Abstract
Quantitative estimates of the Gibbs free-energy change (delta G) for refolding of one beta-turn conformation into another would assist rational protein design. For beta-turn models, we studied a chirally representative set of nine peptides of the form CH3CO-L1-L2-NHCH3, where loop residues L1 (i + 1) and L2 (i + 2) are achiral Gly (G), L-Ala (A), or D-Ala (a). The stabilities of their common (type I) and inverse-common (type I') beta-turn conformers (GGI is the type-I GG conformer, etc.) were estimated by free-energy simulations using explicit water molecules. An alpha-hydrogen atom of a Gly residue at L1 or L2 was replaced by a methyl group by slow growth. The resulting conformers were less stable than GGI and GGI' by about 1-3 kcal/mol (delta G = 0.9 kcal/mol for AGI and aGI', 1.0 kcal/mol for GAI and GaI', 2.1 kcal/mol for aGI and AGI', and 2.8 kcal/mol for GaI and GAI'; 1 kcal = 4.18 kJ). The delta G value for simultaneous growth of one methyl group at L1 and another at L2 was the sum of the two component delta G values. The delta G values for I-->I' refolding of the common beta-turn conformer into the inverse-common beta-turn conformer ranged over 6 kcal/mol (-3.0 for aa, -1.8 for Ga, -1.1 for aG, -0.7 for Aa, 0 for GG, 0.7 for aA, 1.1 for AG, 1.8 for GA, and 3.0 for AA). Thus, replacing L-Ala by D-Ala at both L1 and L2 of a common beta turn may contribute as much as 6 kcal/mol toward its refolding as an inverse-common beta turn.
Full text
PDF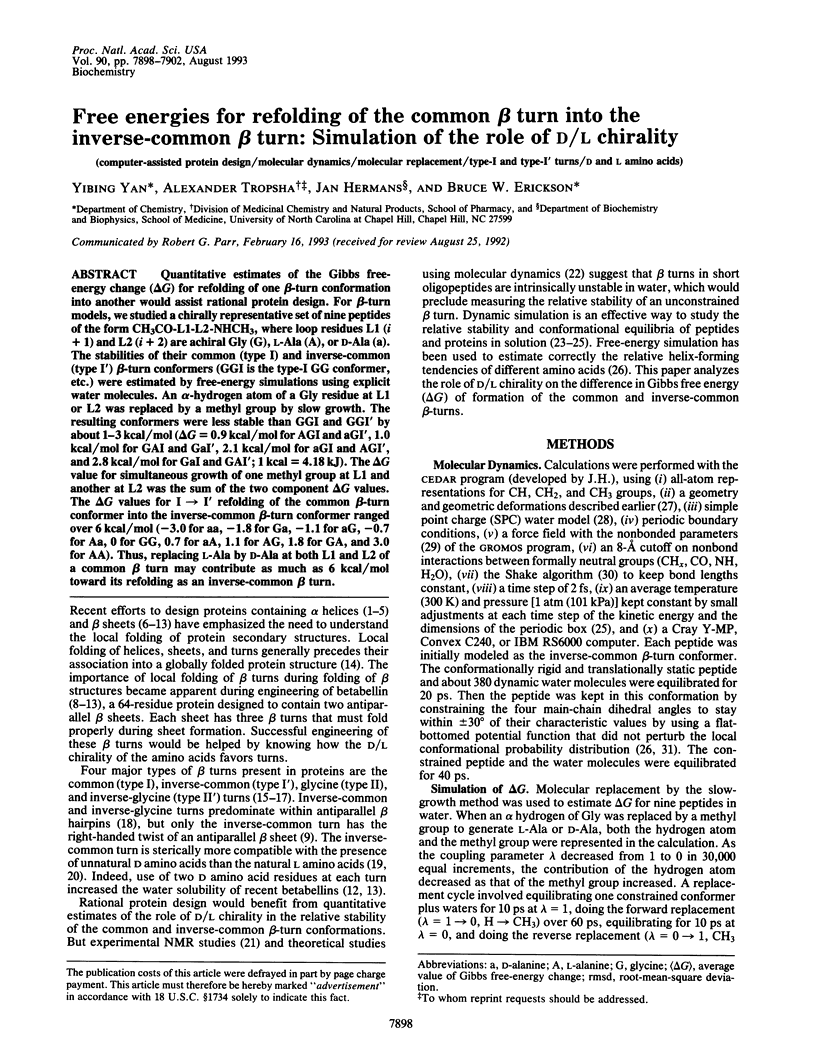
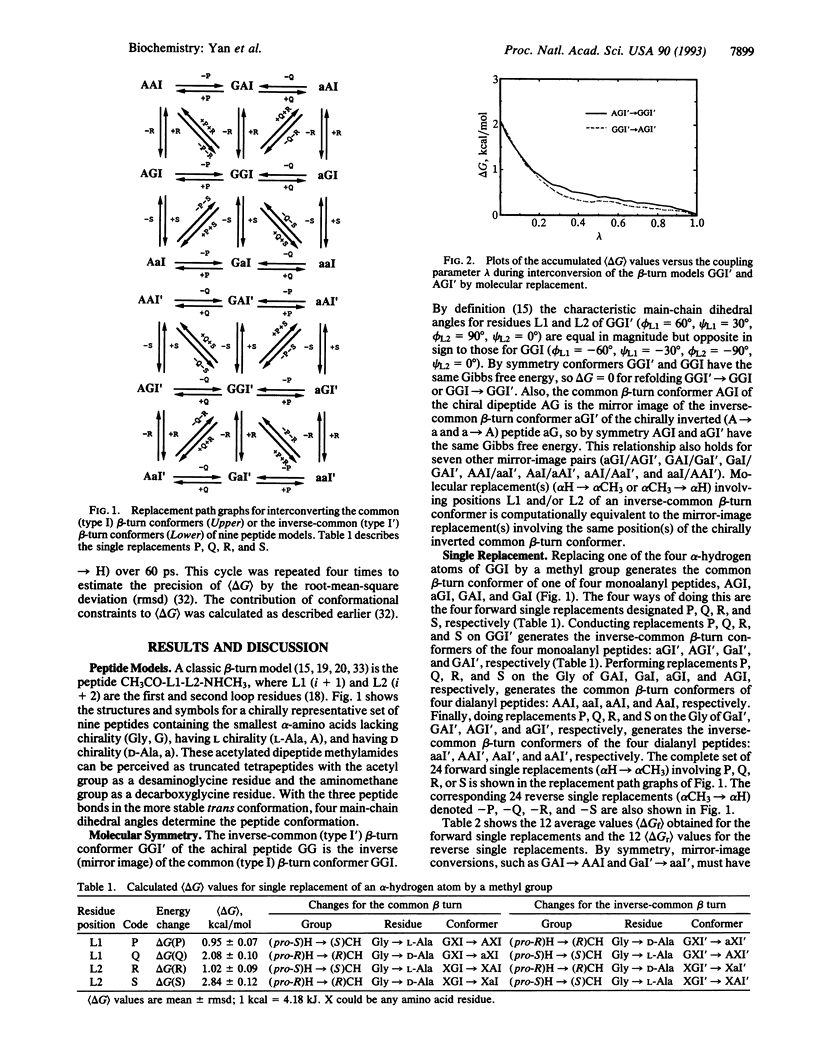
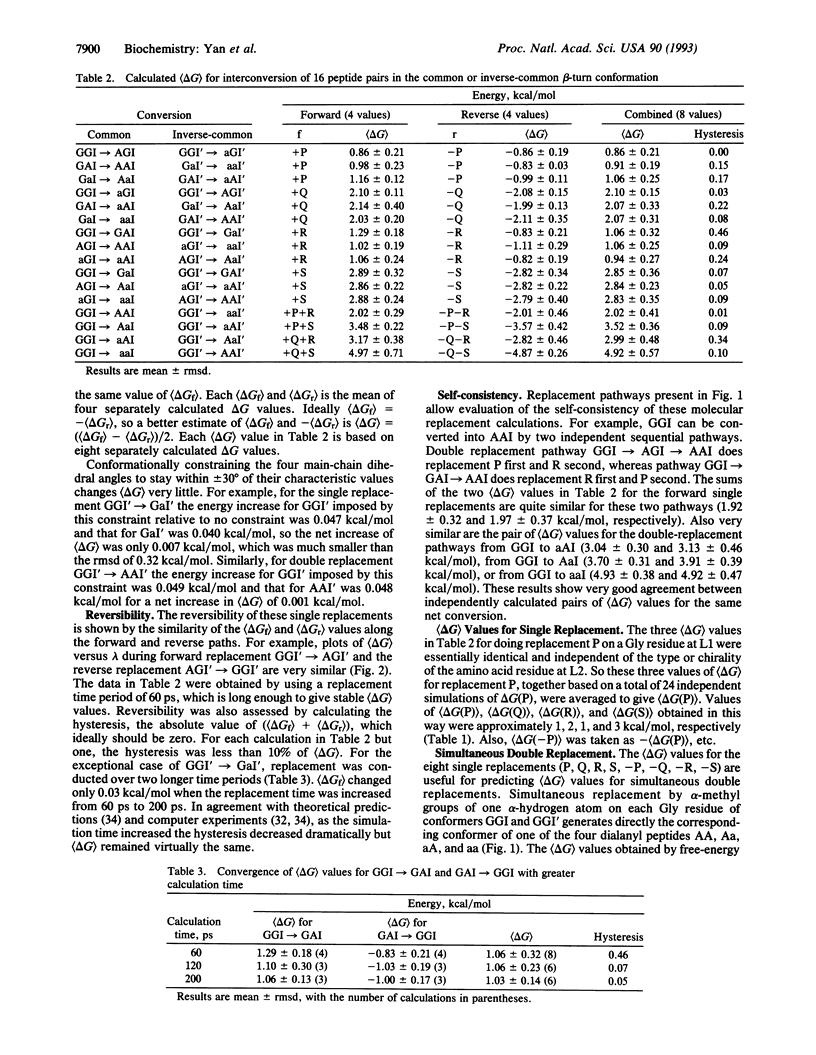
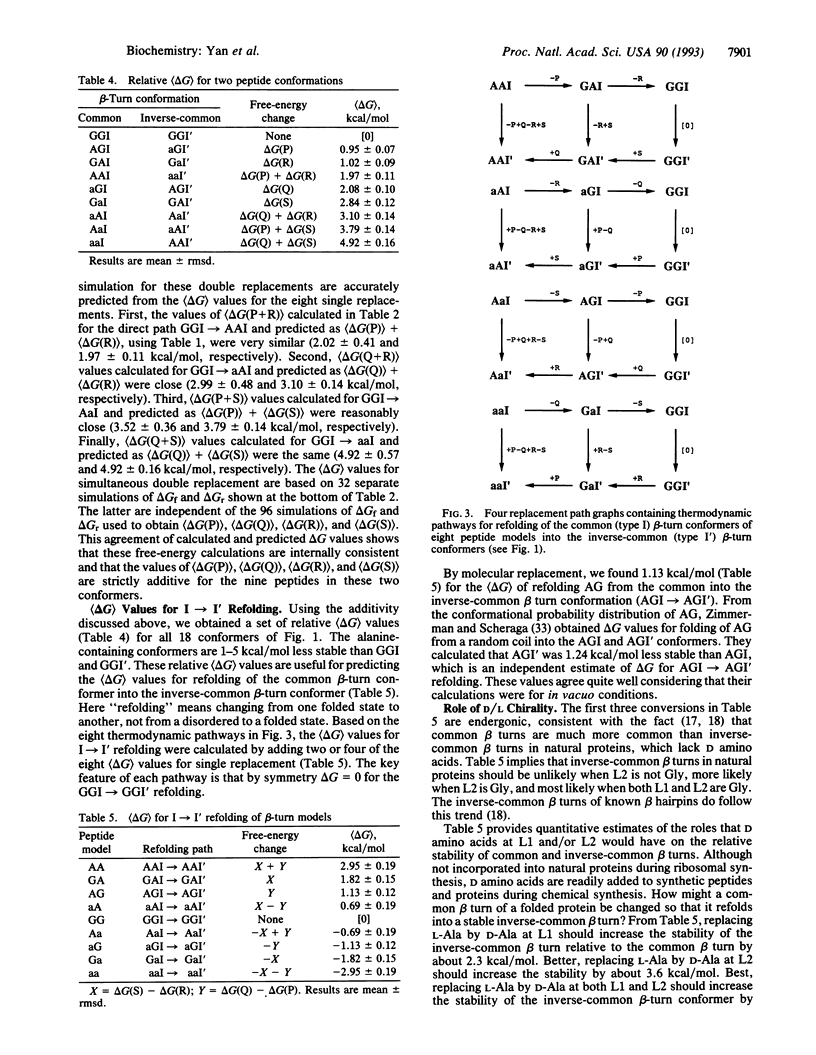
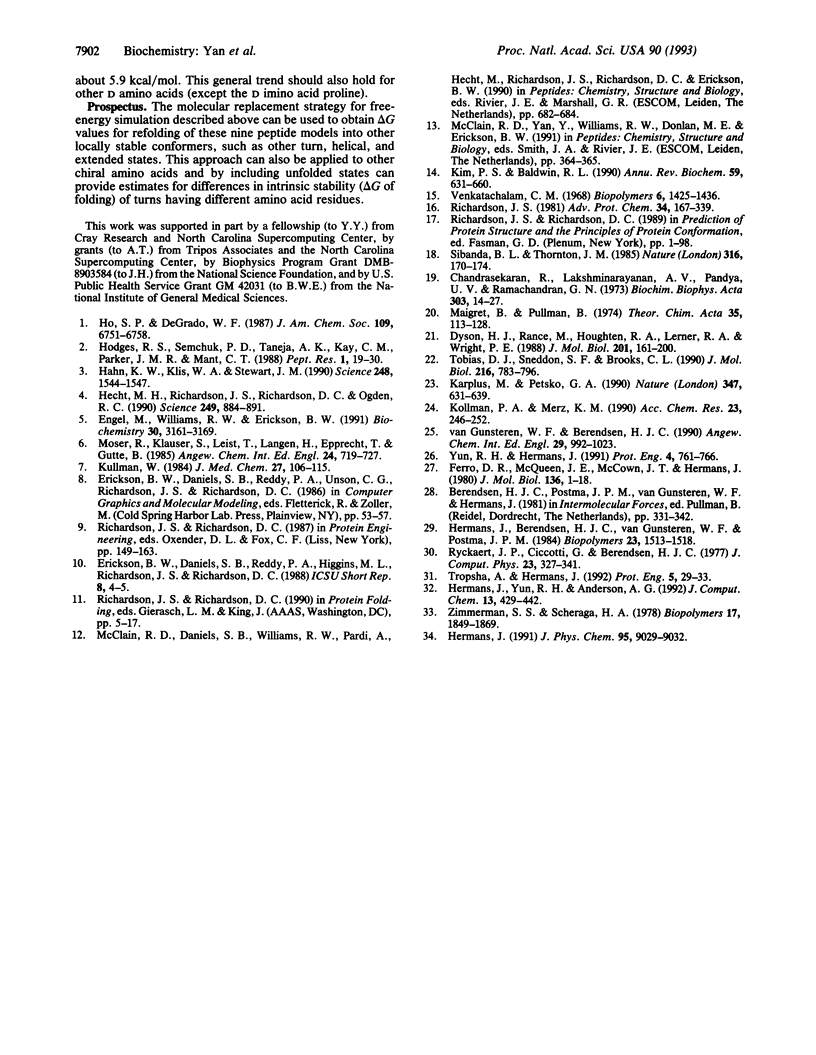
Selected References
These references are in PubMed. This may not be the complete list of references from this article.
- Chandrasekaran R., Lakshminarayanan A. V., Pandya U. V., Ramachandran G. N. Conformation of the LL and LD hairpin bends with internal hydrogen bonds in proteins and peptides. Biochim Biophys Acta. 1973 Mar 23;303(1):14–27. doi: 10.1016/0005-2795(73)90143-8. [DOI] [PubMed] [Google Scholar]
- Dyson H. J., Rance M., Houghten R. A., Lerner R. A., Wright P. E. Folding of immunogenic peptide fragments of proteins in water solution. I. Sequence requirements for the formation of a reverse turn. J Mol Biol. 1988 May 5;201(1):161–200. doi: 10.1016/0022-2836(88)90446-9. [DOI] [PubMed] [Google Scholar]
- Engel M., Williams R. W., Erickson B. W. Designed coiled-coil proteins: synthesis and spectroscopy of two 78-residue alpha-helical dimers. Biochemistry. 1991 Apr 2;30(13):3161–3169. doi: 10.1021/bi00227a002. [DOI] [PubMed] [Google Scholar]
- Ferro D. R., McQueen J. E., Jr, McCown J. T., Hermans J. Energy minimizations of rubredoxin. J Mol Biol. 1980 Jan 5;136(1):1–18. doi: 10.1016/0022-2836(80)90363-0. [DOI] [PubMed] [Google Scholar]
- Hahn K. W., Klis W. A., Stewart J. M. Design and synthesis of a peptide having chymotrypsin-like esterase activity. Science. 1990 Jun 22;248(4962):1544–1547. doi: 10.1126/science.2360048. [DOI] [PubMed] [Google Scholar]
- Hecht M. H., Richardson J. S., Richardson D. C., Ogden R. C. De novo design, expression, and characterization of Felix: a four-helix bundle protein of native-like sequence. Science. 1990 Aug 24;249(4971):884–891. doi: 10.1126/science.2392678. [DOI] [PubMed] [Google Scholar]
- Hodges R. S., Semchuk P. D., Taneja A. K., Kay C. M., Parker J. M., Mant C. T. Protein design using model synthetic peptides. Pept Res. 1988 Sep-Oct;1(1):19–30. [PubMed] [Google Scholar]
- Karplus M., Petsko G. A. Molecular dynamics simulations in biology. Nature. 1990 Oct 18;347(6294):631–639. doi: 10.1038/347631a0. [DOI] [PubMed] [Google Scholar]
- Kim P. S., Baldwin R. L. Intermediates in the folding reactions of small proteins. Annu Rev Biochem. 1990;59:631–660. doi: 10.1146/annurev.bi.59.070190.003215. [DOI] [PubMed] [Google Scholar]
- Kullmann W. Design, synthesis, and binding characteristics of an opiate receptor mimetic peptide. J Med Chem. 1984 Feb;27(2):106–115. doi: 10.1021/jm00368a002. [DOI] [PubMed] [Google Scholar]
- Richardson J. S. The anatomy and taxonomy of protein structure. Adv Protein Chem. 1981;34:167–339. doi: 10.1016/s0065-3233(08)60520-3. [DOI] [PubMed] [Google Scholar]
- Sibanda B. L., Thornton J. M. Beta-hairpin families in globular proteins. Nature. 1985 Jul 11;316(6024):170–174. doi: 10.1038/316170a0. [DOI] [PubMed] [Google Scholar]
- Tobias D. J., Sneddon S. F., Brooks C. L., 3rd Reverse turns in blocked dipeptides are intrinsically unstable in water. J Mol Biol. 1990 Dec 5;216(3):783–796. doi: 10.1016/0022-2836(90)90399-7. [DOI] [PubMed] [Google Scholar]
- Tropsha A., Hermans J. Application of free energy simulations to the binding of a transition-state-analogue inhibitor to HIV protease. Protein Eng. 1992 Jan;5(1):29–33. doi: 10.1093/protein/5.1.29. [DOI] [PubMed] [Google Scholar]
- Venkatachalam C. M. Stereochemical criteria for polypeptides and proteins. V. Conformation of a system of three linked peptide units. Biopolymers. 1968 Oct;6(10):1425–1436. doi: 10.1002/bip.1968.360061006. [DOI] [PubMed] [Google Scholar]
- Yun R. H., Hermans J. Conformational equilibria of valine studied by dynamics simulation. Protein Eng. 1991 Oct;4(7):761–766. doi: 10.1093/protein/4.7.761. [DOI] [PubMed] [Google Scholar]