Abstract
Protein-protein bond formations, such as antibody-antigen complexation or aggregation of protein monomers into dimers and larger aggregates, occur with bimolecular rate constants on the order of 10(6) M-1.s-1, which is only 3 orders of magnitude slower than the diffusion-limited Smoluchowski rate. However, since the protein-protein bond requires rotational alignment to within a few angstroms of tolerance, purely geometric estimates would suggest that the observed rates might be 6 orders of magnitude below the Smoluchowski rate. Previous theoretical treatments have not been solved for the highly specific docking criteria of protein-protein association--the entire subunit interface must be aligned within 2 A of the correct position. Several studies have suggested that diffusion alone could not produce the rapid association kinetics and have postulated "lengthy collisions" and/or the operation of electrostatic or hydrophobic steering forces to accelerate the association. In the present study, the Brownian dynamics simulation method is used to compute the rate of association of neutral spherical model proteins with the stated docking criteria. The Brownian simulation predicts a rate of 2 x 10(6) M-1.s-1 for this generic protein-protein association, a rate that is 2000 times faster than that predicted by the simplest geometric calculation and is essentially equal to the rates observed for protein-protein association in aqueous solution. This high rate is obtained by simple diffusive processes and does not require any attractive or steering forces beyond those achieved for a partially formed bond. The rate enhancement is attributed to a diffusive entrapment effect, in which a protein pair surrounded and trapped by water undergoes multiple collisions with rotational reorientation during each encounter.
Full text
PDF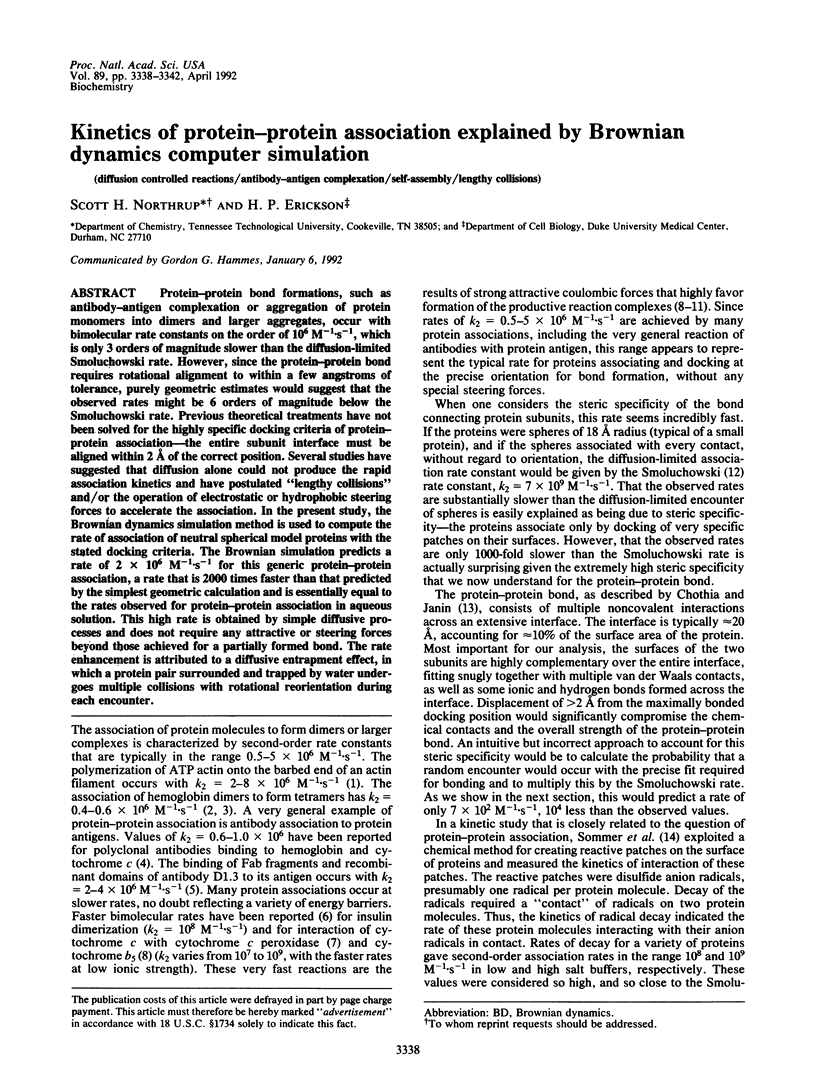
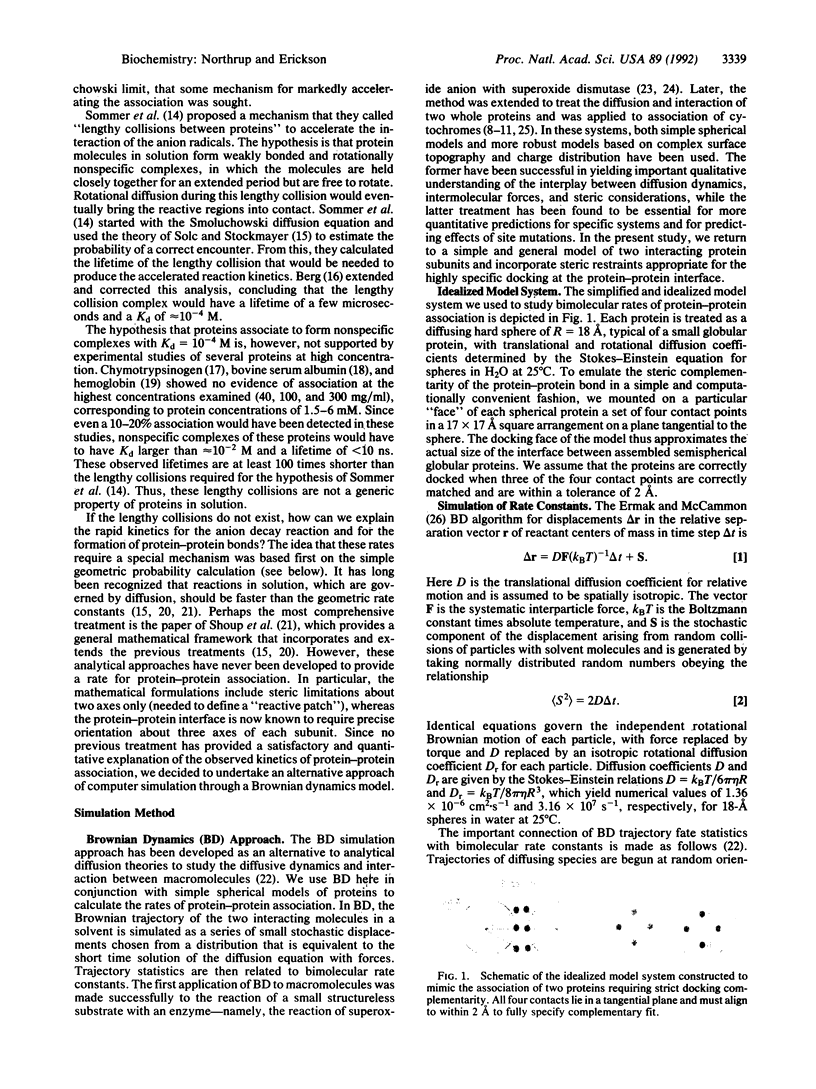
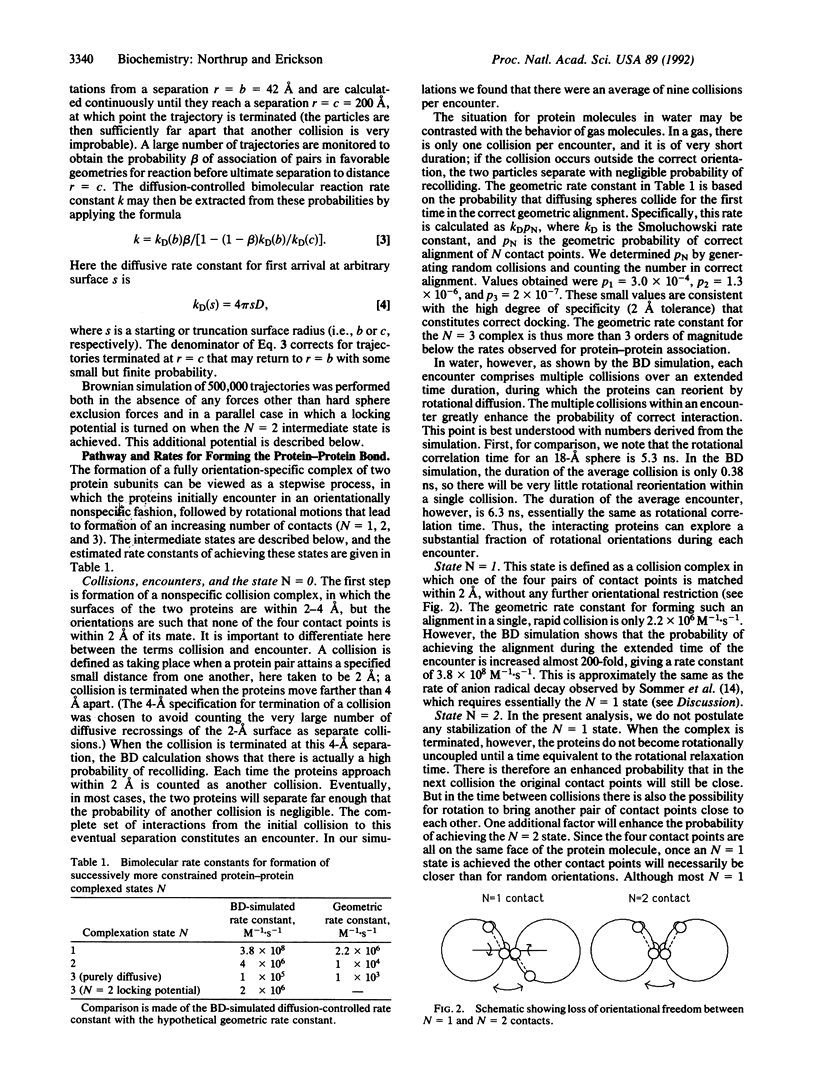
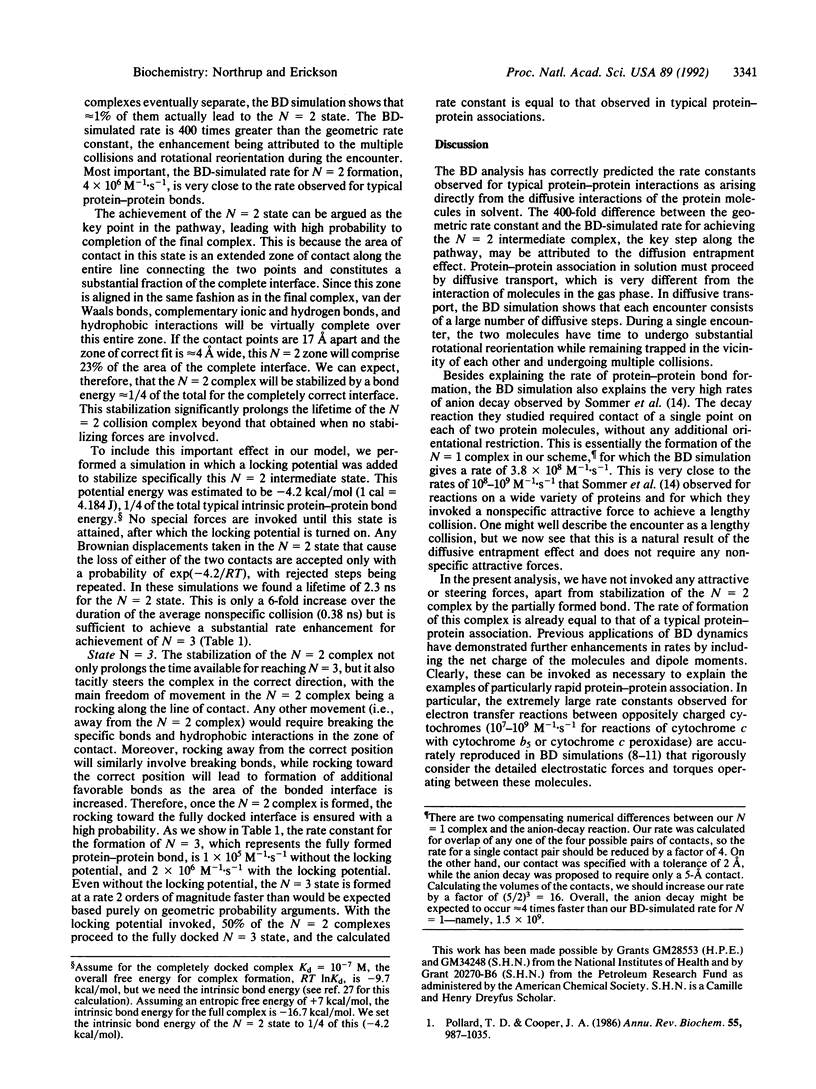
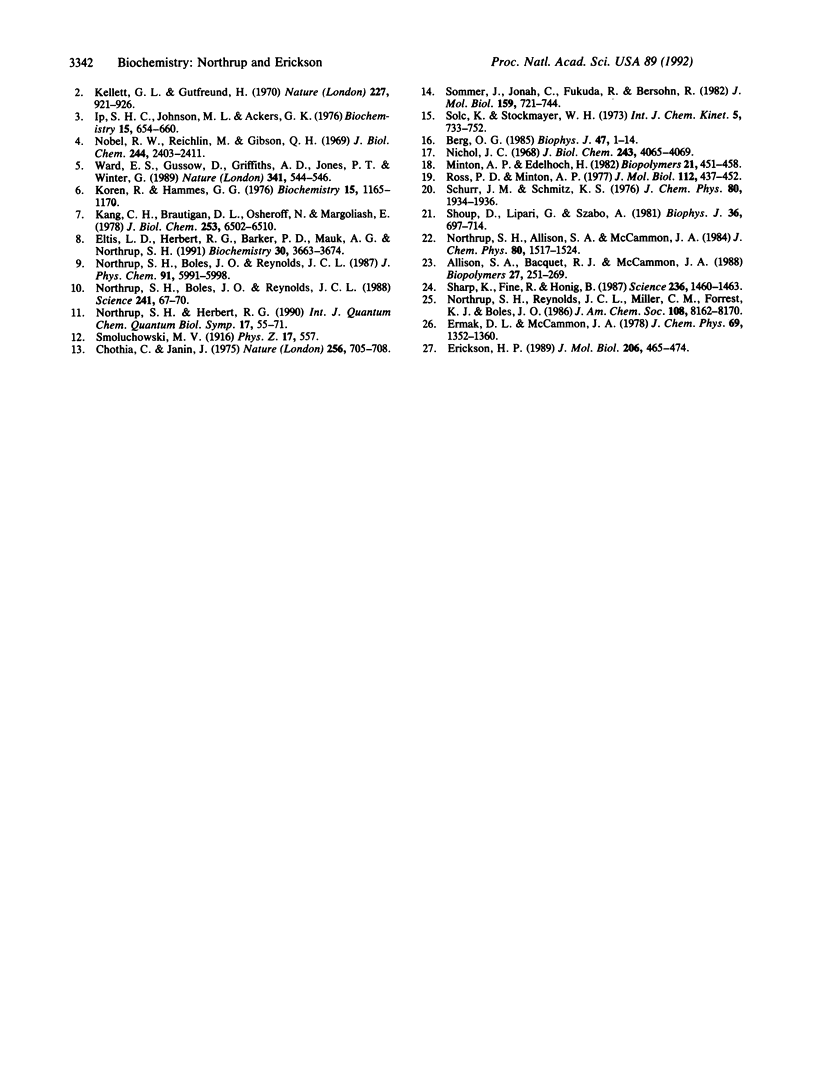
Images in this article
Selected References
These references are in PubMed. This may not be the complete list of references from this article.
- Allison S. A., Bacquet R. J., McCammon J. A. Simulation of the diffusion-controlled reaction between superoxide and superoxide dismutase. II. Detailed models. Biopolymers. 1988 Feb;27(2):251–269. doi: 10.1002/bip.360270207. [DOI] [PubMed] [Google Scholar]
- Berg O. G. Orientation constraints in diffusion-limited macromolecular association. The role of surface diffusion as a rate-enhancing mechanism. Biophys J. 1985 Jan;47(1):1–14. doi: 10.1016/S0006-3495(85)83870-4. [DOI] [PMC free article] [PubMed] [Google Scholar]
- Chothia C., Janin J. Principles of protein-protein recognition. Nature. 1975 Aug 28;256(5520):705–708. doi: 10.1038/256705a0. [DOI] [PubMed] [Google Scholar]
- Eltis L. D., Herbert R. G., Barker P. D., Mauk A. G., Northrup S. H. Reduction of horse heart ferricytochrome c by bovine liver ferrocytochrome b5. Experimental and theoretical analysis. Biochemistry. 1991 Apr 16;30(15):3663–3674. doi: 10.1021/bi00229a011. [DOI] [PubMed] [Google Scholar]
- Erickson H. P. Co-operativity in protein-protein association. The structure and stability of the actin filament. J Mol Biol. 1989 Apr 5;206(3):465–474. doi: 10.1016/0022-2836(89)90494-4. [DOI] [PubMed] [Google Scholar]
- Ip S. H., Johnson M. L., Ackers G. K. Kinetics of deoxyhemoglobin subunit dissociation determined by haptoglobin binding: estimation of the equilibrium constant from forward and reverse rates. Biochemistry. 1976 Feb 10;15(3):654–660. doi: 10.1021/bi00648a032. [DOI] [PubMed] [Google Scholar]
- Kang C. H., Brautigan D. L., Osheroff N., Margoliash E. Definitaion of cytochrome c binding domains by chemical modification. Reaction of carboxydinitrophenyl- and trinitrophenyl-cytochromes c with baker's yeast cytochrome c peroxidase. J Biol Chem. 1978 Sep 25;253(18):6502–6510. [PubMed] [Google Scholar]
- Kellett G. L., Gutfreund H. Reactions of haemoglobin dimers after ligand dissociation. Nature. 1970 Aug 29;227(5261):921–926. doi: 10.1038/227921a0. [DOI] [PubMed] [Google Scholar]
- Koren R., Hammes G. G. A kinetic study of protein-protein interactions. Biochemistry. 1976 Mar 9;15(5):1165–1171. doi: 10.1021/bi00650a032. [DOI] [PubMed] [Google Scholar]
- Nichol J. C. Sedimentation behavior of chymotrypsinogen A in the vicinity of the isoelectric point. J Biol Chem. 1968 Aug 10;243(15):4065–4069. [PubMed] [Google Scholar]
- Noble R. W., Reichlin M., Gibson Q. H. The reactions of antibodies with hemeprotein antgens. The measurement of reaction kinetics and stoichiometry by fluorescence quenching. J Biol Chem. 1969 May 10;244(9):2403–2411. [PubMed] [Google Scholar]
- Northrup S. H., Boles J. O., Reynolds J. C. Brownian dynamics of cytochrome c and cytochrome c peroxidase association. Science. 1988 Jul 1;241(4861):67–70. doi: 10.1126/science.2838904. [DOI] [PubMed] [Google Scholar]
- Pollard T. D., Cooper J. A. Actin and actin-binding proteins. A critical evaluation of mechanisms and functions. Annu Rev Biochem. 1986;55:987–1035. doi: 10.1146/annurev.bi.55.070186.005011. [DOI] [PubMed] [Google Scholar]
- Ross P. D., Minton A. P. Analysis of non-ideal behavior in concentrated hemoglobin solutions. J Mol Biol. 1977 May 25;112(3):437–452. doi: 10.1016/s0022-2836(77)80191-5. [DOI] [PubMed] [Google Scholar]
- Sharp K., Fine R., Honig B. Computer simulations of the diffusion of a substrate to an active site of an enzyme. Science. 1987 Jun 12;236(4807):1460–1463. doi: 10.1126/science.3589666. [DOI] [PubMed] [Google Scholar]
- Shoup D., Lipari G., Szabo A. Diffusion-controlled bimolecular reaction rates. The effect of rotational diffusion and orientation constraints. Biophys J. 1981 Dec;36(3):697–714. doi: 10.1016/S0006-3495(81)84759-5. [DOI] [PMC free article] [PubMed] [Google Scholar]
- Sommer J., Jonah C., Fukuda R., Bersohn R. Production and subsequent second-order decomposition of protein disulfide anions lengthy collisions between proteins. J Mol Biol. 1982 Aug 25;159(4):721–744. doi: 10.1016/0022-2836(82)90110-3. [DOI] [PubMed] [Google Scholar]
- Ward E. S., Güssow D., Griffiths A. D., Jones P. T., Winter G. Binding activities of a repertoire of single immunoglobulin variable domains secreted from Escherichia coli. Nature. 1989 Oct 12;341(6242):544–546. doi: 10.1038/341544a0. [DOI] [PubMed] [Google Scholar]