Abstract
We propose that the key structural feature in the conversion of chemical free energy into mechanical work by actomyosin is a myosin-induced change in the length of the actin filament. As reported earlier, there is evidence that helical actin filaments can untwist into ribbons having an increased intersubunit repeat. Regular patterns of actomyosin interactions arise when ribbons are aligned with myosin thick filaments, because the repeat distance of the myosin lattice (429 A) is an integral multiple of the subunit repeat in the ribbon (35.7 A). This commensurability property of the actomyosin lattice leads to a simple mechanism for controlling the sequence of events in chemical-mechanical transduction. A role for tropomyosin in transmitting the forces developed by actomyosin is proposed. In this paper, we describe how these transduction principles provide the basis for a theory of muscle contraction.
Full text
PDF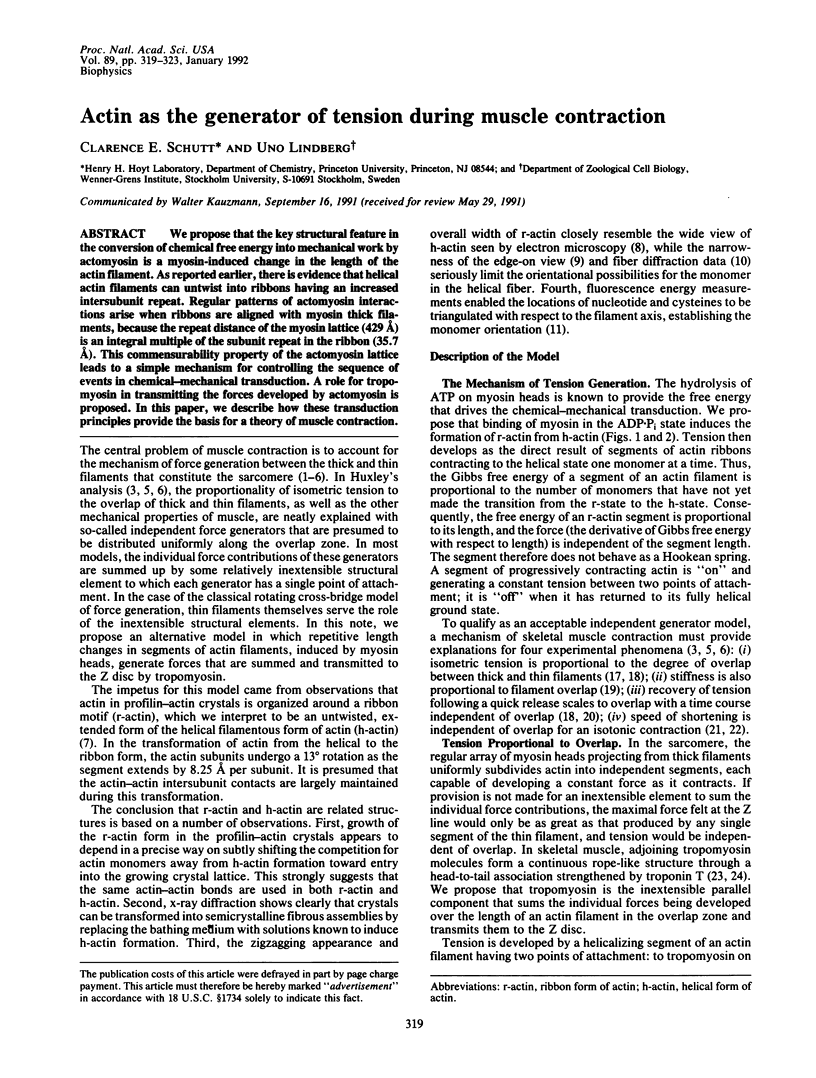
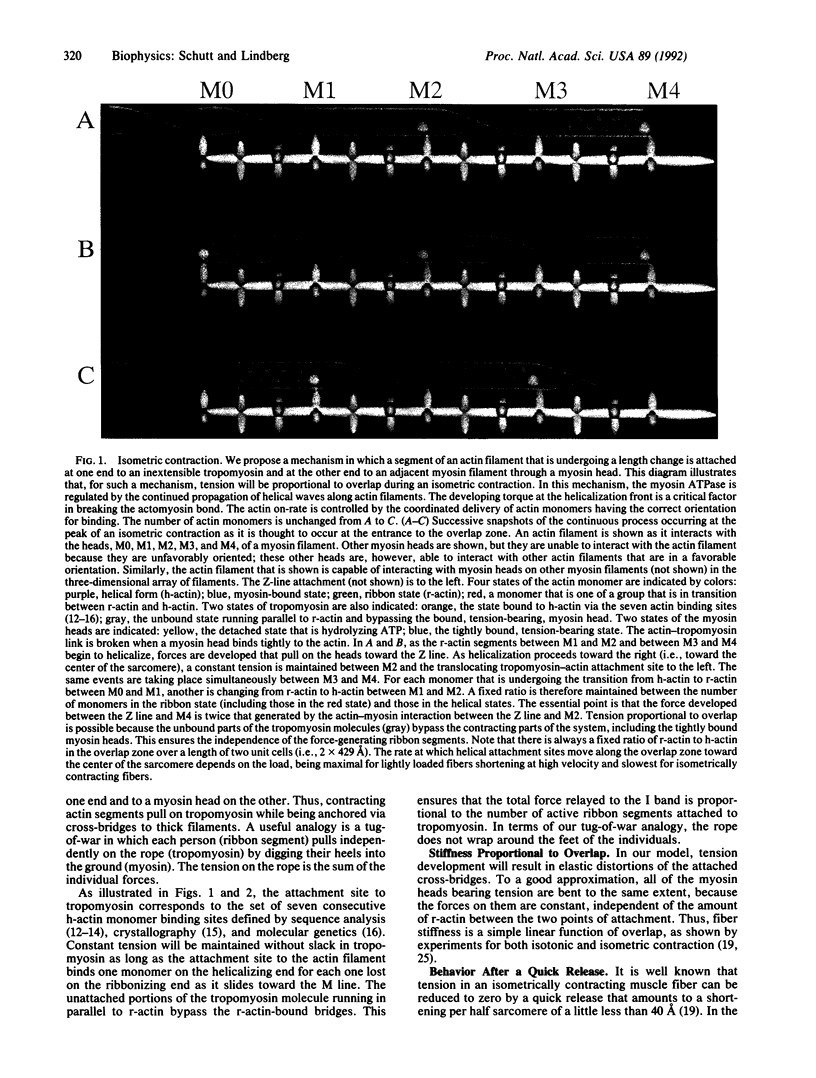
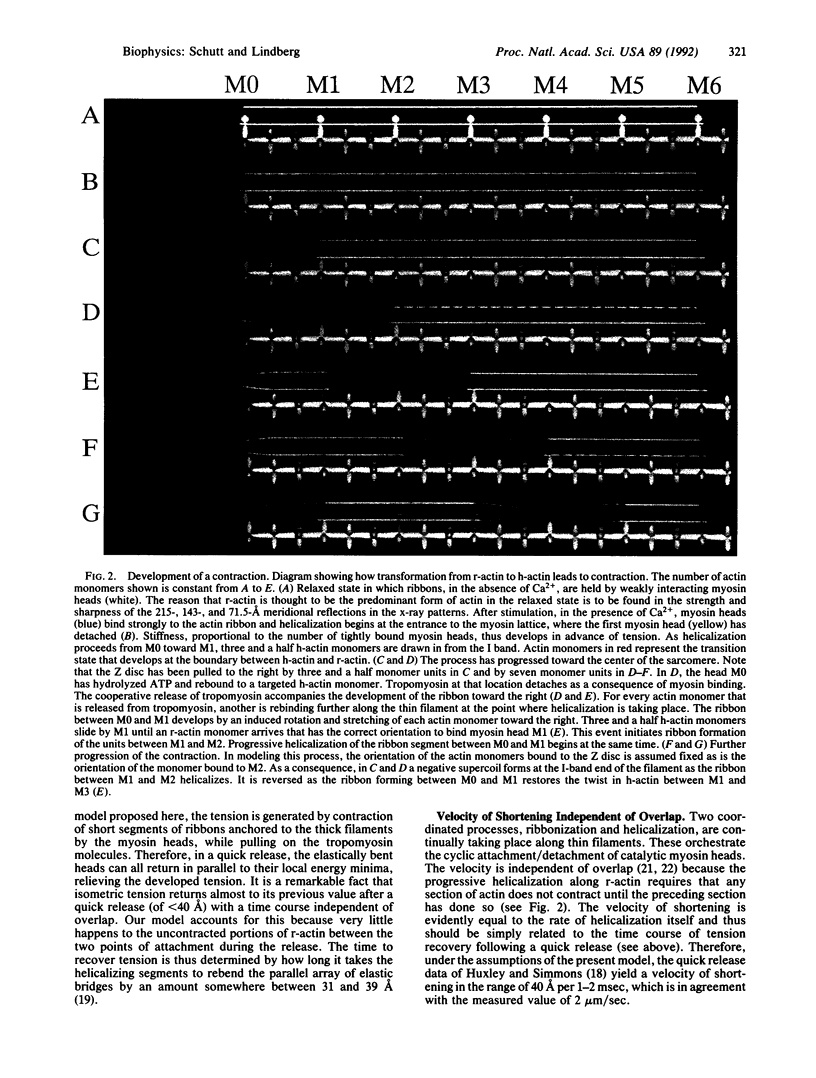
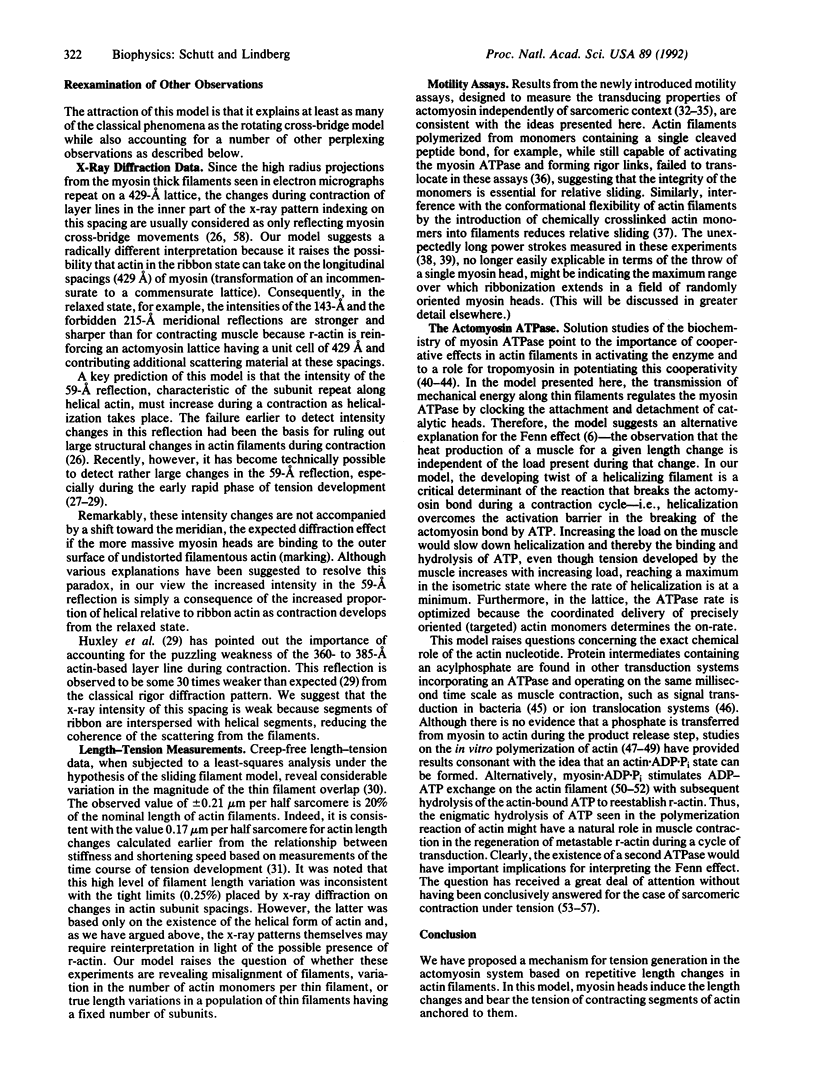
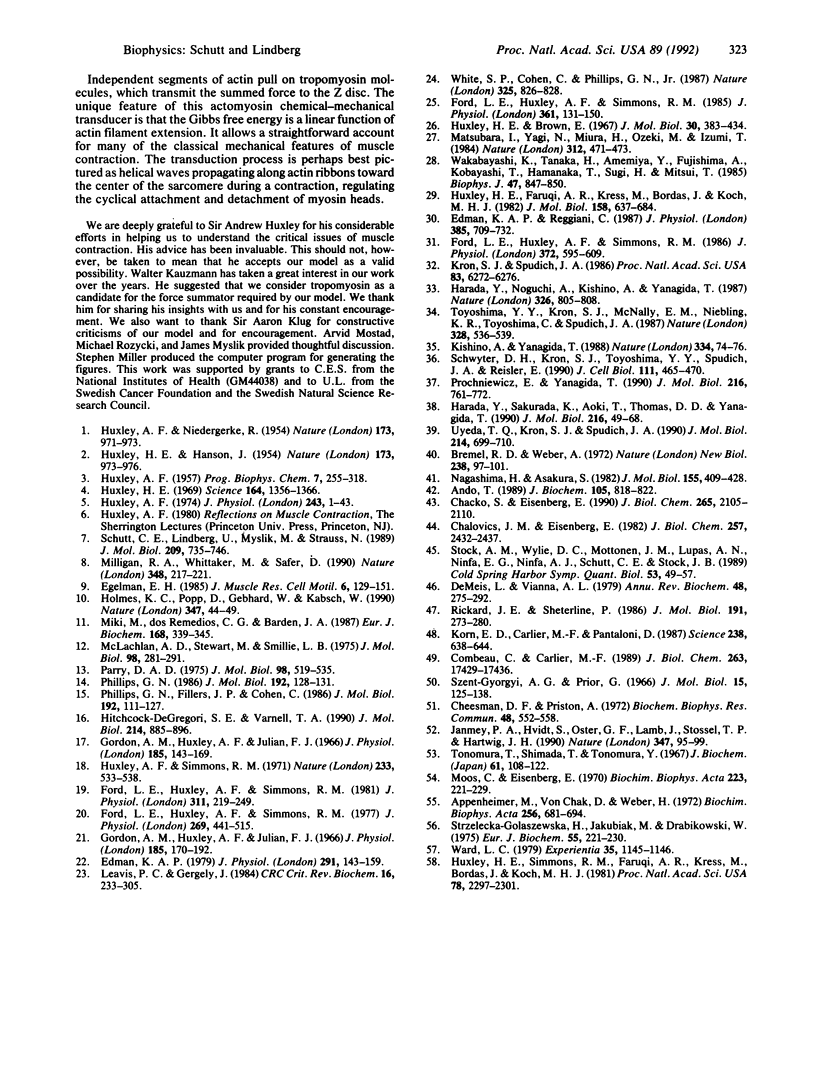
Images in this article
Selected References
These references are in PubMed. This may not be the complete list of references from this article.
- Ando T. Propagation of Acto-S-1 ATPase reaction-coupled conformational change in actin along the filament. J Biochem. 1989 May;105(5):818–822. doi: 10.1093/oxfordjournals.jbchem.a122751. [DOI] [PubMed] [Google Scholar]
- Appenheimer M., von Chak D., Weber H. H. Der Nucleotidaustausch des F-actin in konrahierten, erschlafften und totenstarren Fibrillen in seiner Bedeutung für den molekularen Mechanismus der Muskelkontraktion. Biochim Biophys Acta. 1972 Mar 16;256(3):681–694. doi: 10.1016/0005-2728(72)90203-4. [DOI] [PubMed] [Google Scholar]
- Bremel R. D., Weber A. Cooperation within actin filament in vertebrate skeletal muscle. Nat New Biol. 1972 Jul 26;238(82):97–101. doi: 10.1038/newbio238097a0. [DOI] [PubMed] [Google Scholar]
- Chacko S., Eisenberg E. Cooperativity of actin-activated ATPase of gizzard heavy meromyosin in the presence of gizzard tropomyosin. J Biol Chem. 1990 Feb 5;265(4):2105–2110. [PubMed] [Google Scholar]
- Chalovich J. M., Eisenberg E. Inhibition of actomyosin ATPase activity by troponin-tropomyosin without blocking the binding of myosin to actin. J Biol Chem. 1982 Mar 10;257(5):2432–2437. [PMC free article] [PubMed] [Google Scholar]
- Cheesman D. F., Priston A. Exchange of actin-bound nucleotide in brief electrical stimulation of muscle. Biochem Biophys Res Commun. 1972 Aug 7;48(3):552–558. doi: 10.1016/0006-291x(72)90383-x. [DOI] [PubMed] [Google Scholar]
- Combeau C., Carlier M. F. Probing the mechanism of ATP hydrolysis on F-actin using vanadate and the structural analogs of phosphate BeF-3 and A1F-4. J Biol Chem. 1988 Nov 25;263(33):17429–17436. [PubMed] [Google Scholar]
- Edman K. A., Reggiani C. The sarcomere length-tension relation determined in short segments of intact muscle fibres of the frog. J Physiol. 1987 Apr;385:709–732. doi: 10.1113/jphysiol.1987.sp016516. [DOI] [PMC free article] [PubMed] [Google Scholar]
- Edman K. A. The velocity of unloaded shortening and its relation to sarcomere length and isometric force in vertebrate muscle fibres. J Physiol. 1979 Jun;291:143–159. doi: 10.1113/jphysiol.1979.sp012804. [DOI] [PMC free article] [PubMed] [Google Scholar]
- Egelman E. H. The structure of F-actin. J Muscle Res Cell Motil. 1985 Apr;6(2):129–151. doi: 10.1007/BF00713056. [DOI] [PubMed] [Google Scholar]
- Ford L. E., Huxley A. F., Simmons R. M. Tension responses to sudden length change in stimulated frog muscle fibres near slack length. J Physiol. 1977 Jul;269(2):441–515. doi: 10.1113/jphysiol.1977.sp011911. [DOI] [PMC free article] [PubMed] [Google Scholar]
- Ford L. E., Huxley A. F., Simmons R. M. Tension transients during steady shortening of frog muscle fibres. J Physiol. 1985 Apr;361:131–150. doi: 10.1113/jphysiol.1985.sp015637. [DOI] [PMC free article] [PubMed] [Google Scholar]
- Ford L. E., Huxley A. F., Simmons R. M. Tension transients during the rise of tetanic tension in frog muscle fibres. J Physiol. 1986 Mar;372:595–609. doi: 10.1113/jphysiol.1986.sp016027. [DOI] [PMC free article] [PubMed] [Google Scholar]
- Ford L. E., Huxley A. F., Simmons R. M. The relation between stiffness and filament overlap in stimulated frog muscle fibres. J Physiol. 1981 Feb;311:219–249. doi: 10.1113/jphysiol.1981.sp013582. [DOI] [PMC free article] [PubMed] [Google Scholar]
- Gordon A. M., Huxley A. F., Julian F. J. Tension development in highly stretched vertebrate muscle fibres. J Physiol. 1966 May;184(1):143–169. doi: 10.1113/jphysiol.1966.sp007908. [DOI] [PMC free article] [PubMed] [Google Scholar]
- Gordon A. M., Huxley A. F., Julian F. J. The variation in isometric tension with sarcomere length in vertebrate muscle fibres. J Physiol. 1966 May;184(1):170–192. doi: 10.1113/jphysiol.1966.sp007909. [DOI] [PMC free article] [PubMed] [Google Scholar]
- HUXLEY A. F. Muscle structure and theories of contraction. Prog Biophys Biophys Chem. 1957;7:255–318. [PubMed] [Google Scholar]
- HUXLEY A. F., NIEDERGERKE R. Structural changes in muscle during contraction; interference microscopy of living muscle fibres. Nature. 1954 May 22;173(4412):971–973. doi: 10.1038/173971a0. [DOI] [PubMed] [Google Scholar]
- HUXLEY H., HANSON J. Changes in the cross-striations of muscle during contraction and stretch and their structural interpretation. Nature. 1954 May 22;173(4412):973–976. doi: 10.1038/173973a0. [DOI] [PubMed] [Google Scholar]
- Harada Y., Noguchi A., Kishino A., Yanagida T. Sliding movement of single actin filaments on one-headed myosin filaments. Nature. 1987 Apr 23;326(6115):805–808. doi: 10.1038/326805a0. [DOI] [PubMed] [Google Scholar]
- Harada Y., Sakurada K., Aoki T., Thomas D. D., Yanagida T. Mechanochemical coupling in actomyosin energy transduction studied by in vitro movement assay. J Mol Biol. 1990 Nov 5;216(1):49–68. doi: 10.1016/S0022-2836(05)80060-9. [DOI] [PubMed] [Google Scholar]
- Hitchcock-DeGregori S. E., Varnell T. A. Tropomyosin has discrete actin-binding sites with sevenfold and fourteenfold periodicities. J Mol Biol. 1990 Aug 20;214(4):885–896. doi: 10.1016/0022-2836(90)90343-K. [DOI] [PubMed] [Google Scholar]
- Holmes K. C., Popp D., Gebhard W., Kabsch W. Atomic model of the actin filament. Nature. 1990 Sep 6;347(6288):44–49. doi: 10.1038/347044a0. [DOI] [PubMed] [Google Scholar]
- Huxley A. F. Muscular contraction. J Physiol. 1974 Nov;243(1):1–43. [PMC free article] [PubMed] [Google Scholar]
- Huxley A. F., Simmons R. M. Proposed mechanism of force generation in striated muscle. Nature. 1971 Oct 22;233(5321):533–538. doi: 10.1038/233533a0. [DOI] [PubMed] [Google Scholar]
- Huxley H. E., Brown W. The low-angle x-ray diagram of vertebrate striated muscle and its behaviour during contraction and rigor. J Mol Biol. 1967 Dec 14;30(2):383–434. doi: 10.1016/s0022-2836(67)80046-9. [DOI] [PubMed] [Google Scholar]
- Huxley H. E., Faruqi A. R., Kress M., Bordas J., Koch M. H. Time-resolved X-ray diffraction studies of the myosin layer-line reflections during muscle contraction. J Mol Biol. 1982 Jul 15;158(4):637–684. doi: 10.1016/0022-2836(82)90253-4. [DOI] [PubMed] [Google Scholar]
- Huxley H. E., Simmons R. M., Faruqi A. R., Kress M., Bordas J., Koch M. H. Millisecond time-resolved changes in x-ray reflections from contracting muscle during rapid mechanical transients, recorded using synchrotron radiation. Proc Natl Acad Sci U S A. 1981 Apr;78(4):2297–2301. doi: 10.1073/pnas.78.4.2297. [DOI] [PMC free article] [PubMed] [Google Scholar]
- Huxley H. E. The mechanism of muscular contraction. Science. 1969 Jun 20;164(3886):1356–1365. doi: 10.1126/science.164.3886.1356. [DOI] [PubMed] [Google Scholar]
- Janmey P. A., Hvidt S., Oster G. F., Lamb J., Stossel T. P., Hartwig J. H. Effect of ATP on actin filament stiffness. Nature. 1990 Sep 6;347(6288):95–99. doi: 10.1038/347095a0. [DOI] [PubMed] [Google Scholar]
- Kishino A., Yanagida T. Force measurements by micromanipulation of a single actin filament by glass needles. Nature. 1988 Jul 7;334(6177):74–76. doi: 10.1038/334074a0. [DOI] [PubMed] [Google Scholar]
- Korn E. D., Carlier M. F., Pantaloni D. Actin polymerization and ATP hydrolysis. Science. 1987 Oct 30;238(4827):638–644. doi: 10.1126/science.3672117. [DOI] [PubMed] [Google Scholar]
- Kron S. J., Spudich J. A. Fluorescent actin filaments move on myosin fixed to a glass surface. Proc Natl Acad Sci U S A. 1986 Sep;83(17):6272–6276. doi: 10.1073/pnas.83.17.6272. [DOI] [PMC free article] [PubMed] [Google Scholar]
- Leavis P. C., Gergely J. Thin filament proteins and thin filament-linked regulation of vertebrate muscle contraction. CRC Crit Rev Biochem. 1984;16(3):235–305. doi: 10.3109/10409238409108717. [DOI] [PubMed] [Google Scholar]
- Matsubara I., Yagi N., Miura H., Ozeki M., Izumi T. Intensification of the 5.9-nm actin layer line in contracting muscle. 1984 Nov 29-Dec 5Nature. 312(5993):471–473. doi: 10.1038/312471a0. [DOI] [PubMed] [Google Scholar]
- McLachlan A. D., Stewart M., Smillie L. B. Sequence repeats in alpha-tropomyosin. J Mol Biol. 1975 Oct 25;98(2):281–291. doi: 10.1016/s0022-2836(75)80118-5. [DOI] [PubMed] [Google Scholar]
- Miki M., dos Remedios C. G., Barden J. A. Spatial relationship between the nucleotide-binding site, Lys-61 and Cys-374 in actin and a conformational change induced by myosin subfragment-1 binding. Eur J Biochem. 1987 Oct 15;168(2):339–345. doi: 10.1111/j.1432-1033.1987.tb13425.x. [DOI] [PubMed] [Google Scholar]
- Milligan R. A., Whittaker M., Safer D. Molecular structure of F-actin and location of surface binding sites. Nature. 1990 Nov 15;348(6298):217–221. doi: 10.1038/348217a0. [DOI] [PubMed] [Google Scholar]
- Moos C., Eisenberg E. Effect of myosin on actin-bound nucleotide exchange in the presence and absence of ATP. Biochim Biophys Acta. 1970 Dec 8;223(2):221–229. doi: 10.1016/0005-2728(70)90179-9. [DOI] [PubMed] [Google Scholar]
- Nagashima H., Asakura S. Studies on co-operative properties of tropomyosin-actin and tropomyosin-troponin-actin complexes by the use of N-ethylmaleimide-treated and untreated species of myosin subfragment 1. J Mol Biol. 1982 Mar 15;155(4):409–428. doi: 10.1016/0022-2836(82)90479-x. [DOI] [PubMed] [Google Scholar]
- Parry D. A. Analysis of the primary sequence of alpha-tropomyosin from rabbit skeletal muscle. J Mol Biol. 1975 Nov 5;98(3):519–535. doi: 10.1016/s0022-2836(75)80084-2. [DOI] [PubMed] [Google Scholar]
- Phillips G. N., Jr Construction of an atomic model for tropomyosin and implications for interactions with actin. J Mol Biol. 1986 Nov 5;192(1):128–131. doi: 10.1016/0022-2836(86)90469-9. [DOI] [PubMed] [Google Scholar]
- Phillips G. N., Jr, Fillers J. P., Cohen C. Tropomyosin crystal structure and muscle regulation. J Mol Biol. 1986 Nov 5;192(1):111–131. doi: 10.1016/0022-2836(86)90468-7. [DOI] [PubMed] [Google Scholar]
- Prochniewicz E., Yanagida T. Inhibition of sliding movement of F-actin by crosslinking emphasizes the role of actin structure in the mechanism of motility. J Mol Biol. 1990 Dec 5;216(3):761–772. doi: 10.1016/0022-2836(90)90397-5. [DOI] [PubMed] [Google Scholar]
- Rickard J. E., Sheterline P. Cytoplasmic concentrations of inorganic phosphate affect the critical concentration for assembly of actin in the presence of cytochalasin D or ADP. J Mol Biol. 1986 Sep 20;191(2):273–280. doi: 10.1016/0022-2836(86)90264-0. [DOI] [PubMed] [Google Scholar]
- Schutt C. E., Lindberg U., Myslik J., Strauss N. Molecular packing in profilin: actin crystals and its implications. J Mol Biol. 1989 Oct 20;209(4):735–746. doi: 10.1016/0022-2836(89)90603-7. [DOI] [PubMed] [Google Scholar]
- Schwyter D. H., Kron S. J., Toyoshima Y. Y., Spudich J. A., Reisler E. Subtilisin cleavage of actin inhibits in vitro sliding movement of actin filaments over myosin. J Cell Biol. 1990 Aug;111(2):465–470. doi: 10.1083/jcb.111.2.465. [DOI] [PMC free article] [PubMed] [Google Scholar]
- Stock A. M., Wylie D. C., Mottonen J. M., Lupas A. N., Ninfa E. G., Ninfa A. J., Schutt C. E., Stock J. B. Phosphoproteins involved in bacterial signal transduction. Cold Spring Harb Symp Quant Biol. 1988;53(Pt 1):49–57. doi: 10.1101/sqb.1988.053.01.009. [DOI] [PubMed] [Google Scholar]
- Strzelecka-Golaszewska H., Jakubiak M., Drabikowski W. Changes in the state of actin during superprecipitation of actomyosin. Eur J Biochem. 1975 Jun 16;55(1):221–230. doi: 10.1111/j.1432-1033.1975.tb02154.x. [DOI] [PubMed] [Google Scholar]
- Tokiwa T., Shimada T., Tonomura Y. Role of ADP of F-actin in superprecipitation and enzymatic activity of actomyosin. J Biochem. 1967 Jan;61(1):108–122. doi: 10.1093/oxfordjournals.jbchem.a128510. [DOI] [PubMed] [Google Scholar]
- Toyoshima Y. Y., Kron S. J., McNally E. M., Niebling K. R., Toyoshima C., Spudich J. A. Myosin subfragment-1 is sufficient to move actin filaments in vitro. Nature. 1987 Aug 6;328(6130):536–539. doi: 10.1038/328536a0. [DOI] [PubMed] [Google Scholar]
- Uyeda T. Q., Kron S. J., Spudich J. A. Myosin step size. Estimation from slow sliding movement of actin over low densities of heavy meromyosin. J Mol Biol. 1990 Aug 5;214(3):699–710. doi: 10.1016/0022-2836(90)90287-V. [DOI] [PubMed] [Google Scholar]
- Wakabayashi K., Tanaka H., Amemiya Y., Fujishima A., Kobayashi T., Hamanaka T., Sugi H., Mitsui T. Time-resolved x-ray diffraction studies on the intensity changes of the 5.9 and 5.1 nm actin layer lines from frog skeletal muscle during an isometric tetanus using synchrotron radiation. Biophys J. 1985 Jun;47(6):847–850. doi: 10.1016/S0006-3495(85)83989-8. [DOI] [PMC free article] [PubMed] [Google Scholar]
- Ward L. C. The turnover of F-actin-bound ADP in vivo. Experientia. 1979 Sep 15;35(9):1145–1146. doi: 10.1007/BF01963250. [DOI] [PubMed] [Google Scholar]
- White S. P., Cohen C., Phillips G. N., Jr Structure of co-crystals of tropomyosin and troponin. 1987 Feb 26-Mar 4Nature. 325(6107):826–828. doi: 10.1038/325826a0. [DOI] [PubMed] [Google Scholar]
- de Meis L., Vianna A. L. Energy interconversion by the Ca2+-dependent ATPase of the sarcoplasmic reticulum. Annu Rev Biochem. 1979;48:275–292. doi: 10.1146/annurev.bi.48.070179.001423. [DOI] [PubMed] [Google Scholar]