Abstract
Several self-compatible species of higher plants, such as Arabidopsis thaliana, have recently been found to contain S-like RNases. These S-like RNases are homologous to the S-RNases that have been hypothesized to control self-incompatibility in Solanaceous species. However, the relationship of the S-like RNases to the S-RNases is unknown, and their roles in self-compatible plants are not understood. To address these questions, we have investigated the RNS2 gene, which encodes an S-like RNase (RNS2) of Arabidopsis. Amino acid sequence comparisons indicate that RNS2 and other S-like RNases make up a subclass within an RNase superfamily, which is distinct from the subclass formed by the S-RNases. RNS2 is most similar to RNase LE [Jost, W., Bak, H., Glund, K., Terpstra, P., Beintema, J. J. (1991) Eur. J. Biochem. 198, 1-6.], an S-like RNase from Lycopersicon esculentum, a Solanaceous species. The fact that RNase LE is more similar to RNS2 than to the S-RNases from other Solanaceous plants indicates that the S-like RNases diverged from the S-RNases prior to speciation. Like the S-RNase genes, RNS2 is most highly expressed in flowers, but unlike the S-RNase genes, RNS2 is also expressed in roots, stems, and leaves of Arabidopsis. Moreover, the expression of RNS2 is increased in both leaves and petals of Arabidopsis during senescence. Phosphate starvation can also induce the expression of RNS2. On the basis of these observations, we suggest that one role of RNS2 in Arabidopsis may be to remobilize phosphate, particularly when cells senesce or when phosphate becomes limiting.
Full text
PDF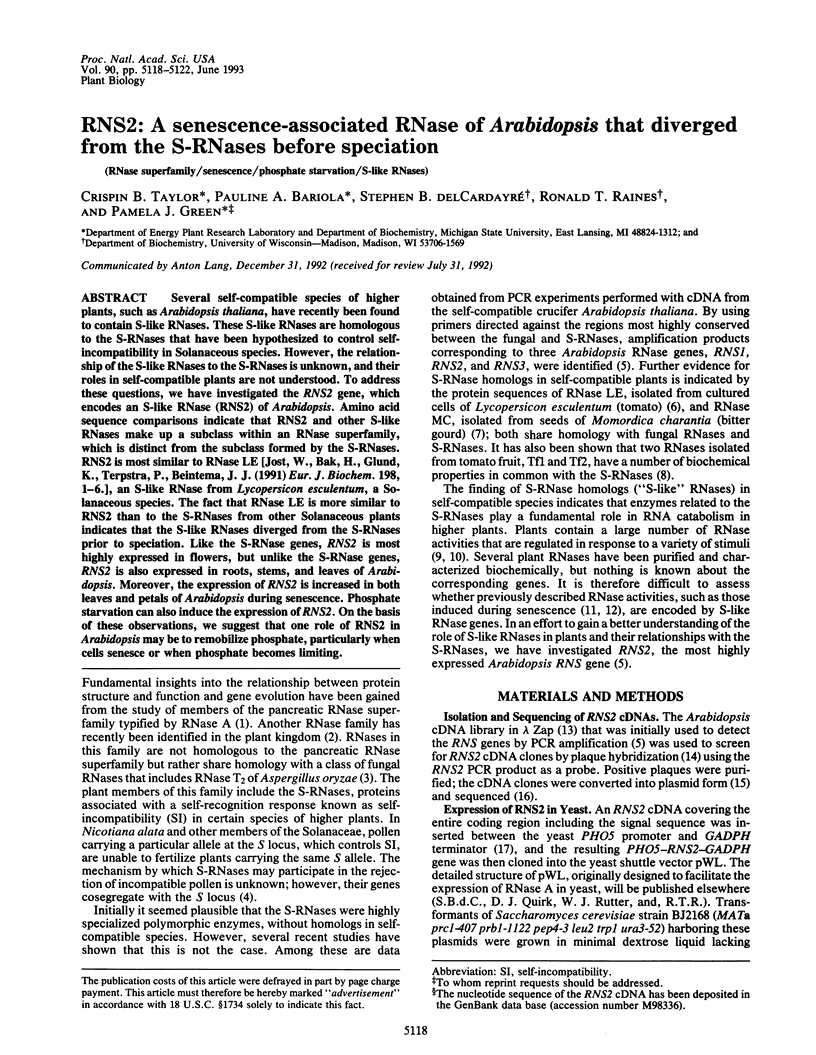
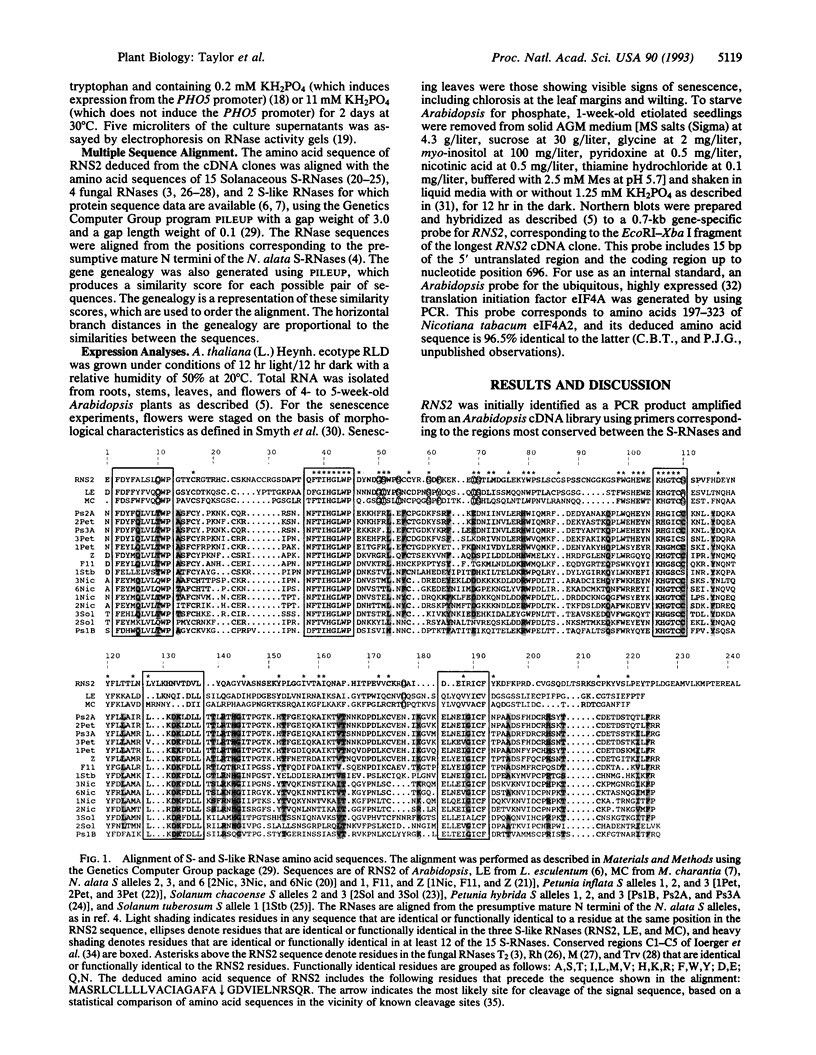
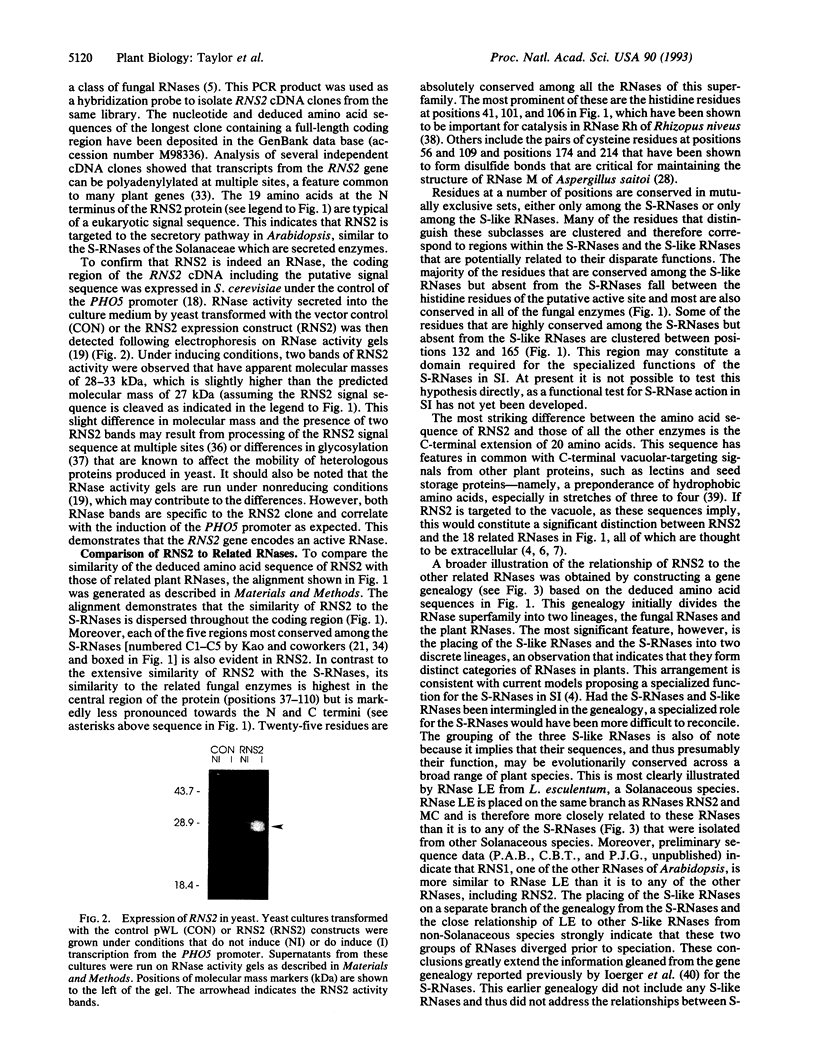
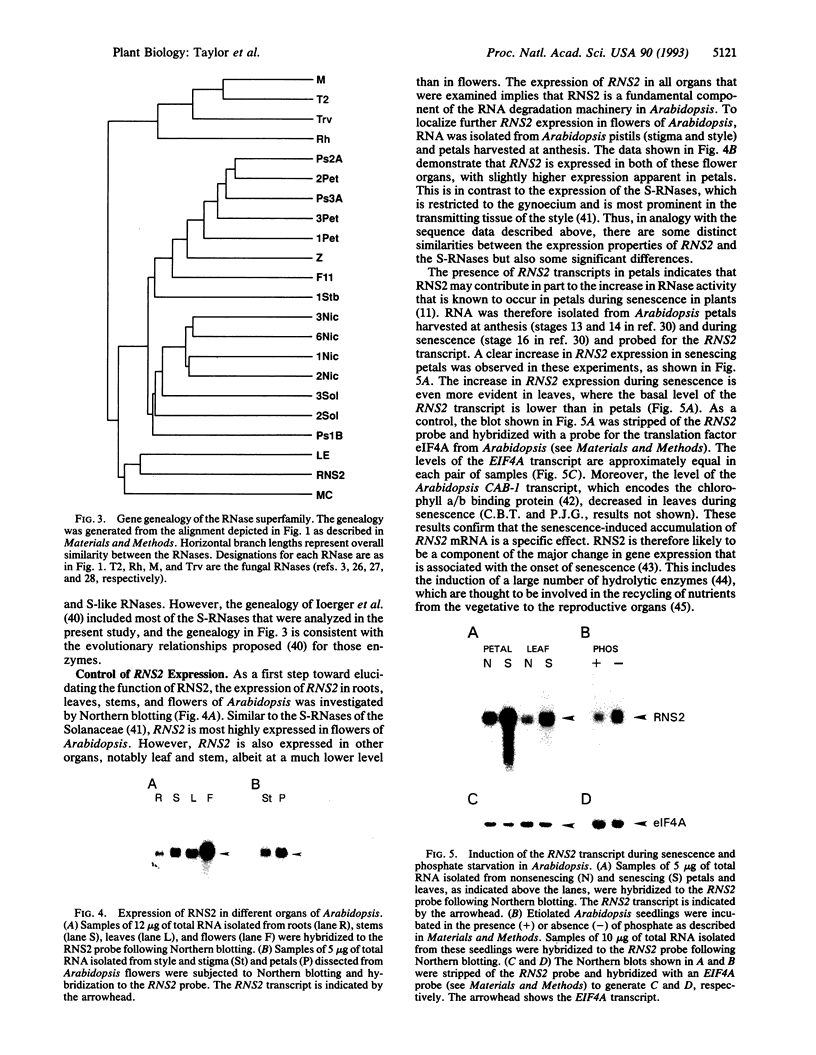
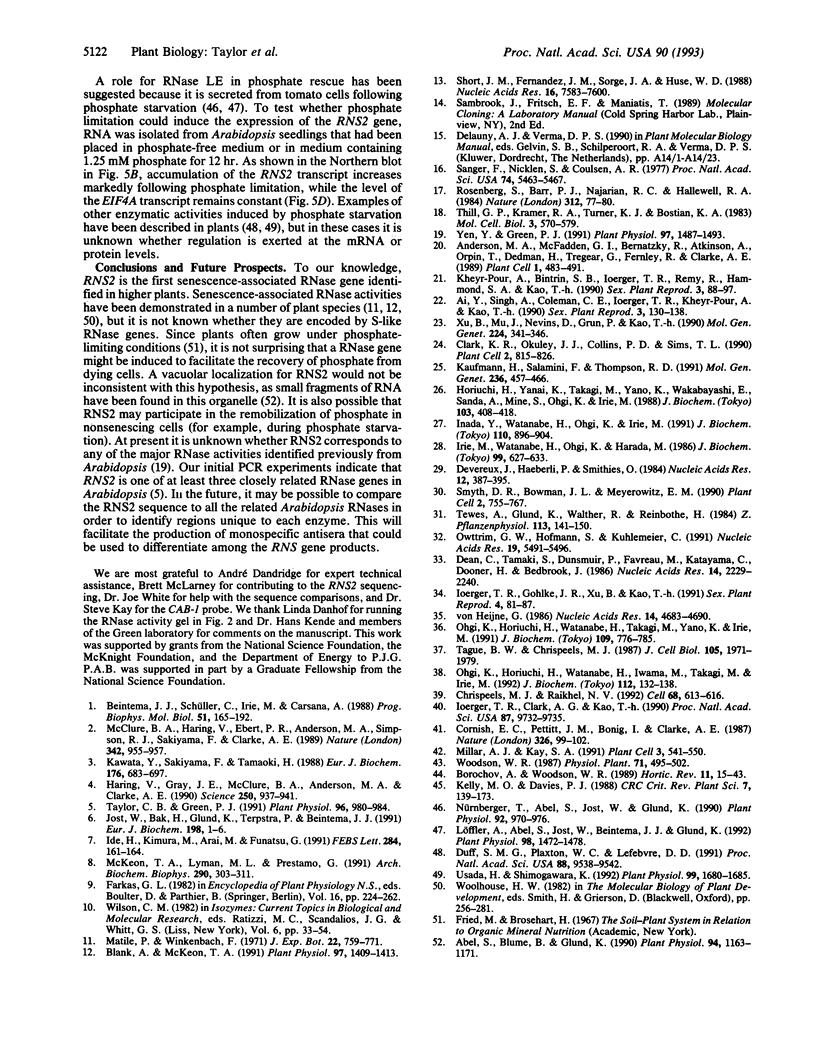
Images in this article
Selected References
These references are in PubMed. This may not be the complete list of references from this article.
- Abel S., Blume B., Glund K. Evidence for RNA-Oligonucleotides in Plant Vacuoles Isolated from Cultured Tomato Cells. Plant Physiol. 1990 Nov;94(3):1163–1171. doi: 10.1104/pp.94.3.1163. [DOI] [PMC free article] [PubMed] [Google Scholar]
- Anderson M. A., McFadden G. I., Bernatzky R., Atkinson A., Orpin T., Dedman H., Tregear G., Fernley R., Clarke A. E. Sequence variability of three alleles of the self-incompatibility gene of Nicotiana alata. Plant Cell. 1989 May;1(5):483–491. doi: 10.1105/tpc.1.5.483. [DOI] [PMC free article] [PubMed] [Google Scholar]
- Beintema J. J., Schüller C., Irie M., Carsana A. Molecular evolution of the ribonuclease superfamily. Prog Biophys Mol Biol. 1988;51(3):165–192. doi: 10.1016/0079-6107(88)90001-6. [DOI] [PubMed] [Google Scholar]
- Blank A., McKeon T. A. Expression of Three RNase Activities during Natural and Dark-Induced Senescence of Wheat Leaves. Plant Physiol. 1991 Dec;97(4):1409–1413. doi: 10.1104/pp.97.4.1409. [DOI] [PMC free article] [PubMed] [Google Scholar]
- Chrispeels M. J., Raikhel N. V. Short peptide domains target proteins to plant vacuoles. Cell. 1992 Feb 21;68(4):613–616. doi: 10.1016/0092-8674(92)90134-x. [DOI] [PubMed] [Google Scholar]
- Clark K. R., Okuley J. J., Collins P. D., Sims T. L. Sequence variability and developmental expression of S-alleles in self-incompatible and pseudo-self-compatible petunia. Plant Cell. 1990 Aug;2(8):815–826. doi: 10.1105/tpc.2.8.815. [DOI] [PMC free article] [PubMed] [Google Scholar]
- Dean C., Tamaki S., Dunsmuir P., Favreau M., Katayama C., Dooner H., Bedbrook J. mRNA transcripts of several plant genes are polyadenylated at multiple sites in vivo. Nucleic Acids Res. 1986 Mar 11;14(5):2229–2240. doi: 10.1093/nar/14.5.2229. [DOI] [PMC free article] [PubMed] [Google Scholar]
- Devereux J., Haeberli P., Smithies O. A comprehensive set of sequence analysis programs for the VAX. Nucleic Acids Res. 1984 Jan 11;12(1 Pt 1):387–395. doi: 10.1093/nar/12.1part1.387. [DOI] [PMC free article] [PubMed] [Google Scholar]
- Duff S. M., Plaxton W. C., Lefebvre D. D. Phosphate-starvation response in plant cells: de novo synthesis and degradation of acid phosphatases. Proc Natl Acad Sci U S A. 1991 Nov 1;88(21):9538–9542. doi: 10.1073/pnas.88.21.9538. [DOI] [PMC free article] [PubMed] [Google Scholar]
- Haring V., Gray J. E., McClure B. A., Anderson M. A., Clarke A. E. Self-incompatibility: a self-recognition system in plants. Science. 1990 Nov 16;250(4983):937–941. doi: 10.1126/science.2237440. [DOI] [PubMed] [Google Scholar]
- Horiuchi H., Yanai K., Takagi M., Yano K., Wakabayashi E., Sanda A., Mine S., Ohgi K., Irie M. Primary structure of a base non-specific ribonuclease from Rhizopus niveus. J Biochem. 1988 Mar;103(3):408–418. doi: 10.1093/oxfordjournals.jbchem.a122284. [DOI] [PubMed] [Google Scholar]
- Ide H., Kimura M., Arai M., Funatsu G. The complete amino acid sequence of ribonuclease from the seeds of bitter gourd (Momordica charantia). FEBS Lett. 1991 Jun 24;284(2):161–164. doi: 10.1016/0014-5793(91)80675-s. [DOI] [PubMed] [Google Scholar]
- Inada Y., Watanabe H., Ohgi K., Irie M. Isolation, characterization, and primary structure of a base non-specific and adenylic acid preferential ribonuclease with higher specific activity from Trichoderma viride. J Biochem. 1991 Dec;110(6):896–904. doi: 10.1093/oxfordjournals.jbchem.a123686. [DOI] [PubMed] [Google Scholar]
- Ioerger T. R., Clark A. G., Kao T. H. Polymorphism at the self-incompatibility locus in Solanaceae predates speciation. Proc Natl Acad Sci U S A. 1990 Dec;87(24):9732–9735. doi: 10.1073/pnas.87.24.9732. [DOI] [PMC free article] [PubMed] [Google Scholar]
- Irie M., Watanabe H., Ohgi K., Harada M. Site of alkylation of the major ribonuclease from Aspergillus saitoi with iodoacetate. J Biochem. 1986 Mar;99(3):627–633. doi: 10.1093/oxfordjournals.jbchem.a135521. [DOI] [PubMed] [Google Scholar]
- Jost W., Bak H., Glund K., Terpstra P., Beintema J. J. Amino acid sequence of an extracellular, phosphate-starvation-induced ribonuclease from cultured tomato (Lycopersicon esculentum) cells. Eur J Biochem. 1991 May 23;198(1):1–6. doi: 10.1111/j.1432-1033.1991.tb15978.x. [DOI] [PubMed] [Google Scholar]
- Kaufmann H., Salamini F., Thompson R. D. Sequence variability and gene structure at the self-incompatibility locus of Solanum tuberosum. Mol Gen Genet. 1991 May;226(3):457–466. doi: 10.1007/BF00260659. [DOI] [PubMed] [Google Scholar]
- Kawata Y., Sakiyama F., Tamaoki H. Amino-acid sequence of ribonuclease T2 from Aspergillus oryzae. Eur J Biochem. 1988 Oct 1;176(3):683–697. doi: 10.1111/j.1432-1033.1988.tb14331.x. [DOI] [PubMed] [Google Scholar]
- Löffler A., Abel S., Jost W., Beintema J. J., Glund K. Phosphate-Regulated Induction of Intracellular Ribonucleases in Cultured Tomato (Lycopersicon esculentum) Cells. Plant Physiol. 1992 Apr;98(4):1472–1478. doi: 10.1104/pp.98.4.1472. [DOI] [PMC free article] [PubMed] [Google Scholar]
- McClure B. A., Haring V., Ebert P. R., Anderson M. A., Simpson R. J., Sakiyama F., Clarke A. E. Style self-incompatibility gene products of Nicotiana alata are ribonucleases. Nature. 1989 Dec 21;342(6252):955–957. doi: 10.1038/342955a0. [DOI] [PubMed] [Google Scholar]
- McKeon T. A., Lyman M. L., Prestamo G. Purification and characterization of two ribonucleases from developing tomato fruit. Arch Biochem Biophys. 1991 Nov 1;290(2):303–311. doi: 10.1016/0003-9861(91)90545-t. [DOI] [PubMed] [Google Scholar]
- Millar A. J., Kay S. A. Circadian Control of cab Gene Transcription and mRNA Accumulation in Arabidopsis. Plant Cell. 1991 May;3(5):541–550. doi: 10.1105/tpc.3.5.541. [DOI] [PMC free article] [PubMed] [Google Scholar]
- Nürnberger T., Abel S., Jost W., Glund K. Induction of an Extracellular Ribonuclease in Cultured Tomato Cells upon Phosphate Starvation. Plant Physiol. 1990 Apr;92(4):970–976. doi: 10.1104/pp.92.4.970. [DOI] [PMC free article] [PubMed] [Google Scholar]
- Ohgi K., Horiuchi H., Watanabe H., Iwama M., Takagi M., Irie M. Evidence that three histidine residues of a base non-specific and adenylic acid preferential ribonuclease from Rhizopus niveus are involved in the catalytic function. J Biochem. 1992 Jul;112(1):132–138. doi: 10.1093/oxfordjournals.jbchem.a123852. [DOI] [PubMed] [Google Scholar]
- Ohgi K., Horiuchi H., Watanabe H., Takagi M., Yano K., Irie M. Expression of RNase Rh from Rhizopus niveus in yeast and characterization of the secreted proteins. J Biochem. 1991 May;109(5):776–785. doi: 10.1093/oxfordjournals.jbchem.a123456. [DOI] [PubMed] [Google Scholar]
- Owttrim G. W., Hofmann S., Kuhlemeier C. Divergent genes for translation initiation factor eIF-4A are coordinately expressed in tobacco. Nucleic Acids Res. 1991 Oct 25;19(20):5491–5496. doi: 10.1093/nar/19.20.5491. [DOI] [PMC free article] [PubMed] [Google Scholar]
- Rosenberg S., Barr P. J., Najarian R. C., Hallewell R. A. Synthesis in yeast of a functional oxidation-resistant mutant of human alpha-antitrypsin. Nature. 1984 Nov 1;312(5989):77–80. doi: 10.1038/312077a0. [DOI] [PubMed] [Google Scholar]
- Sanger F., Nicklen S., Coulson A. R. DNA sequencing with chain-terminating inhibitors. Proc Natl Acad Sci U S A. 1977 Dec;74(12):5463–5467. doi: 10.1073/pnas.74.12.5463. [DOI] [PMC free article] [PubMed] [Google Scholar]
- Short J. M., Fernandez J. M., Sorge J. A., Huse W. D. Lambda ZAP: a bacteriophage lambda expression vector with in vivo excision properties. Nucleic Acids Res. 1988 Aug 11;16(15):7583–7600. doi: 10.1093/nar/16.15.7583. [DOI] [PMC free article] [PubMed] [Google Scholar]
- Smyth D. R., Bowman J. L., Meyerowitz E. M. Early flower development in Arabidopsis. Plant Cell. 1990 Aug;2(8):755–767. doi: 10.1105/tpc.2.8.755. [DOI] [PMC free article] [PubMed] [Google Scholar]
- Tague B. W., Chrispeels M. J. The plant vacuolar protein, phytohemagglutinin, is transported to the vacuole of transgenic yeast. J Cell Biol. 1987 Nov;105(5):1971–1979. doi: 10.1083/jcb.105.5.1971. [DOI] [PMC free article] [PubMed] [Google Scholar]
- Taylor C. B., Green P. J. Genes with Homology to Fungal and S-Gene RNases Are Expressed in Arabidopsis thaliana. Plant Physiol. 1991 Jul;96(3):980–984. doi: 10.1104/pp.96.3.980. [DOI] [PMC free article] [PubMed] [Google Scholar]
- Thill G. P., Kramer R. A., Turner K. J., Bostian K. A. Comparative analysis of the 5'-end regions of two repressible acid phosphatase genes in Saccharomyces cerevisiae. Mol Cell Biol. 1983 Apr;3(4):570–579. doi: 10.1128/mcb.3.4.570. [DOI] [PMC free article] [PubMed] [Google Scholar]
- Usuda H., Shimogawara K. Phosphate Deficiency in Maize : III. Changes in Enzyme Activities during the Course of Phosphate Deprivation. Plant Physiol. 1992 Aug;99(4):1680–1685. doi: 10.1104/pp.99.4.1680. [DOI] [PMC free article] [PubMed] [Google Scholar]
- Xu B. B., Mu J. H., Nevins D. L., Grun P., Kao T. H. Cloning and sequencing of cDNAs encoding two self-incompatibility associated proteins in Solanum chacoense. Mol Gen Genet. 1990 Dec;224(3):341–346. doi: 10.1007/BF00262427. [DOI] [PubMed] [Google Scholar]
- Yen Y., Green P. J. Identification and Properties of the Major Ribonucleases of Arabidopsis thaliana. Plant Physiol. 1991 Dec;97(4):1487–1493. doi: 10.1104/pp.97.4.1487. [DOI] [PMC free article] [PubMed] [Google Scholar]
- von Heijne G. A new method for predicting signal sequence cleavage sites. Nucleic Acids Res. 1986 Jun 11;14(11):4683–4690. doi: 10.1093/nar/14.11.4683. [DOI] [PMC free article] [PubMed] [Google Scholar]