Abstract
Openings of single L-type Ca2+ channels following repolarization to negative membrane potentials from a depolarizing step (repolarization openings, ROs) have been described previously in brain cell preparations. However, these ROs have been reported to occur only infrequently. Here we report that the frequency of ROs in cell-attached patches of cultured rat hippocampal neurons can be increased dramatically by lowering the pipette Ba2+ concentration to 20 mM from the usual 90-110 mM. This increased opening probability can last for hundreds to thousands of milliseconds following repolarization. Current-voltage analyses of open probability show that the depolarization pulse threshold for inducing ROs in 20 mM Ba2+ is -10 to 0 mV but that the probability of ROs reaches maximal levels following depolarizing pulses that approach the apparent null (equilibrium) potential for Ba2+. Comparable current-voltage curves in 110 mM Ba2+ from a more positive holding potential (-50 mV) indicate that membrane surface charge screening accounts for some, but not all, of the effect of lowering the Ba2+ concentration. Consequently, current-dependent inactivation or some other ion-dependent mechanism (e.g., ion binding inside the pore) also appears to regulate this potentially major pathway of Ca2+ entry. A high probability of ROs also can be induced under relatively physiological conditions (5-ms depolarizing steps, 2-5 mM Ca2+ in the pipette). Thus, the high open probability state at negative potentials may underlie the long Ca2+ tail currents in hippocampus that were described previously and appears to have major implications for physiological functions (e.g., the slow Ca(2+)-dependent afterhyperpolarization), particularly in brain neurons.
Full text
PDF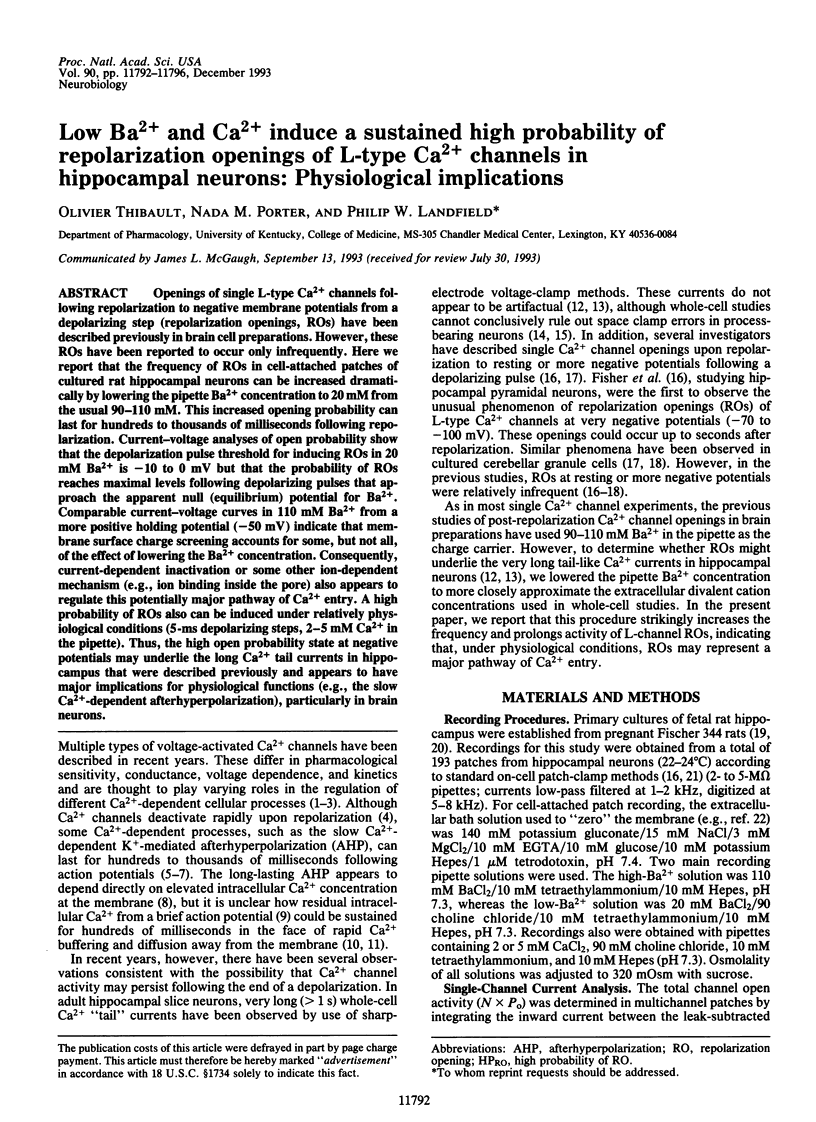
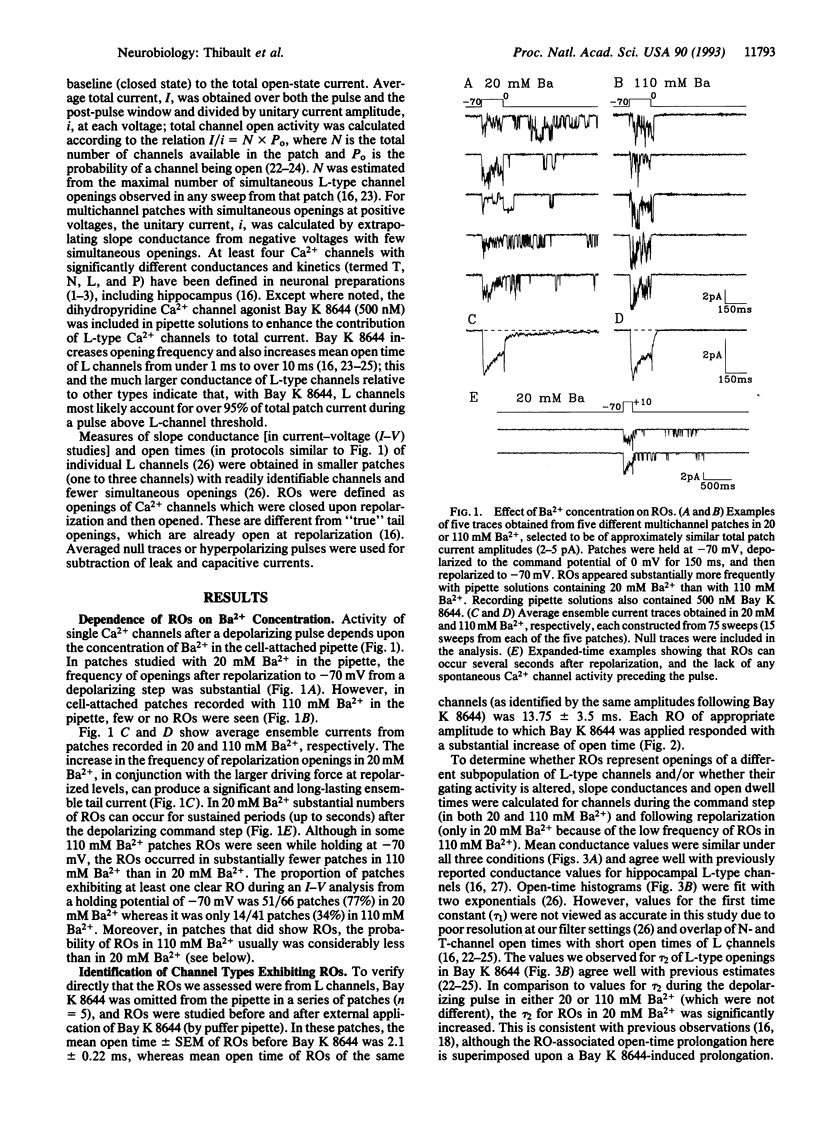
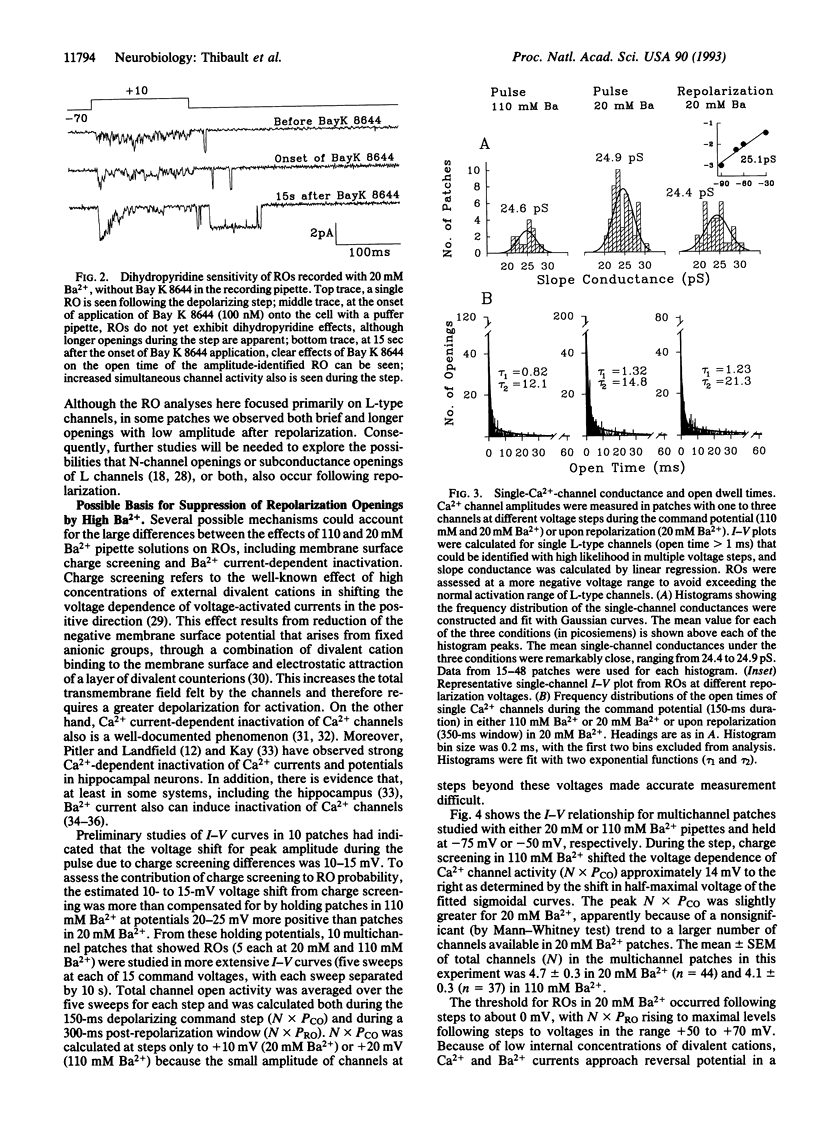
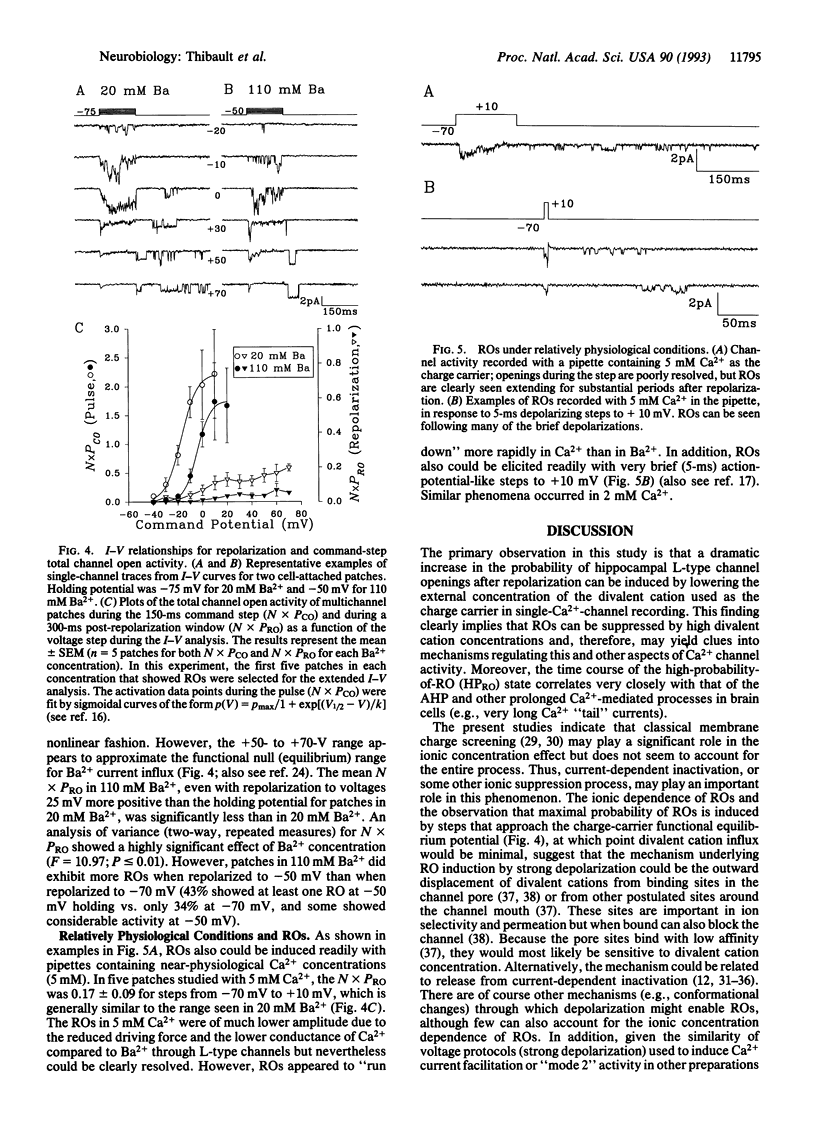
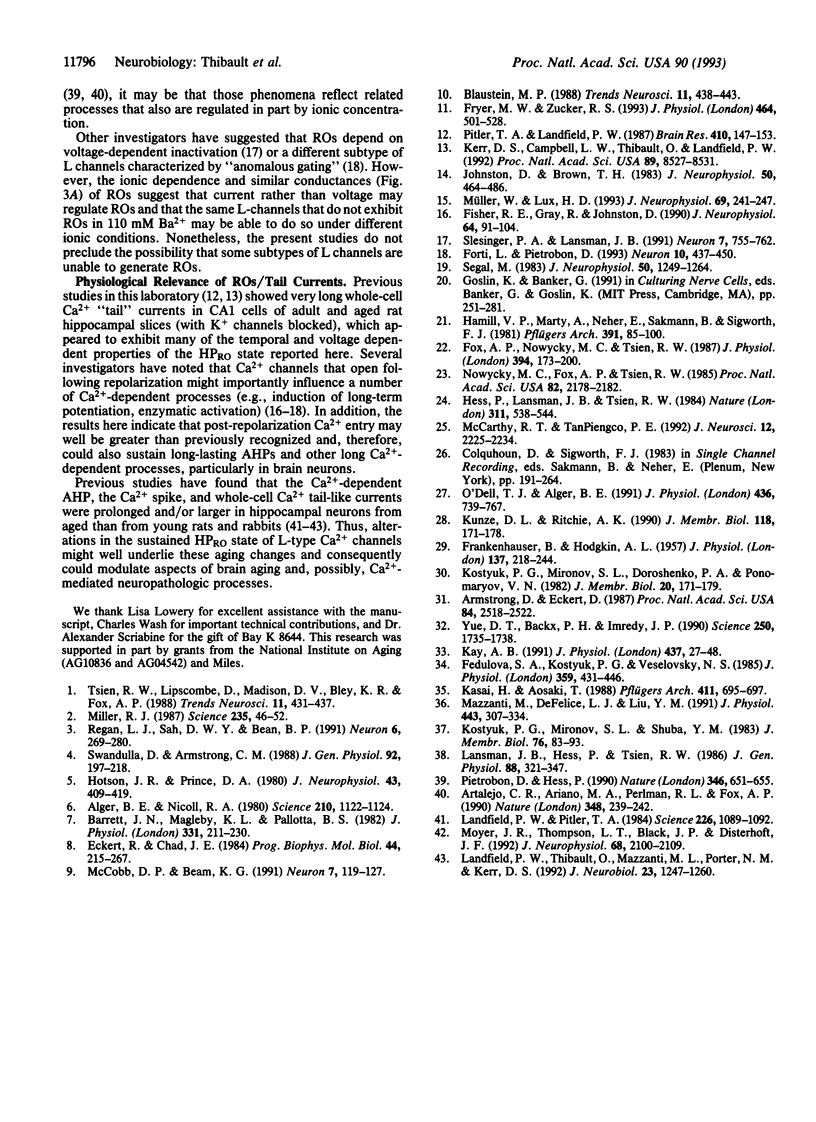
Selected References
These references are in PubMed. This may not be the complete list of references from this article.
- Alger B. E., Nicoll R. A. Epileptiform burst afterhyperolarization: calcium-dependent potassium potential in hippocampal CA1 pyramidal cells. Science. 1980 Dec 5;210(4474):1122–1124. doi: 10.1126/science.7444438. [DOI] [PubMed] [Google Scholar]
- Armstrong D., Eckert R. Voltage-activated calcium channels that must be phosphorylated to respond to membrane depolarization. Proc Natl Acad Sci U S A. 1987 Apr;84(8):2518–2522. doi: 10.1073/pnas.84.8.2518. [DOI] [PMC free article] [PubMed] [Google Scholar]
- Artalejo C. R., Ariano M. A., Perlman R. L., Fox A. P. Activation of facilitation calcium channels in chromaffin cells by D1 dopamine receptors through a cAMP/protein kinase A-dependent mechanism. Nature. 1990 Nov 15;348(6298):239–242. doi: 10.1038/348239a0. [DOI] [PubMed] [Google Scholar]
- Barrett J. N., Magleby K. L., Pallotta B. S. Properties of single calcium-activated potassium channels in cultured rat muscle. J Physiol. 1982 Oct;331:211–230. doi: 10.1113/jphysiol.1982.sp014370. [DOI] [PMC free article] [PubMed] [Google Scholar]
- Blaustein M. P. Calcium transport and buffering in neurons. Trends Neurosci. 1988 Oct;11(10):438–443. doi: 10.1016/0166-2236(88)90195-6. [DOI] [PubMed] [Google Scholar]
- Eckert R., Chad J. E. Inactivation of Ca channels. Prog Biophys Mol Biol. 1984;44(3):215–267. doi: 10.1016/0079-6107(84)90009-9. [DOI] [PubMed] [Google Scholar]
- FRANKENHAEUSER B., HODGKIN A. L. The action of calcium on the electrical properties of squid axons. J Physiol. 1957 Jul 11;137(2):218–244. doi: 10.1113/jphysiol.1957.sp005808. [DOI] [PMC free article] [PubMed] [Google Scholar]
- Fedulova S. A., Kostyuk P. G., Veselovsky N. S. Two types of calcium channels in the somatic membrane of new-born rat dorsal root ganglion neurones. J Physiol. 1985 Feb;359:431–446. doi: 10.1113/jphysiol.1985.sp015594. [DOI] [PMC free article] [PubMed] [Google Scholar]
- Fisher R. E., Gray R., Johnston D. Properties and distribution of single voltage-gated calcium channels in adult hippocampal neurons. J Neurophysiol. 1990 Jul;64(1):91–104. doi: 10.1152/jn.1990.64.1.91. [DOI] [PubMed] [Google Scholar]
- Forti L., Pietrobon D. Functional diversity of L-type calcium channels in rat cerebellar neurons. Neuron. 1993 Mar;10(3):437–450. doi: 10.1016/0896-6273(93)90332-l. [DOI] [PubMed] [Google Scholar]
- Fox A. P., Nowycky M. C., Tsien R. W. Single-channel recordings of three types of calcium channels in chick sensory neurones. J Physiol. 1987 Dec;394:173–200. doi: 10.1113/jphysiol.1987.sp016865. [DOI] [PMC free article] [PubMed] [Google Scholar]
- Fryer M. W., Zucker R. S. Ca(2+)-dependent inactivation of Ca2+ current in Aplysia neurons: kinetic studies using photolabile Ca2+ chelators. J Physiol. 1993 May;464:501–528. doi: 10.1113/jphysiol.1993.sp019648. [DOI] [PMC free article] [PubMed] [Google Scholar]
- Hamill O. P., Marty A., Neher E., Sakmann B., Sigworth F. J. Improved patch-clamp techniques for high-resolution current recording from cells and cell-free membrane patches. Pflugers Arch. 1981 Aug;391(2):85–100. doi: 10.1007/BF00656997. [DOI] [PubMed] [Google Scholar]
- Hess P., Lansman J. B., Tsien R. W. Different modes of Ca channel gating behaviour favoured by dihydropyridine Ca agonists and antagonists. Nature. 1984 Oct 11;311(5986):538–544. doi: 10.1038/311538a0. [DOI] [PubMed] [Google Scholar]
- Hotson J. R., Prince D. A. A calcium-activated hyperpolarization follows repetitive firing in hippocampal neurons. J Neurophysiol. 1980 Feb;43(2):409–419. doi: 10.1152/jn.1980.43.2.409. [DOI] [PubMed] [Google Scholar]
- Johnston D., Brown T. H. Interpretation of voltage-clamp measurements in hippocampal neurons. J Neurophysiol. 1983 Aug;50(2):464–486. doi: 10.1152/jn.1983.50.2.464. [DOI] [PubMed] [Google Scholar]
- Kasai H., Aosaki T. Divalent cation dependent inactivation of the high-voltage-activated Ca-channel current in chick sensory neurons. Pflugers Arch. 1988 Jun;411(6):695–697. doi: 10.1007/BF00580869. [DOI] [PubMed] [Google Scholar]
- Kay A. R. Inactivation kinetics of calcium current of acutely dissociated CA1 pyramidal cells of the mature guinea-pig hippocampus. J Physiol. 1991 Jun;437:27–48. doi: 10.1113/jphysiol.1991.sp018581. [DOI] [PMC free article] [PubMed] [Google Scholar]
- Kerr D. S., Campbell L. W., Thibault O., Landfield P. W. Hippocampal glucocorticoid receptor activation enhances voltage-dependent Ca2+ conductances: relevance to brain aging. Proc Natl Acad Sci U S A. 1992 Sep 15;89(18):8527–8531. doi: 10.1073/pnas.89.18.8527. [DOI] [PMC free article] [PubMed] [Google Scholar]
- Kunze D. L., Ritchie A. K. Multiple conductance levels of the dihydropyridine-sensitive calcium channel in GH3 cells. J Membr Biol. 1990 Nov;118(2):171–178. doi: 10.1007/BF01868474. [DOI] [PubMed] [Google Scholar]
- Landfield P. W., Pitler T. A. Prolonged Ca2+-dependent afterhyperpolarizations in hippocampal neurons of aged rats. Science. 1984 Nov 30;226(4678):1089–1092. doi: 10.1126/science.6494926. [DOI] [PubMed] [Google Scholar]
- Landfield P. W., Thibault O., Mazzanti M. L., Porter N. M., Kerr D. S. Mechanisms of neuronal death in brain aging and Alzheimer's disease: role of endocrine-mediated calcium dyshomeostasis. J Neurobiol. 1992 Nov;23(9):1247–1260. doi: 10.1002/neu.480230914. [DOI] [PubMed] [Google Scholar]
- Lansman J. B., Hess P., Tsien R. W. Blockade of current through single calcium channels by Cd2+, Mg2+, and Ca2+. Voltage and concentration dependence of calcium entry into the pore. J Gen Physiol. 1986 Sep;88(3):321–347. doi: 10.1085/jgp.88.3.321. [DOI] [PMC free article] [PubMed] [Google Scholar]
- Mazzanti M., DeFelice L. J., Liu Y. M. Gating of L-type Ca2+ channels in embryonic chick ventricle cells: dependence on voltage, current and channel density. J Physiol. 1991 Nov;443:307–334. doi: 10.1113/jphysiol.1991.sp018835. [DOI] [PMC free article] [PubMed] [Google Scholar]
- McCarthy R. T., TanPiengco P. E. Multiple types of high-threshold calcium channels in rabbit sensory neurons: high-affinity block of neuronal L-type by nimodipine. J Neurosci. 1992 Jun;12(6):2225–2234. doi: 10.1523/JNEUROSCI.12-06-02225.1992. [DOI] [PMC free article] [PubMed] [Google Scholar]
- McCobb D. P., Beam K. G. Action potential waveform voltage-clamp commands reveal striking differences in calcium entry via low and high voltage-activated calcium channels. Neuron. 1991 Jul;7(1):119–127. doi: 10.1016/0896-6273(91)90080-j. [DOI] [PubMed] [Google Scholar]
- Miller R. J. Multiple calcium channels and neuronal function. Science. 1987 Jan 2;235(4784):46–52. doi: 10.1126/science.2432656. [DOI] [PubMed] [Google Scholar]
- Moyer J. R., Jr, Thompson L. T., Black J. P., Disterhoft J. F. Nimodipine increases excitability of rabbit CA1 pyramidal neurons in an age- and concentration-dependent manner. J Neurophysiol. 1992 Dec;68(6):2100–2109. doi: 10.1152/jn.1992.68.6.2100. [DOI] [PubMed] [Google Scholar]
- Müller W., Lux H. D. Analysis of voltage-dependent membrane currents in spatially extended neurons from point-clamp data. J Neurophysiol. 1993 Jan;69(1):241–247. doi: 10.1152/jn.1993.69.1.241. [DOI] [PubMed] [Google Scholar]
- Nowycky M. C., Fox A. P., Tsien R. W. Long-opening mode of gating of neuronal calcium channels and its promotion by the dihydropyridine calcium agonist Bay K 8644. Proc Natl Acad Sci U S A. 1985 Apr;82(7):2178–2182. doi: 10.1073/pnas.82.7.2178. [DOI] [PMC free article] [PubMed] [Google Scholar]
- O'Dell T. J., Alger B. E. Single calcium channels in rat and guinea-pig hippocampal neurons. J Physiol. 1991 May;436:739–767. doi: 10.1113/jphysiol.1991.sp018577. [DOI] [PMC free article] [PubMed] [Google Scholar]
- Pietrobon D., Hess P. Novel mechanism of voltage-dependent gating in L-type calcium channels. Nature. 1990 Aug 16;346(6285):651–655. doi: 10.1038/346651a0. [DOI] [PubMed] [Google Scholar]
- Pitler T. A., Landfield P. W. Probable Ca2+-mediated inactivation of Ca2+ currents in mammalian brain neurons. Brain Res. 1987 Apr 28;410(1):147–153. doi: 10.1016/s0006-8993(87)80037-9. [DOI] [PubMed] [Google Scholar]
- Regan L. J., Sah D. W., Bean B. P. Ca2+ channels in rat central and peripheral neurons: high-threshold current resistant to dihydropyridine blockers and omega-conotoxin. Neuron. 1991 Feb;6(2):269–280. doi: 10.1016/0896-6273(91)90362-4. [DOI] [PubMed] [Google Scholar]
- Segal M. Rat hippocampal neurons in culture: responses to electrical and chemical stimuli. J Neurophysiol. 1983 Dec;50(6):1249–1264. doi: 10.1152/jn.1983.50.6.1249. [DOI] [PubMed] [Google Scholar]
- Slesinger P. A., Lansman J. B. Reopening of Ca2+ channels in mouse cerebellar neurons at resting membrane potentials during recovery from inactivation. Neuron. 1991 Nov;7(5):755–762. doi: 10.1016/0896-6273(91)90278-8. [DOI] [PubMed] [Google Scholar]
- Swandulla D., Armstrong C. M. Fast-deactivating calcium channels in chick sensory neurons. J Gen Physiol. 1988 Aug;92(2):197–218. doi: 10.1085/jgp.92.2.197. [DOI] [PMC free article] [PubMed] [Google Scholar]
- Tsien R. W., Lipscombe D., Madison D. V., Bley K. R., Fox A. P. Multiple types of neuronal calcium channels and their selective modulation. Trends Neurosci. 1988 Oct;11(10):431–438. doi: 10.1016/0166-2236(88)90194-4. [DOI] [PubMed] [Google Scholar]
- Yue D. T., Backx P. H., Imredy J. P. Calcium-sensitive inactivation in the gating of single calcium channels. Science. 1990 Dec 21;250(4988):1735–1738. doi: 10.1126/science.2176745. [DOI] [PubMed] [Google Scholar]