Abstract
Protein-folding codes embodied in sequence-dependent energy functions can be optimized using spin-glass theory. Optimal folding codes for associative-memory Hamiltonians based on aligned sequences are deduced. A screening method based on these codes correctly recognizes protein structures in the "twilight zone" of sequence identity in the overwhelming majority of cases. Simulated annealing for the optimally encoded Hamiltonian generally leads to qualitatively correct structures.
Full text
PDF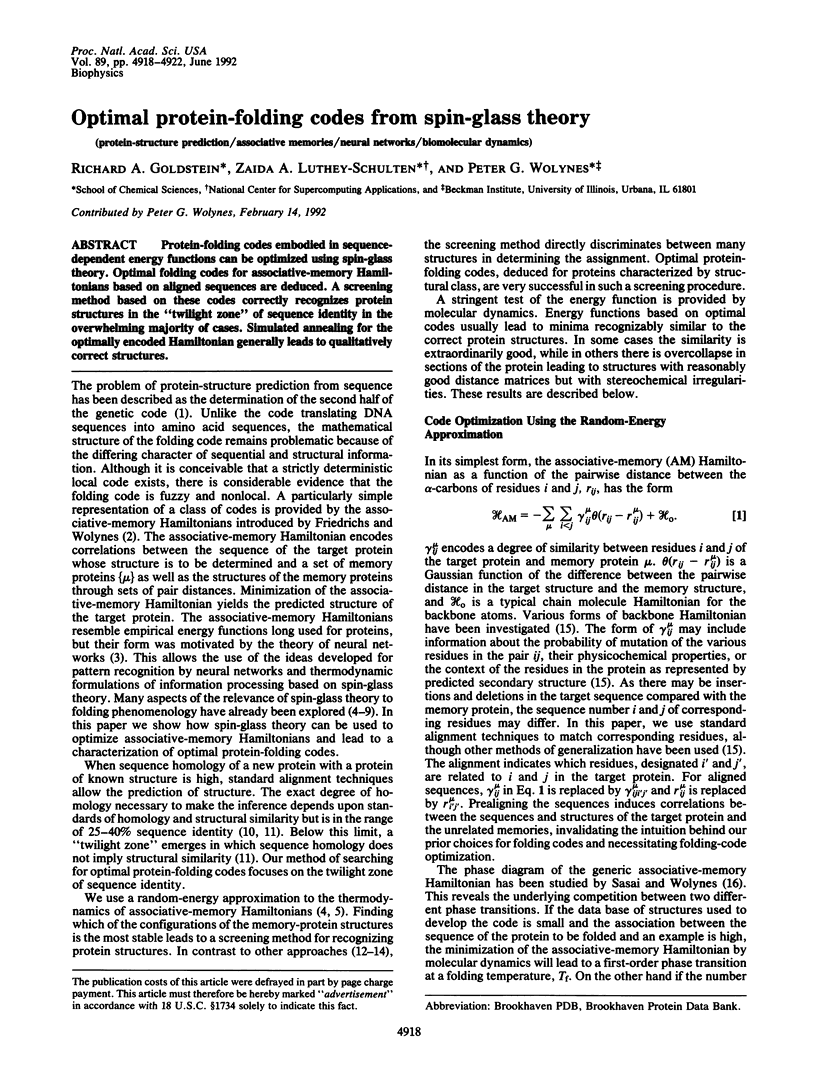
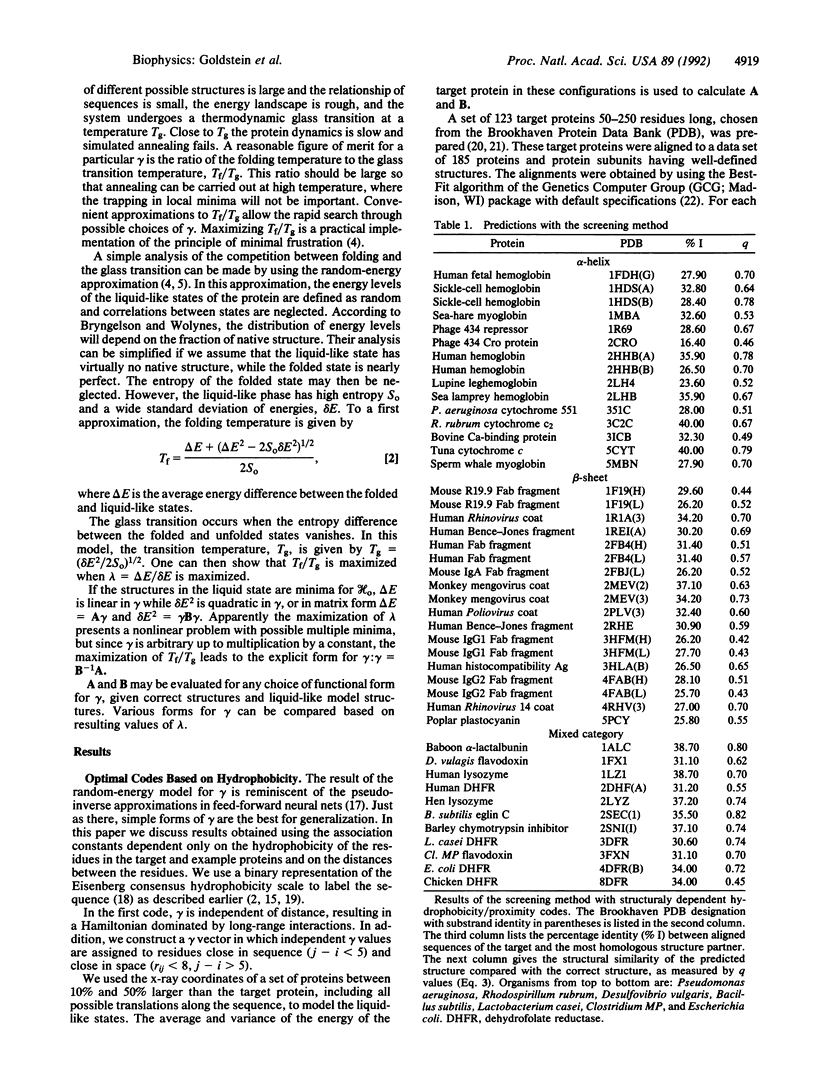
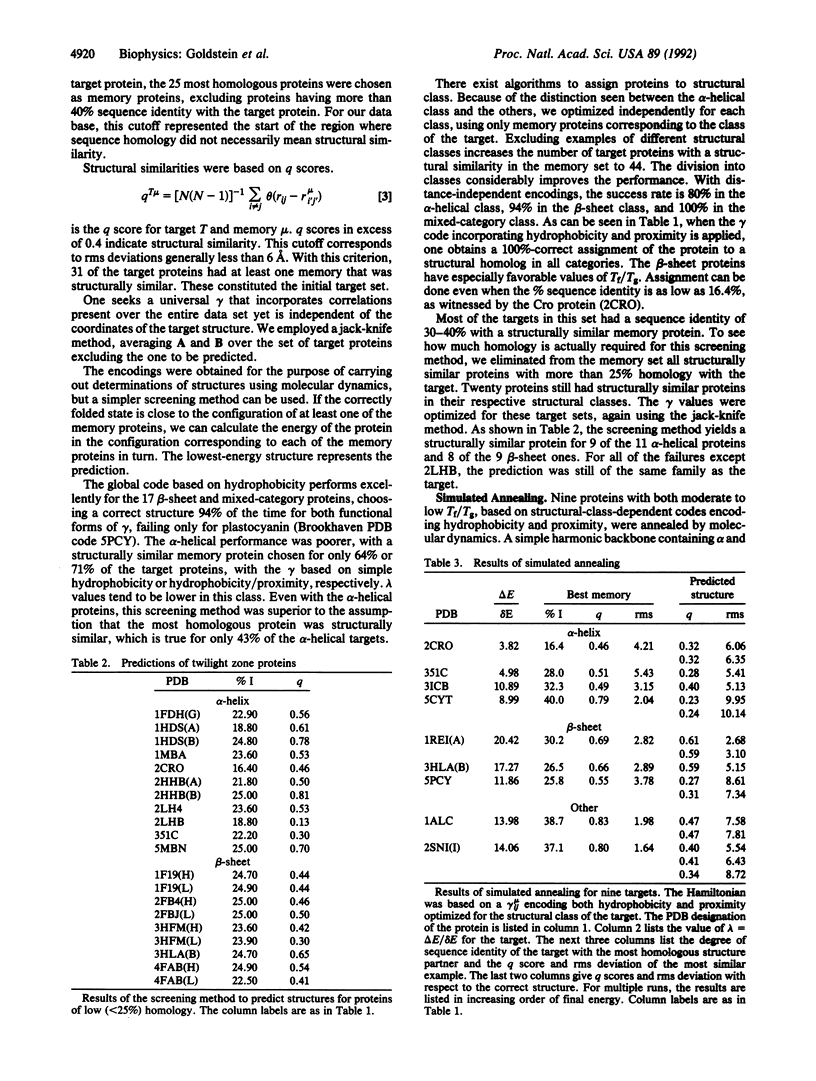
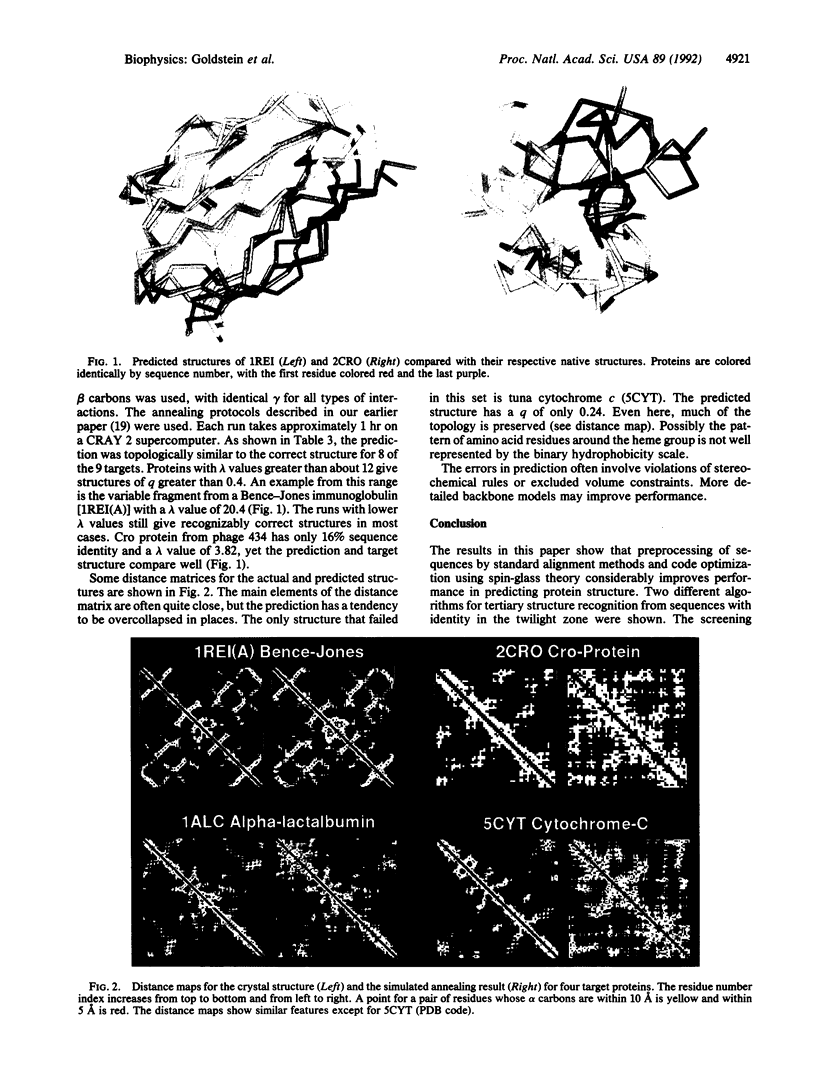
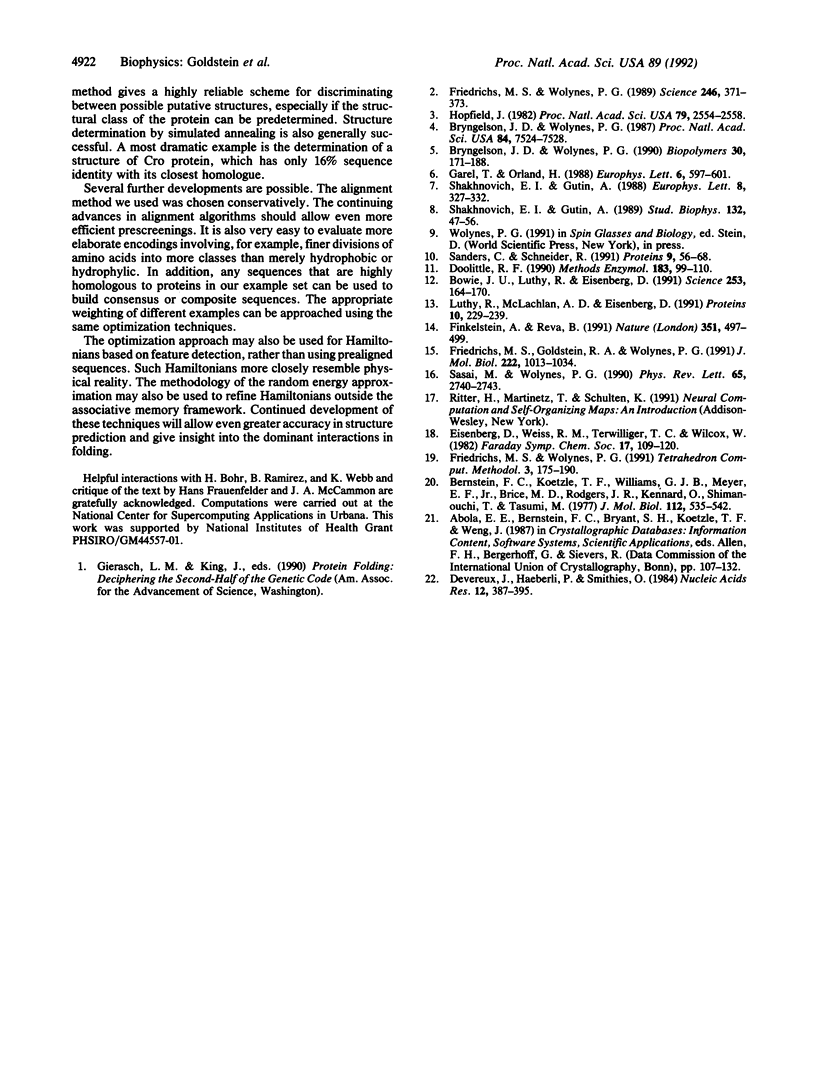
Images in this article
Selected References
These references are in PubMed. This may not be the complete list of references from this article.
- Bernstein F. C., Koetzle T. F., Williams G. J., Meyer E. F., Jr, Brice M. D., Rodgers J. R., Kennard O., Shimanouchi T., Tasumi M. The Protein Data Bank: a computer-based archival file for macromolecular structures. J Mol Biol. 1977 May 25;112(3):535–542. doi: 10.1016/s0022-2836(77)80200-3. [DOI] [PubMed] [Google Scholar]
- Bowie J. U., Lüthy R., Eisenberg D. A method to identify protein sequences that fold into a known three-dimensional structure. Science. 1991 Jul 12;253(5016):164–170. doi: 10.1126/science.1853201. [DOI] [PubMed] [Google Scholar]
- Bryngelson J. D., Wolynes P. G. Spin glasses and the statistical mechanics of protein folding. Proc Natl Acad Sci U S A. 1987 Nov;84(21):7524–7528. doi: 10.1073/pnas.84.21.7524. [DOI] [PMC free article] [PubMed] [Google Scholar]
- Devereux J., Haeberli P., Smithies O. A comprehensive set of sequence analysis programs for the VAX. Nucleic Acids Res. 1984 Jan 11;12(1 Pt 1):387–395. doi: 10.1093/nar/12.1part1.387. [DOI] [PMC free article] [PubMed] [Google Scholar]
- Doolittle R. F. Searching through sequence databases. Methods Enzymol. 1990;183:99–110. doi: 10.1016/0076-6879(90)83008-w. [DOI] [PubMed] [Google Scholar]
- Finkelstein A. V., Reva B. A. A search for the most stable folds of protein chains. Nature. 1991 Jun 6;351(6326):497–499. doi: 10.1038/351497a0. [DOI] [PubMed] [Google Scholar]
- Friedrichs M. S., Goldstein R. A., Wolynes P. G. Generalized protein tertiary structure recognition using associative memory Hamiltonians. J Mol Biol. 1991 Dec 20;222(4):1013–1034. doi: 10.1016/0022-2836(91)90591-s. [DOI] [PubMed] [Google Scholar]
- Friedrichs M. S., Wolynes P. G. Toward protein tertiary structure recognition by means of associative memory hamiltonians. Science. 1989 Oct 20;246(4928):371–373. doi: 10.1126/science.246.4928.371. [DOI] [PubMed] [Google Scholar]
- Hopfield J. J. Neural networks and physical systems with emergent collective computational abilities. Proc Natl Acad Sci U S A. 1982 Apr;79(8):2554–2558. doi: 10.1073/pnas.79.8.2554. [DOI] [PMC free article] [PubMed] [Google Scholar]
- Lüthy R., McLachlan A. D., Eisenberg D. Secondary structure-based profiles: use of structure-conserving scoring tables in searching protein sequence databases for structural similarities. Proteins. 1991;10(3):229–239. doi: 10.1002/prot.340100307. [DOI] [PubMed] [Google Scholar]
- Sander C., Schneider R. Database of homology-derived protein structures and the structural meaning of sequence alignment. Proteins. 1991;9(1):56–68. doi: 10.1002/prot.340090107. [DOI] [PubMed] [Google Scholar]
- Sasai M, Wolynes PG. Molecular theory of associative memory Hamiltonian models of protein folding. Phys Rev Lett. 1990 Nov 19;65(21):2740–2743. doi: 10.1103/PhysRevLett.65.2740. [DOI] [PubMed] [Google Scholar]