Abstract
The middle base (U35) of the anticodon of tRNA(Gln) is a major element ensuring the accuracy of aminoacylation by Escherichia coli glutaminyl-tRNA synthetase (GlnRS). An opal suppressor of tRNA(Gln) (su+2UGA) containing C35 (anticodon UCA) was isolated by genetic selection and mutagenesis. Suppression of a UGA mutation in the E. coli fol gene followed by N-terminal sequence analysis of purified dihydrofolate reductase showed that this tRNA was an efficient suppressor that inserted predominantly tryptophan. Mutations of the 3-70 base pair (U70 and A3U70) were made. These mutants of su+2UGA are less efficient suppressors and inserted predominantly tryptophan in vivo; alanine insertion was not observed. Mutations of the discriminator nucleotide (A73, U73, C73) result in very weak opal suppressors. Aminoacylation in vitro by E. coli TrpRS of tRNA(Gln) transcripts mutated in the anticodon demonstrate that TrpRS recognizes all three nucleotides of the anticodon. The results show the interchangeability of the glutamine and tryptophan identities by base substitutions in their respective tRNAs. The amber suppressor (anticodon CUA) tRNA(Trp) was known previously to insert predominantly glutamine. We show that the opal suppressor (anticodon UCA) tRNA(Gln) inserts mainly tryptophan. Discrimination by these synthetases for tRNA includes position 35, with recognition of C35 by TrpRS and U35 by GlnRS. As the use of the UGA codon as tryptophan in mycoplasma and in yeast mitochondria is conserved, recognition of the UCA anticodon by TrpRS may also be maintained in evolution.
Full text
PDF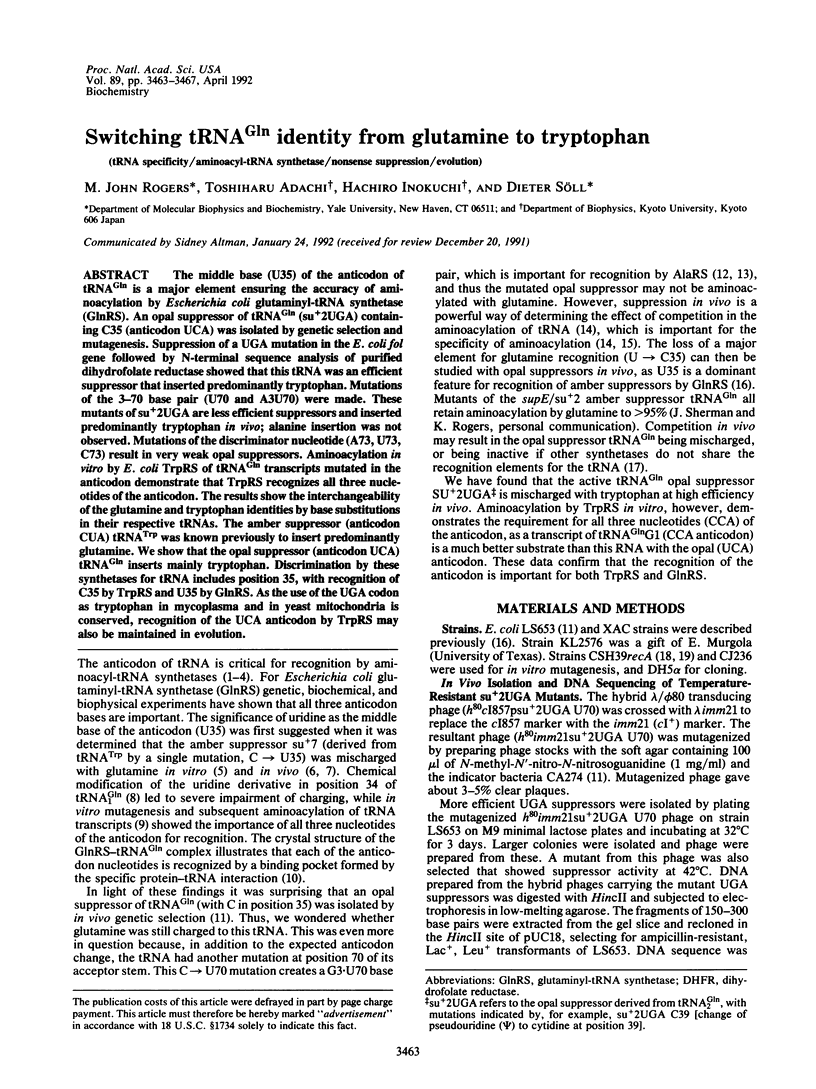
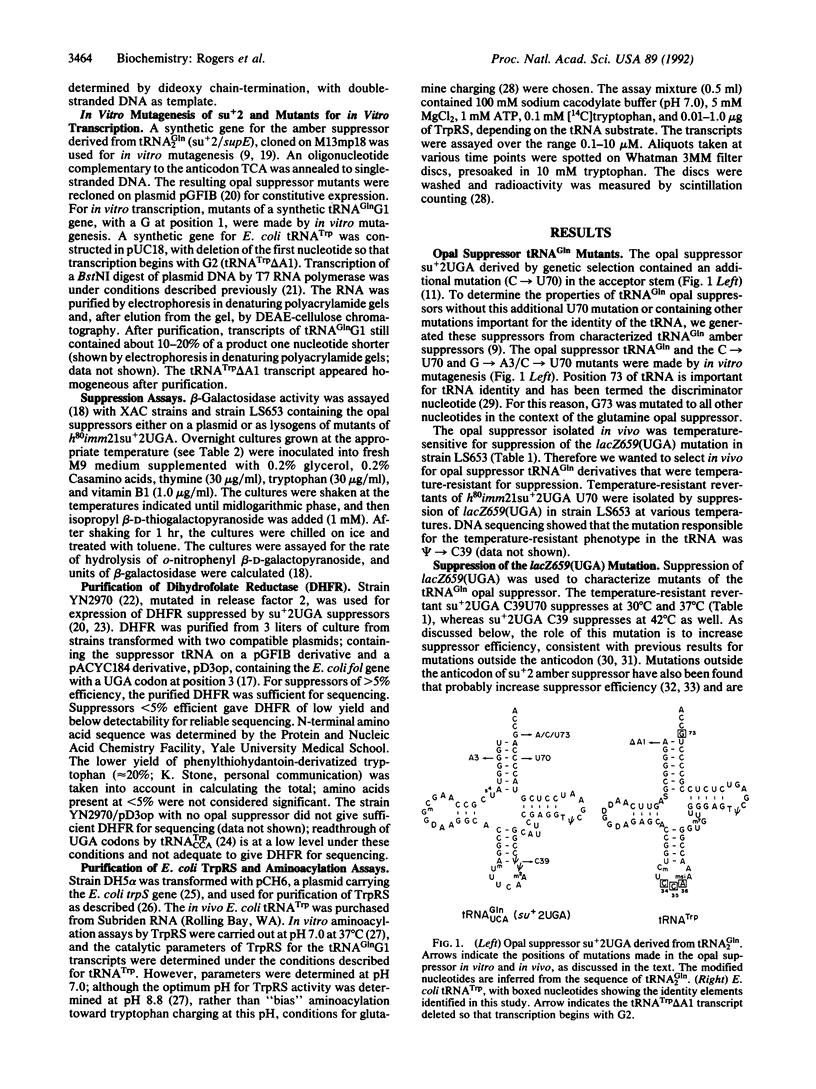
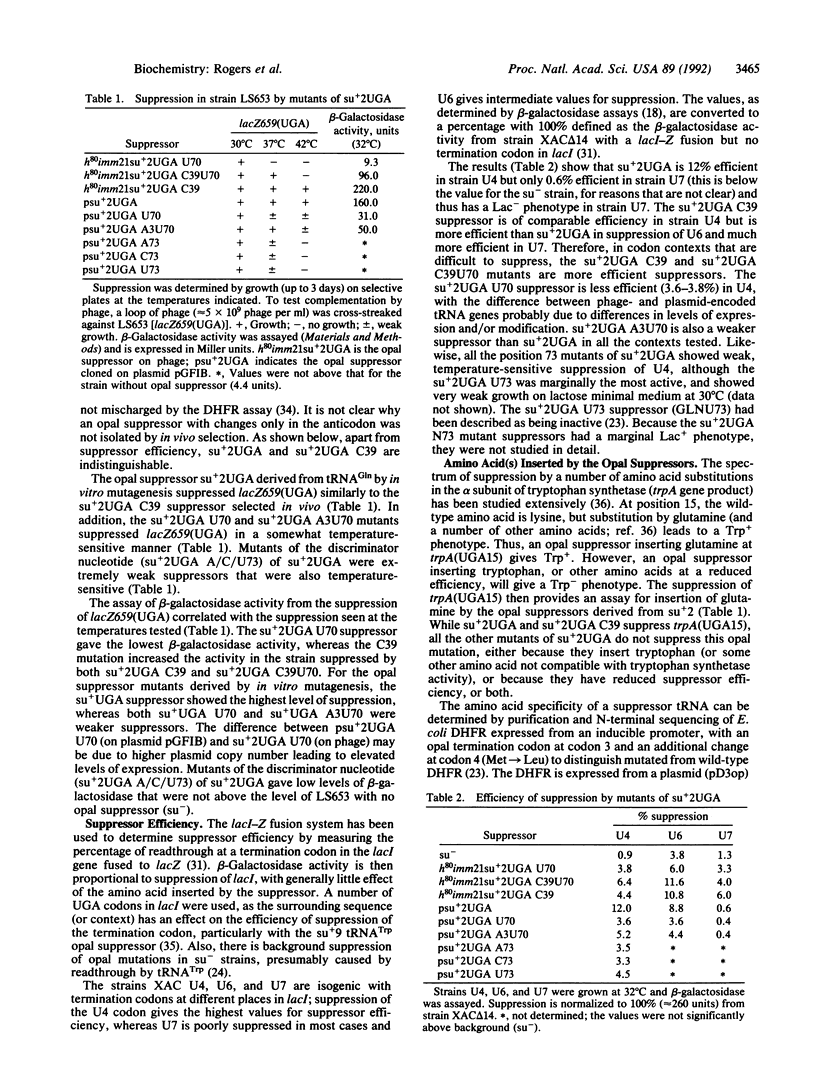
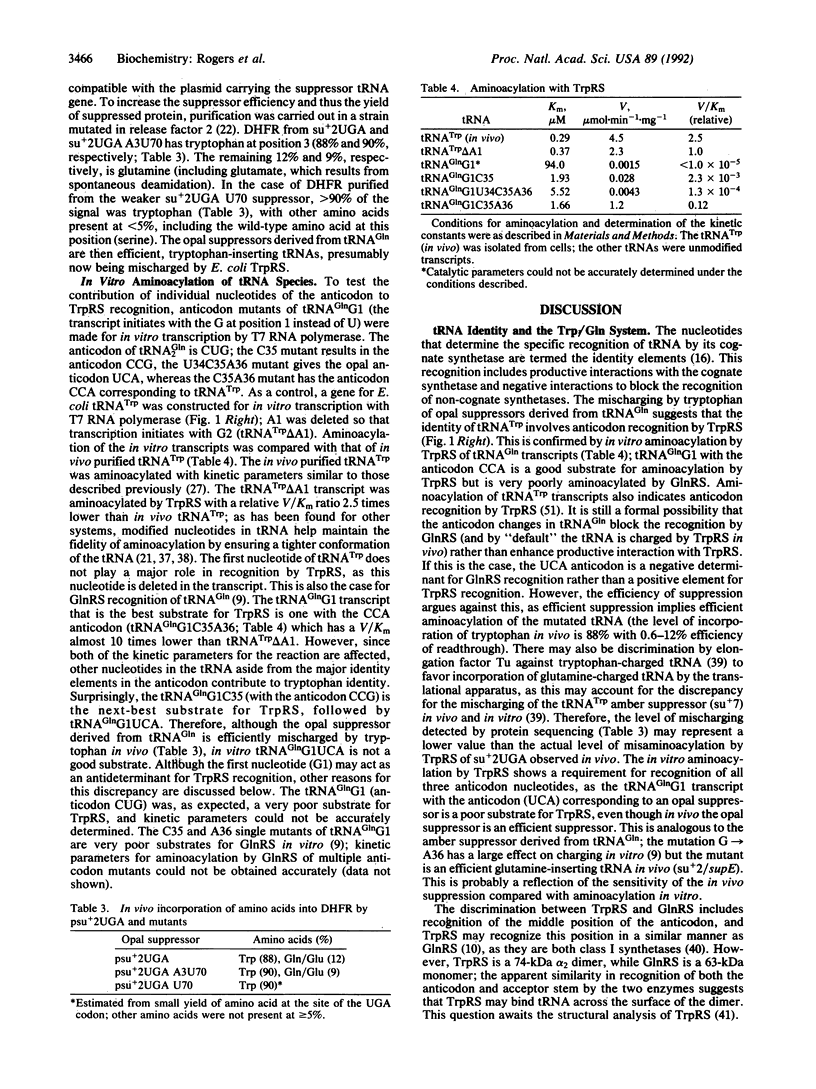
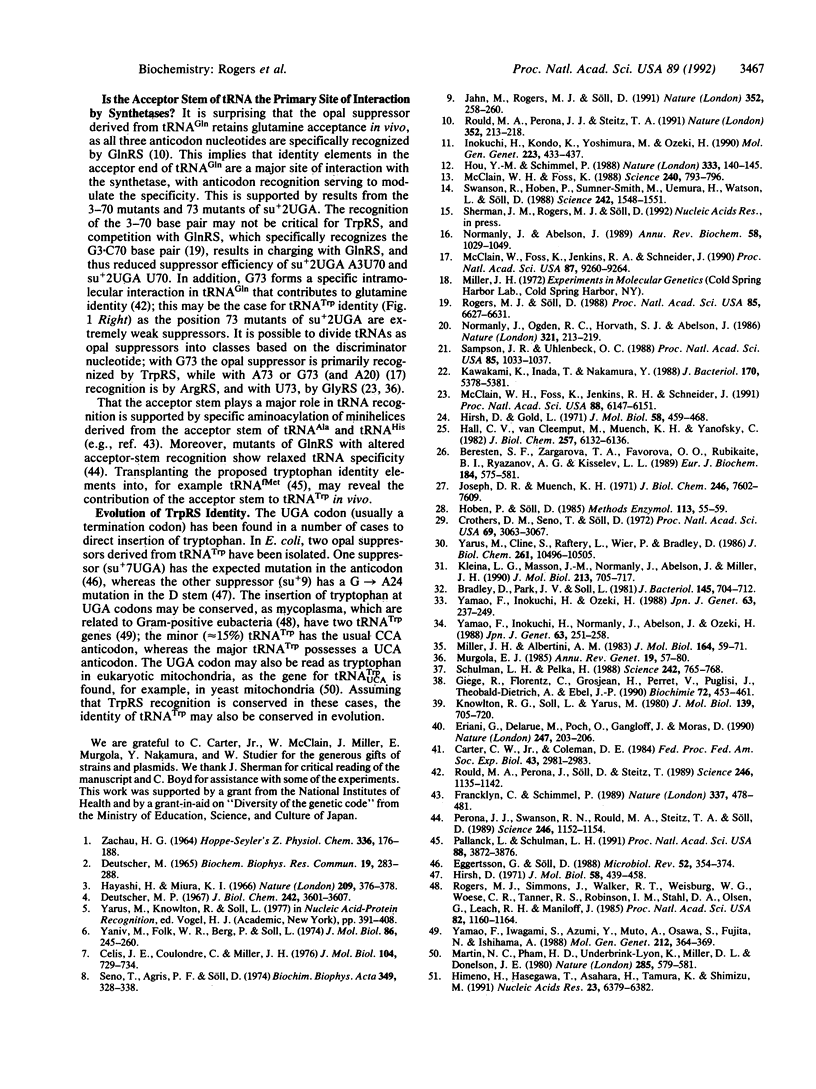
Selected References
These references are in PubMed. This may not be the complete list of references from this article.
- Beresten S. F., Zargarova T. A., Favorova O. O., Rubikaite B. I., Ryazanov A. G., Kisselev L. L. Molecular and cellular studies of tryptophanyl-tRNA synthetase using monoclonal antibodies. Evaluation of a common antigenic determinant in eukaryotic, prokaryotic and archaebacterial enzymes which maps outside the catalytic domain. Eur J Biochem. 1989 Oct 1;184(3):575–581. doi: 10.1111/j.1432-1033.1989.tb15052.x. [DOI] [PubMed] [Google Scholar]
- Bradley D., Park J. V., Soll L. TRNA2Gln Su+2 mutants that increase amber suppression. J Bacteriol. 1981 Feb;145(2):704–712. doi: 10.1128/jb.145.2.704-712.1981. [DOI] [PMC free article] [PubMed] [Google Scholar]
- Carter C. W., Jr, Coleman D. E. Crystallization of substrate and product analog complexes of tryptophanyl-tRNA synthetase. Fed Proc. 1984 Dec;43(15):2981–2983. [PubMed] [Google Scholar]
- Celis J. E., Coulondre C., Miller J. H. Suppressor su+7 inserts tryptophan in addition to glutamine. J Mol Biol. 1976 Jul 5;104(3):729–734. doi: 10.1016/0022-2836(76)90132-7. [DOI] [PubMed] [Google Scholar]
- Crothers D. M., Seno T., Söll G. Is there a discriminator site in transfer RNA? Proc Natl Acad Sci U S A. 1972 Oct;69(10):3063–3067. doi: 10.1073/pnas.69.10.3063. [DOI] [PMC free article] [PubMed] [Google Scholar]
- DEUTSCHER M. THE EFFECT OF POLYNUCLEOTIDES ON AMINOACYL-RNA SYNTHETASES. 1. INHIBITION BY SYNTHETIC POLYNUCLEOTIDES. Biochem Biophys Res Commun. 1965 Apr 23;19:283–288. doi: 10.1016/0006-291x(65)90455-9. [DOI] [PubMed] [Google Scholar]
- Eggertsson G., Söll D. Transfer ribonucleic acid-mediated suppression of termination codons in Escherichia coli. Microbiol Rev. 1988 Sep;52(3):354–374. doi: 10.1128/mr.52.3.354-374.1988. [DOI] [PMC free article] [PubMed] [Google Scholar]
- Eriani G., Delarue M., Poch O., Gangloff J., Moras D. Partition of tRNA synthetases into two classes based on mutually exclusive sets of sequence motifs. Nature. 1990 Sep 13;347(6289):203–206. doi: 10.1038/347203a0. [DOI] [PubMed] [Google Scholar]
- Francklyn C., Schimmel P. Aminoacylation of RNA minihelices with alanine. Nature. 1989 Feb 2;337(6206):478–481. doi: 10.1038/337478a0. [DOI] [PubMed] [Google Scholar]
- Giegé R., Florentz C., Garcia A., Grosjean H., Perret V., Puglisi J., Théobald-Dietrich A., Ebel J. P. Exploring the aminoacylation function of transfer RNA by macromolecular engineering approaches. Involvement of conformational features in the charging process of yeast tRNA(Asp). Biochimie. 1990 Jun-Jul;72(6-7):453–461. doi: 10.1016/0300-9084(90)90069-s. [DOI] [PubMed] [Google Scholar]
- Hall C. V., vanCleemput M., Muench K. H., Yanofsky C. The nucleotide sequence of the structural gene for Escherichia coli tryptophanyl-tRNA synthetase. J Biol Chem. 1982 Jun 10;257(11):6132–6136. [PubMed] [Google Scholar]
- Hayashi H., Miura K. I. Functional sites in transfer ribonucleic acid. Nature. 1966 Jan 22;209(5021):376–378. doi: 10.1038/209376a0. [DOI] [PubMed] [Google Scholar]
- Himeno H., Hasegawa T., Asahara H., Tamura K., Shimizu M. Identity determinants of E. coli tryptophan tRNA. Nucleic Acids Res. 1991 Dec 11;19(23):6379–6382. doi: 10.1093/nar/19.23.6379. [DOI] [PMC free article] [PubMed] [Google Scholar]
- Hirsh D., Gold L. Translation of the UGA triplet in vitro by tryptophan transfer RNA's. J Mol Biol. 1971 Jun 14;58(2):459–468. doi: 10.1016/0022-2836(71)90363-9. [DOI] [PubMed] [Google Scholar]
- Hirsh D. Tryptophan transfer RNA as the UGA suppressor. J Mol Biol. 1971 Jun 14;58(2):439–458. doi: 10.1016/0022-2836(71)90362-7. [DOI] [PubMed] [Google Scholar]
- Hoben P., Söll D. Glutaminyl-tRNA synthetase of Escherichia coli. Methods Enzymol. 1985;113:55–59. doi: 10.1016/s0076-6879(85)13011-9. [DOI] [PubMed] [Google Scholar]
- Hou Y. M., Schimmel P. A simple structural feature is a major determinant of the identity of a transfer RNA. Nature. 1988 May 12;333(6169):140–145. doi: 10.1038/333140a0. [DOI] [PubMed] [Google Scholar]
- Inokuchi H., Kondo K., Yoshimura M., Ozeki H. Mutant of the glutamine transfer RNA gene as UGA suppressor in Escherichia coli. Mol Gen Genet. 1990 Sep;223(3):433–437. doi: 10.1007/BF00264450. [DOI] [PubMed] [Google Scholar]
- Jahn M., Rogers M. J., Söll D. Anticodon and acceptor stem nucleotides in tRNA(Gln) are major recognition elements for E. coli glutaminyl-tRNA synthetase. Nature. 1991 Jul 18;352(6332):258–260. doi: 10.1038/352258a0. [DOI] [PubMed] [Google Scholar]
- Joseph D. R., Muench K. H. Tryptophanyl transfer ribonucleic acid synthetase of Escherichia coli. I. Purification of the enzyme and of tryptrophan transfer ribonucleic acid. J Biol Chem. 1971 Dec 25;246(24):7602–7609. [PubMed] [Google Scholar]
- Kawakami K., Inada T., Nakamura Y. Conditionally lethal and recessive UGA-suppressor mutations in the prfB gene encoding peptide chain release factor 2 of Escherichia coli. J Bacteriol. 1988 Nov;170(11):5378–5381. doi: 10.1128/jb.170.11.5378-5381.1988. [DOI] [PMC free article] [PubMed] [Google Scholar]
- Kleina L. G., Masson J. M., Normanly J., Abelson J., Miller J. H. Construction of Escherichia coli amber suppressor tRNA genes. II. Synthesis of additional tRNA genes and improvement of suppressor efficiency. J Mol Biol. 1990 Jun 20;213(4):705–717. doi: 10.1016/S0022-2836(05)80257-8. [DOI] [PubMed] [Google Scholar]
- Knowlton R. G., Soll L., Yarus M. Dual specificity of su+ 7 tRNA. Evidence for translational discrimination. J Mol Biol. 1980 Jun 5;139(4):705–720. doi: 10.1016/0022-2836(80)90056-x. [DOI] [PubMed] [Google Scholar]
- Martin N. C., Pham H. D., Underbrink-Lyon K., Miller D. l., Donelson J. E. Yeast mitochondrial tRNATrp can recognize the nonsense codon UGA. Nature. 1980 Jun 19;285(5766):579–581. doi: 10.1038/285579a0. [DOI] [PubMed] [Google Scholar]
- McClain W. H., Foss K. Changing the identity of a tRNA by introducing a G-U wobble pair near the 3' acceptor end. Science. 1988 May 6;240(4853):793–796. doi: 10.1126/science.2452483. [DOI] [PubMed] [Google Scholar]
- McClain W. H., Foss K., Jenkins R. A., Schneider J. Nucleotides that determine Escherichia coli tRNA(Arg) and tRNA(Lys) acceptor identities revealed by analyses of mutant opal and amber suppressor tRNAs. Proc Natl Acad Sci U S A. 1990 Dec;87(23):9260–9264. doi: 10.1073/pnas.87.23.9260. [DOI] [PMC free article] [PubMed] [Google Scholar]
- McClain W. H., Foss K., Jenkins R. A., Schneider J. Rapid determination of nucleotides that define tRNA(Gly) acceptor identity. Proc Natl Acad Sci U S A. 1991 Jul 15;88(14):6147–6151. doi: 10.1073/pnas.88.14.6147. [DOI] [PMC free article] [PubMed] [Google Scholar]
- Miller J. H., Albertini A. M. Effects of surrounding sequence on the suppression of nonsense codons. J Mol Biol. 1983 Feb 15;164(1):59–71. doi: 10.1016/0022-2836(83)90087-6. [DOI] [PubMed] [Google Scholar]
- Murgola E. J. tRNA, suppression, and the code. Annu Rev Genet. 1985;19:57–80. doi: 10.1146/annurev.ge.19.120185.000421. [DOI] [PubMed] [Google Scholar]
- Normanly J., Abelson J. tRNA identity. Annu Rev Biochem. 1989;58:1029–1049. doi: 10.1146/annurev.bi.58.070189.005121. [DOI] [PubMed] [Google Scholar]
- Normanly J., Ogden R. C., Horvath S. J., Abelson J. Changing the identity of a transfer RNA. Nature. 1986 May 15;321(6067):213–219. doi: 10.1038/321213a0. [DOI] [PubMed] [Google Scholar]
- Pallanck L., Schulman L. H. Anticodon-dependent aminoacylation of a noncognate tRNA with isoleucine, valine, and phenylalanine in vivo. Proc Natl Acad Sci U S A. 1991 May 1;88(9):3872–3876. doi: 10.1073/pnas.88.9.3872. [DOI] [PMC free article] [PubMed] [Google Scholar]
- Perona J. J., Swanson R. N., Rould M. A., Steitz T. A., Söll D. Structural basis for misaminoacylation by mutant E. coli glutaminyl-tRNA synthetase enzymes. Science. 1989 Dec 1;246(4934):1152–1154. doi: 10.1126/science.2686030. [DOI] [PubMed] [Google Scholar]
- Rogers M. J., Simmons J., Walker R. T., Weisburg W. G., Woese C. R., Tanner R. S., Robinson I. M., Stahl D. A., Olsen G., Leach R. H. Construction of the mycoplasma evolutionary tree from 5S rRNA sequence data. Proc Natl Acad Sci U S A. 1985 Feb;82(4):1160–1164. doi: 10.1073/pnas.82.4.1160. [DOI] [PMC free article] [PubMed] [Google Scholar]
- Rogers M. J., Söll D. Discrimination between glutaminyl-tRNA synthetase and seryl-tRNA synthetase involves nucleotides in the acceptor helix of tRNA. Proc Natl Acad Sci U S A. 1988 Sep;85(18):6627–6631. doi: 10.1073/pnas.85.18.6627. [DOI] [PMC free article] [PubMed] [Google Scholar]
- Rould M. A., Perona J. J., Steitz T. A. Structural basis of anticodon loop recognition by glutaminyl-tRNA synthetase. Nature. 1991 Jul 18;352(6332):213–218. doi: 10.1038/352213a0. [DOI] [PubMed] [Google Scholar]
- Rould M. A., Perona J. J., Söll D., Steitz T. A. Structure of E. coli glutaminyl-tRNA synthetase complexed with tRNA(Gln) and ATP at 2.8 A resolution. Science. 1989 Dec 1;246(4934):1135–1142. doi: 10.1126/science.2479982. [DOI] [PubMed] [Google Scholar]
- Sampson J. R., Uhlenbeck O. C. Biochemical and physical characterization of an unmodified yeast phenylalanine transfer RNA transcribed in vitro. Proc Natl Acad Sci U S A. 1988 Feb;85(4):1033–1037. doi: 10.1073/pnas.85.4.1033. [DOI] [PMC free article] [PubMed] [Google Scholar]
- Schulman L. H., Pelka H. Anticodon switching changes the identity of methionine and valine transfer RNAs. Science. 1988 Nov 4;242(4879):765–768. doi: 10.1126/science.3055296. [DOI] [PubMed] [Google Scholar]
- Seno T., Agris P. F., Söll D. Involvement of the anticodon region of Escherichia coli tRNAGln and tRNAGlu in the specific interaction with cognate aminoacyl-tRNA synthetase. Alteration of the 2-thiouridine derivatives located in the anticodon of the tRNAs by BrCN or sulfur deprivation. Biochim Biophys Acta. 1974 May 31;349(3):328–338. doi: 10.1016/0005-2787(74)90120-8. [DOI] [PubMed] [Google Scholar]
- Swanson R., Hoben P., Sumner-Smith M., Uemura H., Watson L., Söll D. Accuracy of in vivo aminoacylation requires proper balance of tRNA and aminoacyl-tRNA synthetase. Science. 1988 Dec 16;242(4885):1548–1551. doi: 10.1126/science.3144042. [DOI] [PubMed] [Google Scholar]
- Yamao F., Inokuchi H., Normanly J., Abelson J., Ozeki H. Mischarging mutants of Su+2 glutamine tRNA in E. coli. II. Amino acid specificities of the mutant tRNAs. Jpn J Genet. 1988 Jun;63(3):251–258. doi: 10.1266/jjg.63.251. [DOI] [PubMed] [Google Scholar]
- Yamao F., Inokuchi H., Ozeki H. Mischarging mutants of Su+2 glutamine tRNA in E. coli. I. Mutations near the anticodon cause mischarging. Jpn J Genet. 1988 Jun;63(3):237–249. doi: 10.1266/jjg.63.237. [DOI] [PubMed] [Google Scholar]
- Yamao F., Iwagami S., Azumi Y., Muto A., Osawa S., Fujita N., Ishihama A. Evolutionary dynamics of tryptophan tRNAs in Mycoplasma capricolum. Mol Gen Genet. 1988 May;212(2):364–369. doi: 10.1007/BF00334708. [DOI] [PubMed] [Google Scholar]
- Yaniv M., Folk W. R., Berg P., Soll L. A single mutational modification of a tryptophan-specific transfer RNA permits aminoacylation by glutamine and translation of the codon UAG. J Mol Biol. 1974 Jun 25;86(2):245–260. doi: 10.1016/0022-2836(74)90016-3. [DOI] [PubMed] [Google Scholar]
- Yarus M., Cline S., Raftery L., Wier P., Bradley D. The translational efficiency of tRNA is a property of the anticodon arm. J Biol Chem. 1986 Aug 15;261(23):10496–10505. [PubMed] [Google Scholar]
- ZACHAU H. G. UV-INDUZIERTE URACIL-DIMERISIERUNG IN LOESLICHER RIBONUCLEINSAEURE. Hoppe Seylers Z Physiol Chem. 1964;336:176–188. doi: 10.1515/bchm2.1964.336.1.176. [DOI] [PubMed] [Google Scholar]