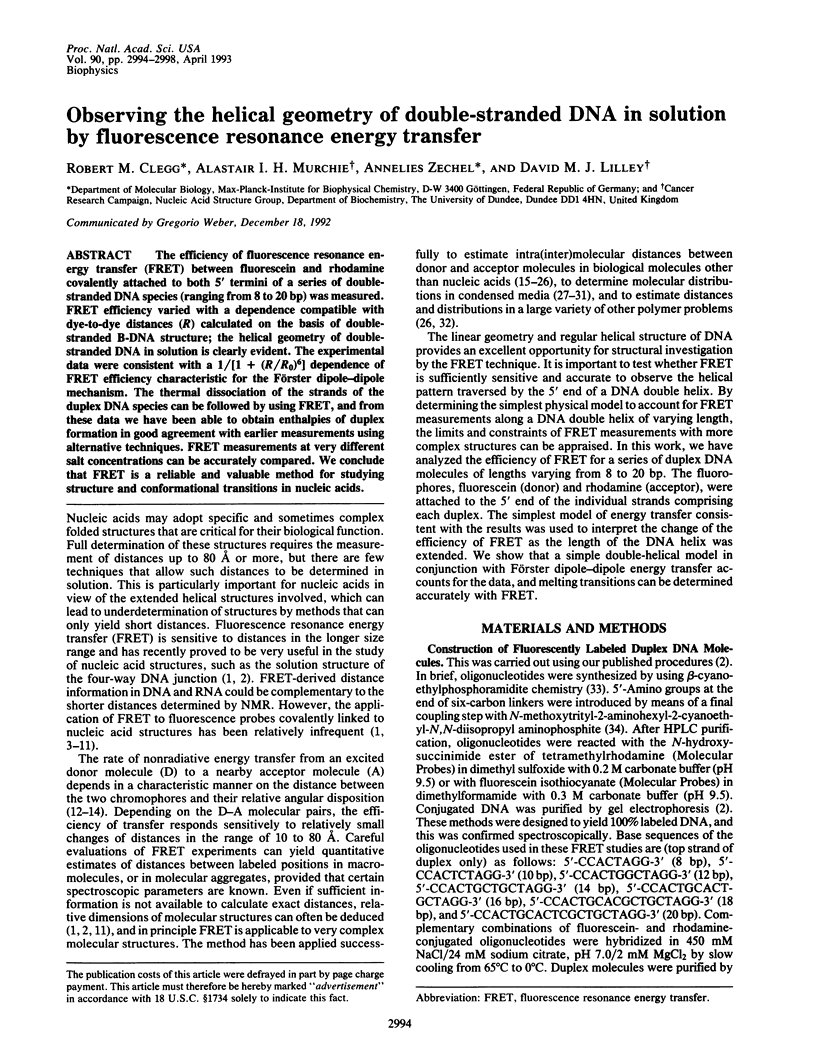
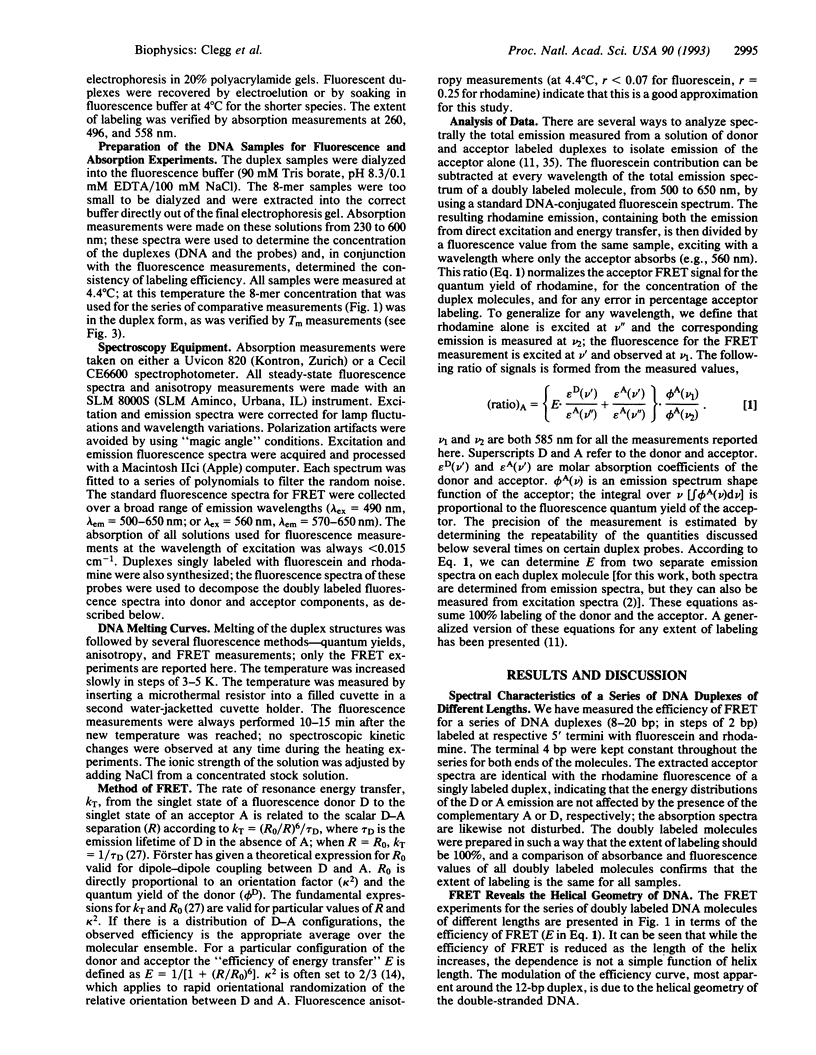
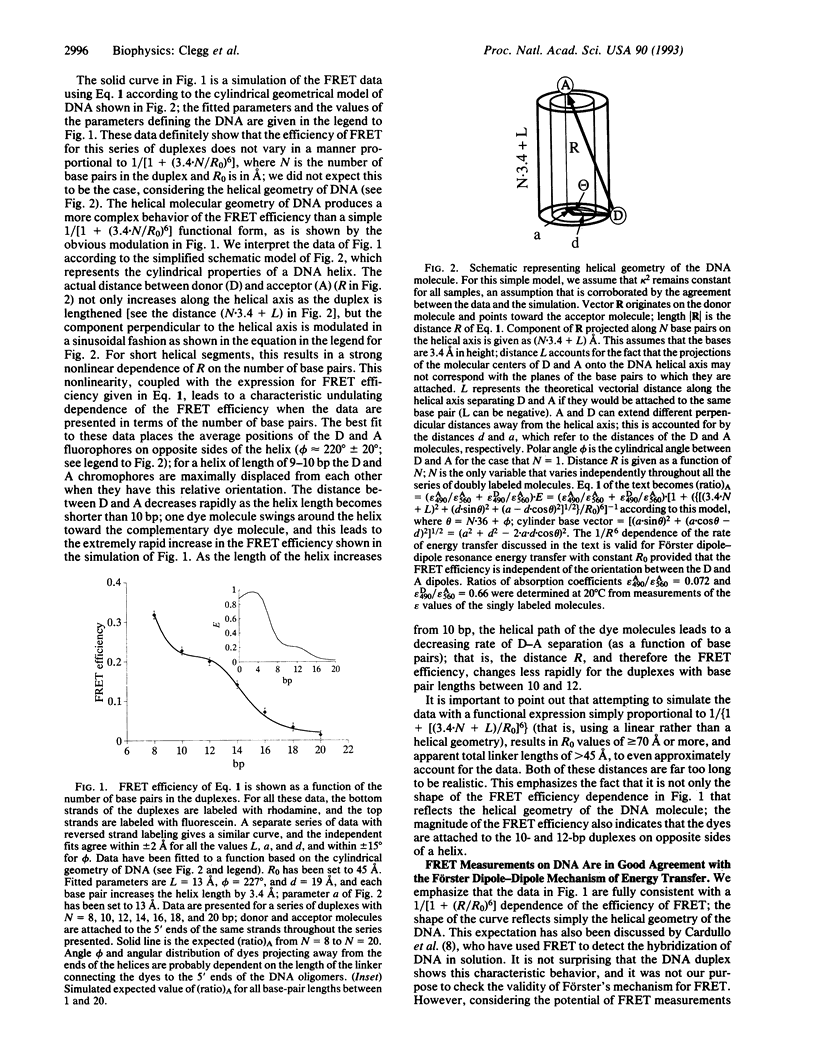
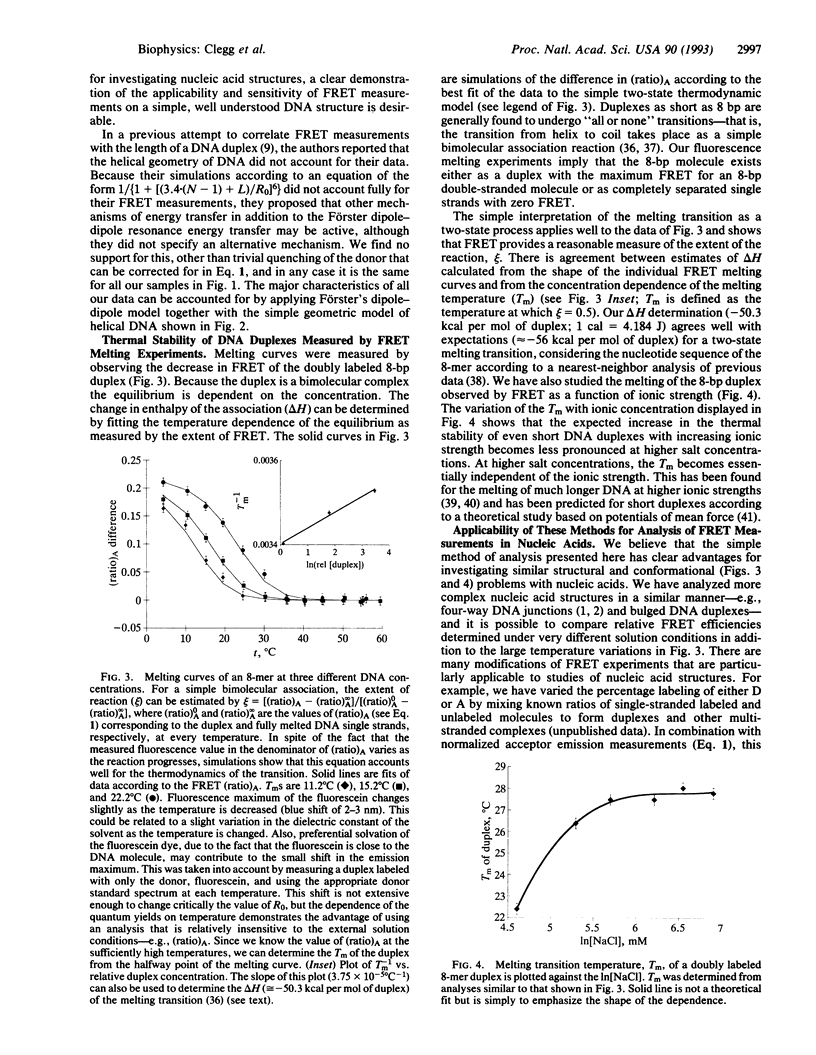
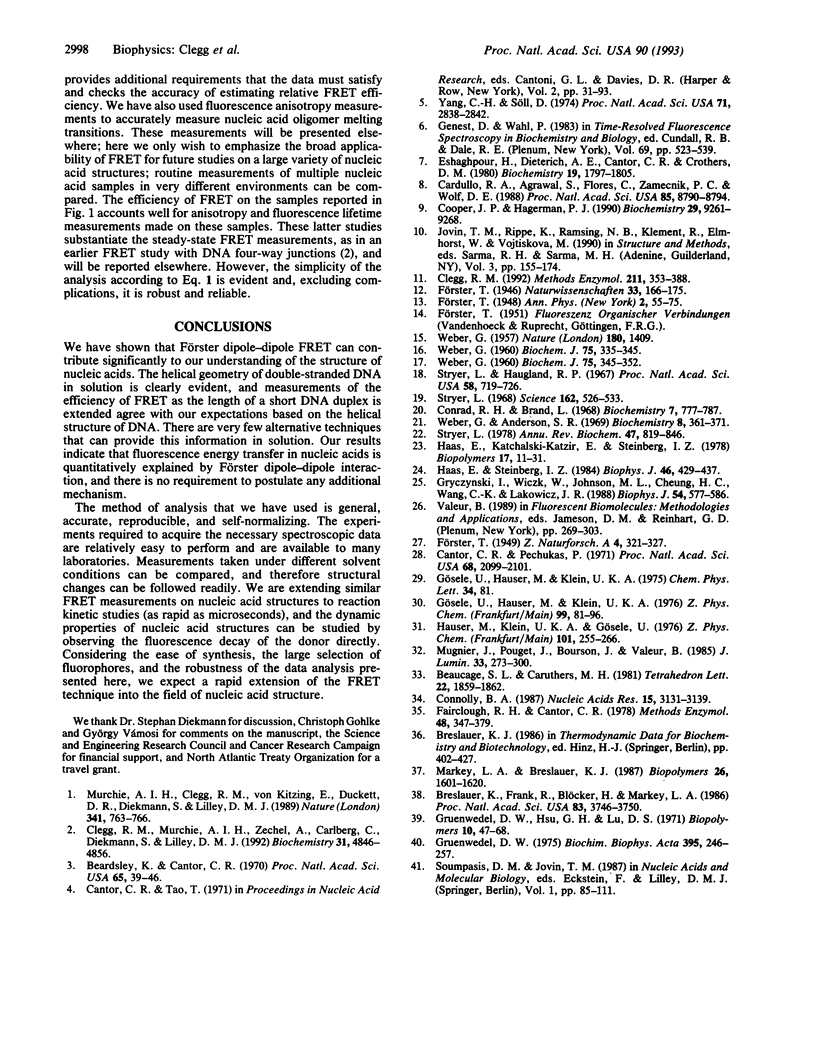
Selected References
These references are in PubMed. This may not be the complete list of references from this article.
- Beardsley K., Cantor C. R. Studies of transfer RNA tertiary structure by singlet-singlet energy transfer. Proc Natl Acad Sci U S A. 1970 Jan;65(1):39–46. doi: 10.1073/pnas.65.1.39. [DOI] [PMC free article] [PubMed] [Google Scholar]
- Breslauer K. J., Frank R., Blöcker H., Marky L. A. Predicting DNA duplex stability from the base sequence. Proc Natl Acad Sci U S A. 1986 Jun;83(11):3746–3750. doi: 10.1073/pnas.83.11.3746. [DOI] [PMC free article] [PubMed] [Google Scholar]
- Cantor C. R., Pechukas P. Determination of distance distribution functions by singlet-singlet energy transfer. Proc Natl Acad Sci U S A. 1971 Sep;68(9):2099–2101. doi: 10.1073/pnas.68.9.2099. [DOI] [PMC free article] [PubMed] [Google Scholar]
- Cardullo R. A., Agrawal S., Flores C., Zamecnik P. C., Wolf D. E. Detection of nucleic acid hybridization by nonradiative fluorescence resonance energy transfer. Proc Natl Acad Sci U S A. 1988 Dec;85(23):8790–8794. doi: 10.1073/pnas.85.23.8790. [DOI] [PMC free article] [PubMed] [Google Scholar]
- Clegg R. M. Fluorescence resonance energy transfer and nucleic acids. Methods Enzymol. 1992;211:353–388. doi: 10.1016/0076-6879(92)11020-j. [DOI] [PubMed] [Google Scholar]
- Clegg R. M., Murchie A. I., Zechel A., Carlberg C., Diekmann S., Lilley D. M. Fluorescence resonance energy transfer analysis of the structure of the four-way DNA junction. Biochemistry. 1992 May 26;31(20):4846–4856. doi: 10.1021/bi00135a016. [DOI] [PubMed] [Google Scholar]
- Connolly B. A. The synthesis of oligonucleotides containing a primary amino group at the 5'-terminus. Nucleic Acids Res. 1987 Apr 10;15(7):3131–3139. doi: 10.1093/nar/15.7.3131. [DOI] [PMC free article] [PubMed] [Google Scholar]
- Conrad R. H., Brand L. Intramolecular transfer of excitation from tryptophan to 1-dimethylaminonaphthalene 5-sulfonamide in a series of model compounds. Biochemistry. 1968 Feb;7(2):777–787. doi: 10.1021/bi00842a036. [DOI] [PubMed] [Google Scholar]
- Cooper J. P., Hagerman P. J. Analysis of fluorescence energy transfer in duplex and branched DNA molecules. Biochemistry. 1990 Oct 2;29(39):9261–9268. doi: 10.1021/bi00491a022. [DOI] [PubMed] [Google Scholar]
- Eshaghpour H., Dieterich A. E., Cantor C. R., Crothers D. M. Singlet-singlet energy transfer studies of the internal organization of nucleosomes. Biochemistry. 1980 Apr 29;19(9):1797–1805. doi: 10.1021/bi00550a011. [DOI] [PubMed] [Google Scholar]
- Fairclough R. H., Cantor C. R. The use of singlet-singlet energy transfer to study macromolecular assemblies. Methods Enzymol. 1978;48:347–379. doi: 10.1016/s0076-6879(78)48019-x. [DOI] [PubMed] [Google Scholar]
- Gruenwedel D. W., Hsu C. H., Lu D. S. The effects of aqueous neutral-salt solutions on the melting temperatures of deoxyribonucleic acids. Biopolymers. 1971;10(1):47–68. doi: 10.1002/bip.360100106. [DOI] [PubMed] [Google Scholar]
- Gruenwedel D. W. Salt effects on the denaturation of DNA. IV. A calorimetric study of the helix-coil conversion of the alternating copolymer poly[d(A-T)]. Biochim Biophys Acta. 1975 Jul 7;395(3):246–257. doi: 10.1016/0005-2787(75)90195-1. [DOI] [PubMed] [Google Scholar]
- Gryczynski I., Wiczk W., Johnson M. L., Cheung H. C., Wang C. K., Lakowicz J. R. Resolution of end-to-end distance distributions of flexible molecules using quenching-induced variations of the Forster distance for fluorescence energy transfer. Biophys J. 1988 Oct;54(4):577–586. doi: 10.1016/S0006-3495(88)82992-8. [DOI] [PMC free article] [PubMed] [Google Scholar]
- Haas E., Steinberg I. Z. Intramolecular dynamics of chain molecules monitored by fluctuations in efficiency of excitation energy transfer. A theoretical study. Biophys J. 1984 Oct;46(4):429–437. doi: 10.1016/S0006-3495(84)84040-0. [DOI] [PMC free article] [PubMed] [Google Scholar]
- Marky L. A., Breslauer K. J. Calculating thermodynamic data for transitions of any molecularity from equilibrium melting curves. Biopolymers. 1987 Sep;26(9):1601–1620. doi: 10.1002/bip.360260911. [DOI] [PubMed] [Google Scholar]
- Murchie A. I., Clegg R. M., von Kitzing E., Duckett D. R., Diekmann S., Lilley D. M. Fluorescence energy transfer shows that the four-way DNA junction is a right-handed cross of antiparallel molecules. Nature. 1989 Oct 26;341(6244):763–766. doi: 10.1038/341763a0. [DOI] [PubMed] [Google Scholar]
- Stryer L. Fluorescence energy transfer as a spectroscopic ruler. Annu Rev Biochem. 1978;47:819–846. doi: 10.1146/annurev.bi.47.070178.004131. [DOI] [PubMed] [Google Scholar]
- Stryer L. Fluorescence spectroscopy of proteins. Science. 1968 Nov 1;162(3853):526–533. doi: 10.1126/science.162.3853.526. [DOI] [PubMed] [Google Scholar]
- Stryer L., Haugland R. P. Energy transfer: a spectroscopic ruler. Proc Natl Acad Sci U S A. 1967 Aug;58(2):719–726. doi: 10.1073/pnas.58.2.719. [DOI] [PMC free article] [PubMed] [Google Scholar]
- WEBER G. Fluorescence-polarization spectrum and electronic-energy transfer in proteins. Biochem J. 1960 May;75:345–352. doi: 10.1042/bj0750345. [DOI] [PMC free article] [PubMed] [Google Scholar]
- WEBER G. Fluorescence-polarization spectrum and electronic-energy transfer in tyrosine, tryptophan and related compounds. Biochem J. 1960 May;75:335–345. doi: 10.1042/bj0750335. [DOI] [PMC free article] [PubMed] [Google Scholar]
- Weber G., Anderson S. R. The effects of energy transfer and rotational diffusion upon the fluorescence polarization of macromolecules. Biochemistry. 1969 Jan;8(1):361–371. doi: 10.1021/bi00829a050. [DOI] [PubMed] [Google Scholar]
- Yang C. H., Söll D. Studies of transfer RNA tertiary structure of singlet-singlet energy transfer. Proc Natl Acad Sci U S A. 1974 Jul;71(7):2838–2842. doi: 10.1073/pnas.71.7.2838. [DOI] [PMC free article] [PubMed] [Google Scholar]