Abstract
We describe an efficient method for the construction of small-insert genomic libraries enriched for highly polymorphic, simple sequence repeats. With this approach, libraries in which 40-50% of the members contain (CA)n repeats are produced, representing an approximately 50-fold enrichment over conventional small-insert genomic DNA libraries. Briefly, a genomic library with an average insert size of less than 500 base pairs was constructed in a phagemid vector. Amplification of this library in a dut ung strain of Escherichia coli allowed the recovery of the library as closed circular single-stranded DNA with uracil frequently incorporated in place of thymine. This DNA was used as a template for second-strand DNA synthesis, primed with (CA)n or (TG)n oligonucleotides, at elevated temperatures by a thermostable DNA polymerase. Transformation of this mixture into wild-type E. coli strains resulted in the recovery of primer-extended products as a consequence of the strong genetic selection against single-stranded uracil-containing DNA molecules. In this manner, a library highly enriched for the targeted microsatellite-containing clones was recovered. This approach is widely applicable and can be used to generate marker-selected libraries bearing any simple sequence repeat from cDNAs, whole genomes, single chromosomes, or more restricted chromosomal regions of interest.
Full text
PDF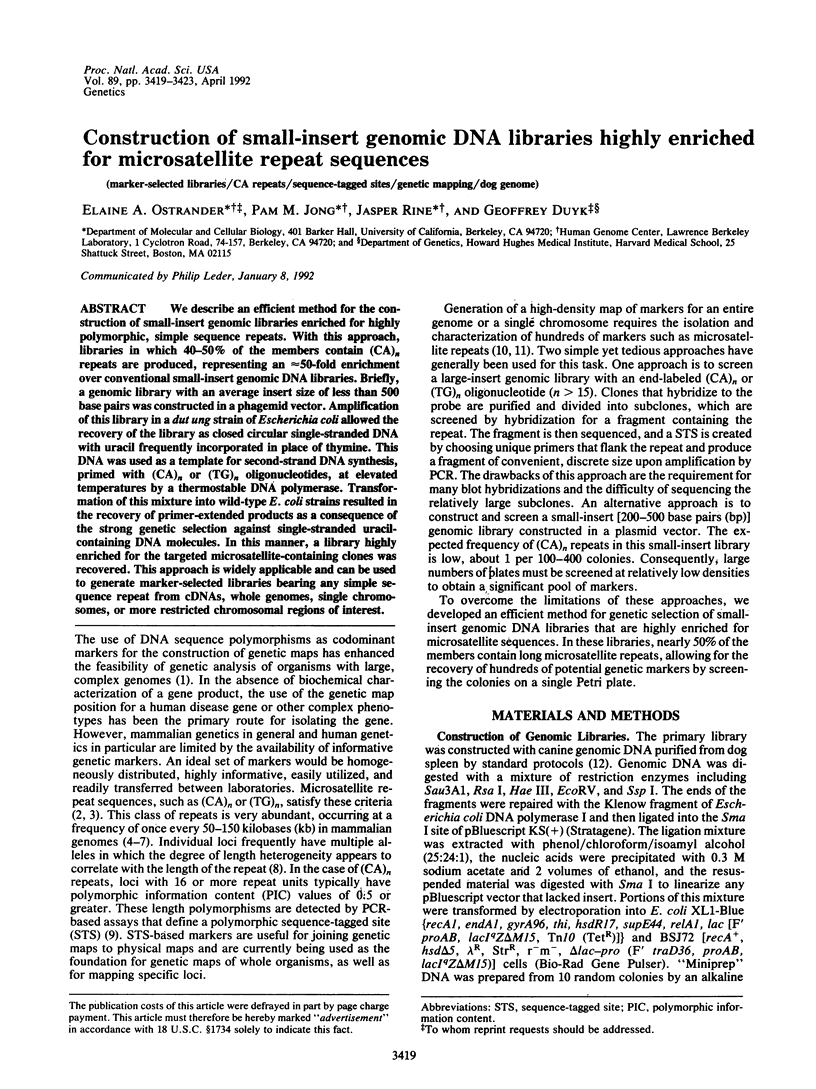
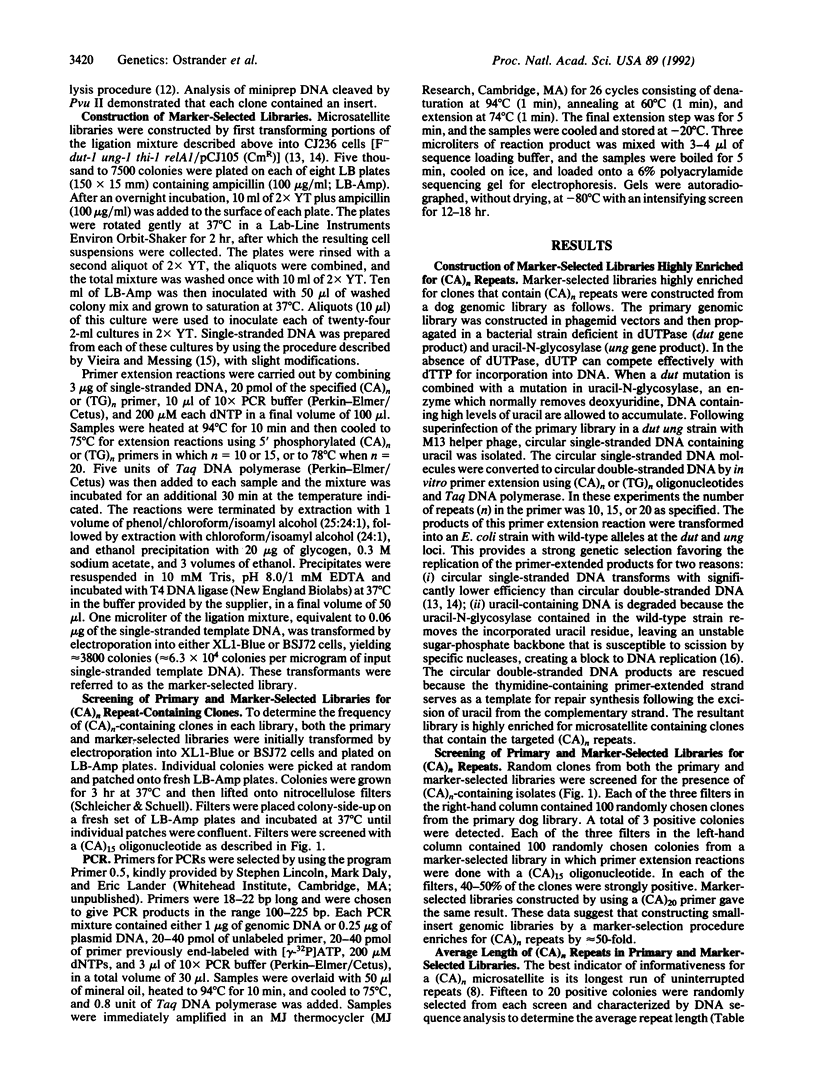
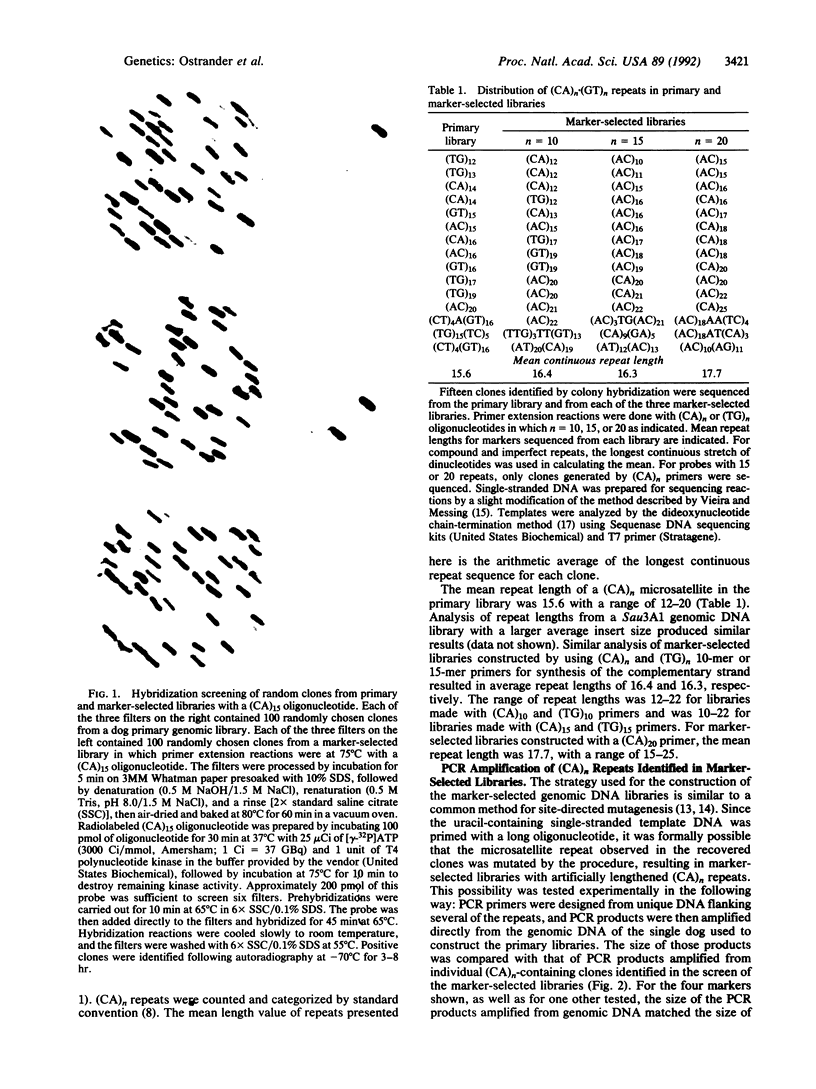
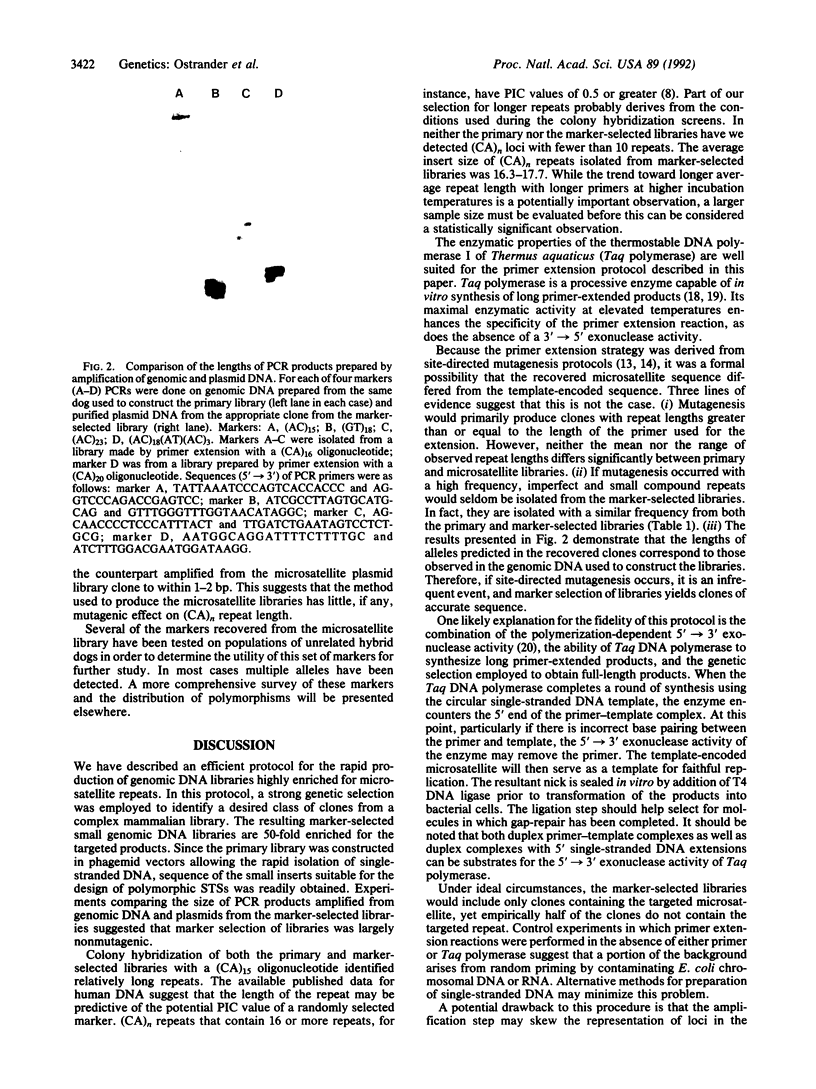
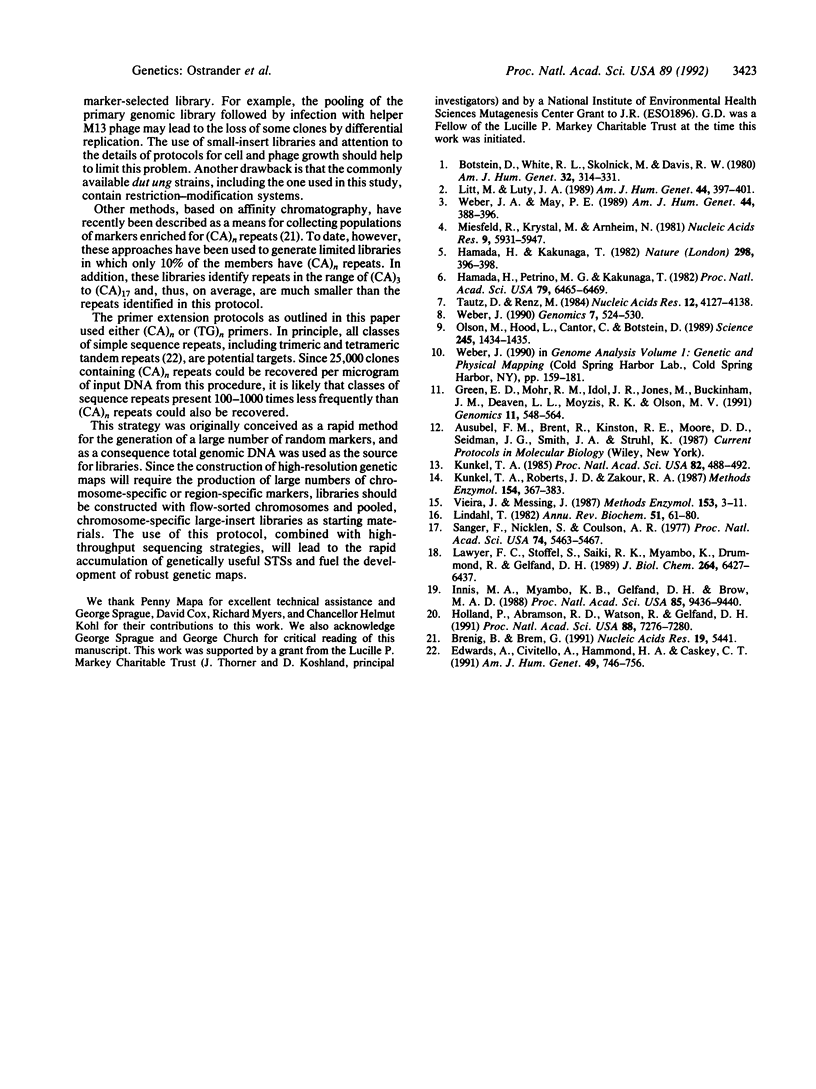
Images in this article
Selected References
These references are in PubMed. This may not be the complete list of references from this article.
- Botstein D., White R. L., Skolnick M., Davis R. W. Construction of a genetic linkage map in man using restriction fragment length polymorphisms. Am J Hum Genet. 1980 May;32(3):314–331. [PMC free article] [PubMed] [Google Scholar]
- Brenig B., Brem G. Direct cloning of sequence tagged microsatellite sites by DNA affinity chromatography. Nucleic Acids Res. 1991 Oct 11;19(19):5441–5441. doi: 10.1093/nar/19.19.5441. [DOI] [PMC free article] [PubMed] [Google Scholar]
- Edwards A., Civitello A., Hammond H. A., Caskey C. T. DNA typing and genetic mapping with trimeric and tetrameric tandem repeats. Am J Hum Genet. 1991 Oct;49(4):746–756. [PMC free article] [PubMed] [Google Scholar]
- Green E. D., Mohr R. M., Idol J. R., Jones M., Buckingham J. M., Deaven L. L., Moyzis R. K., Olson M. V. Systematic generation of sequence-tagged sites for physical mapping of human chromosomes: application to the mapping of human chromosome 7 using yeast artificial chromosomes. Genomics. 1991 Nov;11(3):548–564. doi: 10.1016/0888-7543(91)90062-j. [DOI] [PubMed] [Google Scholar]
- Hamada H., Kakunaga T. Potential Z-DNA forming sequences are highly dispersed in the human genome. Nature. 1982 Jul 22;298(5872):396–398. doi: 10.1038/298396a0. [DOI] [PubMed] [Google Scholar]
- Hamada H., Petrino M. G., Kakunaga T. A novel repeated element with Z-DNA-forming potential is widely found in evolutionarily diverse eukaryotic genomes. Proc Natl Acad Sci U S A. 1982 Nov;79(21):6465–6469. doi: 10.1073/pnas.79.21.6465. [DOI] [PMC free article] [PubMed] [Google Scholar]
- Holland P. M., Abramson R. D., Watson R., Gelfand D. H. Detection of specific polymerase chain reaction product by utilizing the 5'----3' exonuclease activity of Thermus aquaticus DNA polymerase. Proc Natl Acad Sci U S A. 1991 Aug 15;88(16):7276–7280. doi: 10.1073/pnas.88.16.7276. [DOI] [PMC free article] [PubMed] [Google Scholar]
- Innis M. A., Myambo K. B., Gelfand D. H., Brow M. A. DNA sequencing with Thermus aquaticus DNA polymerase and direct sequencing of polymerase chain reaction-amplified DNA. Proc Natl Acad Sci U S A. 1988 Dec;85(24):9436–9440. doi: 10.1073/pnas.85.24.9436. [DOI] [PMC free article] [PubMed] [Google Scholar]
- Kunkel T. A. Rapid and efficient site-specific mutagenesis without phenotypic selection. Proc Natl Acad Sci U S A. 1985 Jan;82(2):488–492. doi: 10.1073/pnas.82.2.488. [DOI] [PMC free article] [PubMed] [Google Scholar]
- Kunkel T. A., Roberts J. D., Zakour R. A. Rapid and efficient site-specific mutagenesis without phenotypic selection. Methods Enzymol. 1987;154:367–382. doi: 10.1016/0076-6879(87)54085-x. [DOI] [PubMed] [Google Scholar]
- Lawyer F. C., Stoffel S., Saiki R. K., Myambo K., Drummond R., Gelfand D. H. Isolation, characterization, and expression in Escherichia coli of the DNA polymerase gene from Thermus aquaticus. J Biol Chem. 1989 Apr 15;264(11):6427–6437. [PubMed] [Google Scholar]
- Lindahl T. DNA repair enzymes. Annu Rev Biochem. 1982;51:61–87. doi: 10.1146/annurev.bi.51.070182.000425. [DOI] [PubMed] [Google Scholar]
- Litt M., Luty J. A. A hypervariable microsatellite revealed by in vitro amplification of a dinucleotide repeat within the cardiac muscle actin gene. Am J Hum Genet. 1989 Mar;44(3):397–401. [PMC free article] [PubMed] [Google Scholar]
- Miesfeld R., Krystal M., Arnheim N. A member of a new repeated sequence family which is conserved throughout eucaryotic evolution is found between the human delta and beta globin genes. Nucleic Acids Res. 1981 Nov 25;9(22):5931–5947. doi: 10.1093/nar/9.22.5931. [DOI] [PMC free article] [PubMed] [Google Scholar]
- Olson M., Hood L., Cantor C., Botstein D. A common language for physical mapping of the human genome. Science. 1989 Sep 29;245(4925):1434–1435. doi: 10.1126/science.2781285. [DOI] [PubMed] [Google Scholar]
- Sanger F., Nicklen S., Coulson A. R. DNA sequencing with chain-terminating inhibitors. Proc Natl Acad Sci U S A. 1977 Dec;74(12):5463–5467. doi: 10.1073/pnas.74.12.5463. [DOI] [PMC free article] [PubMed] [Google Scholar]
- Tautz D., Renz M. Simple sequences are ubiquitous repetitive components of eukaryotic genomes. Nucleic Acids Res. 1984 May 25;12(10):4127–4138. doi: 10.1093/nar/12.10.4127. [DOI] [PMC free article] [PubMed] [Google Scholar]
- Vieira J., Messing J. Production of single-stranded plasmid DNA. Methods Enzymol. 1987;153:3–11. doi: 10.1016/0076-6879(87)53044-0. [DOI] [PubMed] [Google Scholar]
- Weber J. L. Informativeness of human (dC-dA)n.(dG-dT)n polymorphisms. Genomics. 1990 Aug;7(4):524–530. doi: 10.1016/0888-7543(90)90195-z. [DOI] [PubMed] [Google Scholar]