Abstract
Although the role of acetylcholine in processing stimuli in the cerebral cortex is becoming defined, the impact of cholinergic activity on the character of cortical maps remains unclear. In the somatosensory cortex, topographic maps appear capable of lifelong modifications in response to alterations in the periphery. One factor proposed to influence this adaptational ability is the presence of acetylcholine in the cortex. The studies presented here, using the 2-deoxyglucose technique, demonstrate that the unilateral removal of a digit in cats, followed by stimulation of an adjacent digit, produces a pattern of metabolic activity in the somatosensory cortex that is dramatically expanded when compared with the opposite (normal) hemisphere. In contrast, experiments in which the somatosensory cortex was depleted of acetylcholine and the animal received a similar amputation led not to patterns of expanded metabolic activity, but rather to reductions in the evoked metabolic distribution. These studies implicate acetylcholine in normal map formation and in the maintenance of the capacity of cortical maps to adapt to changes in the periphery.
Full text
PDF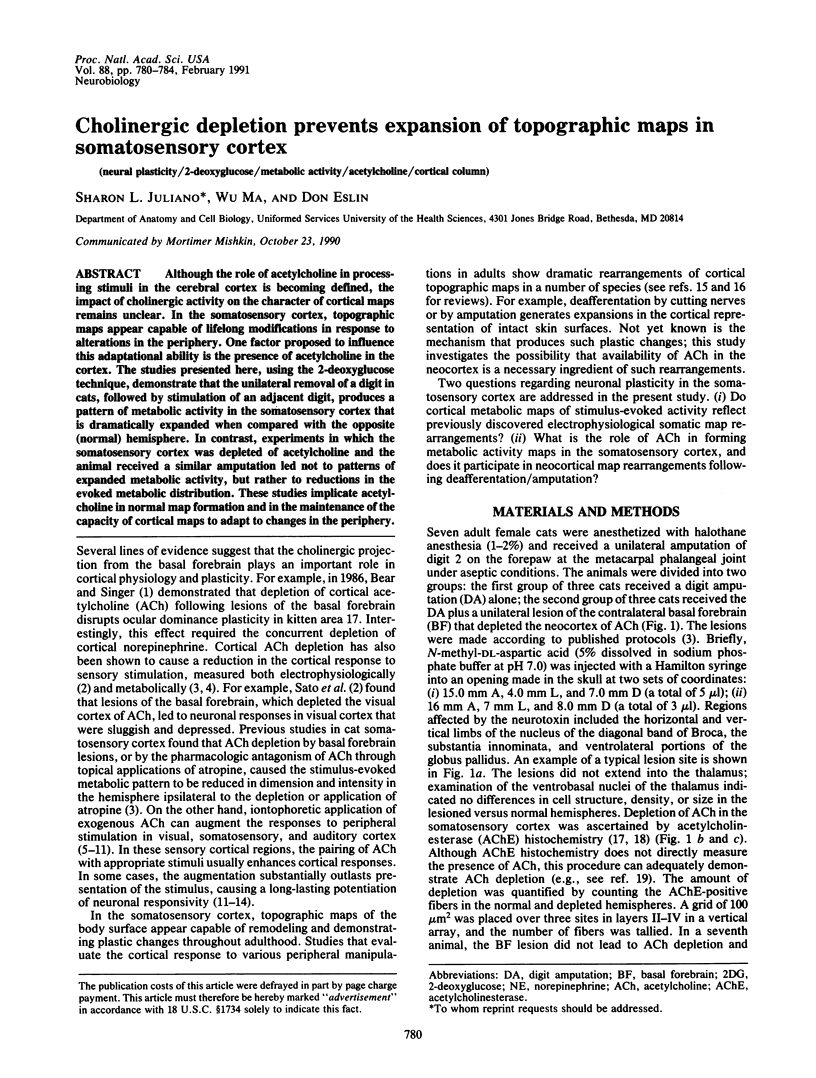
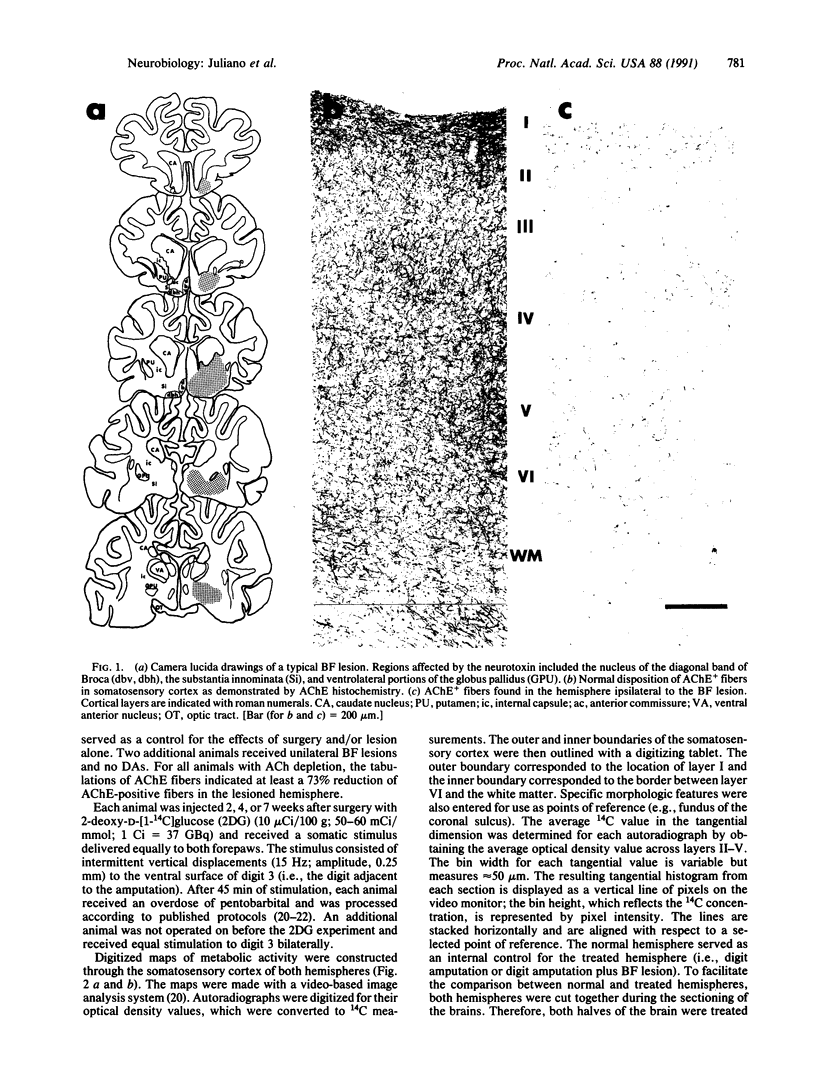
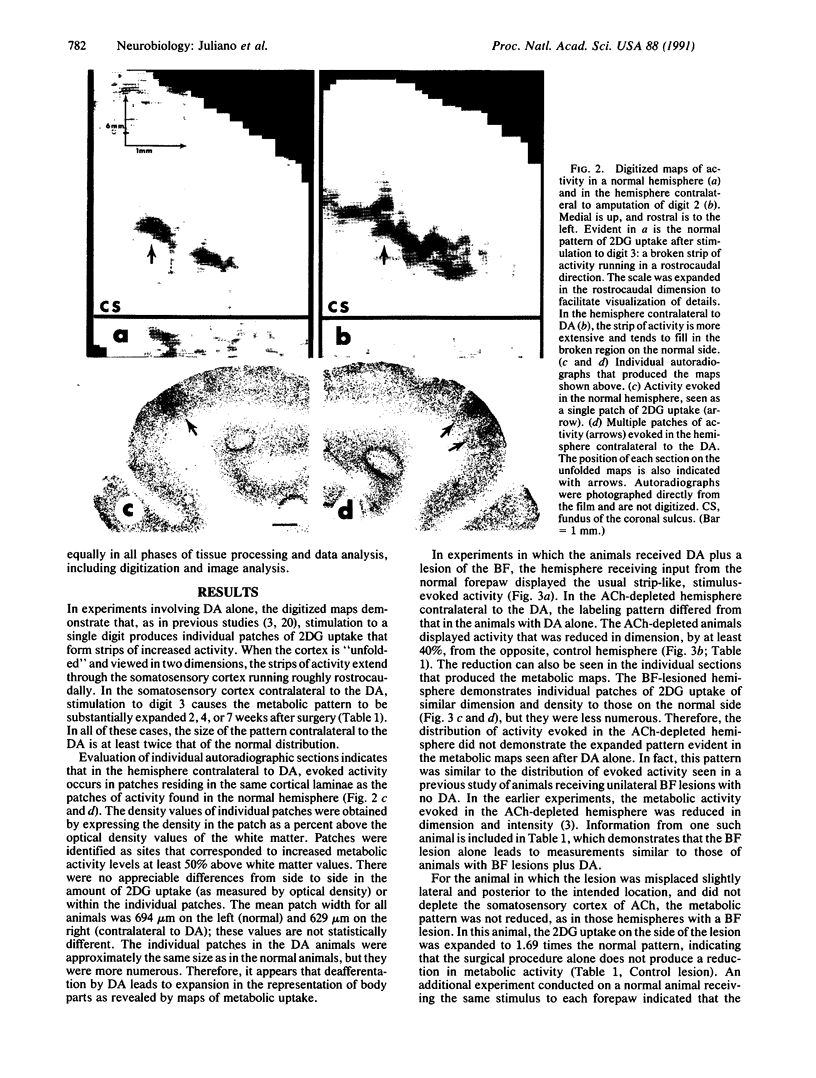
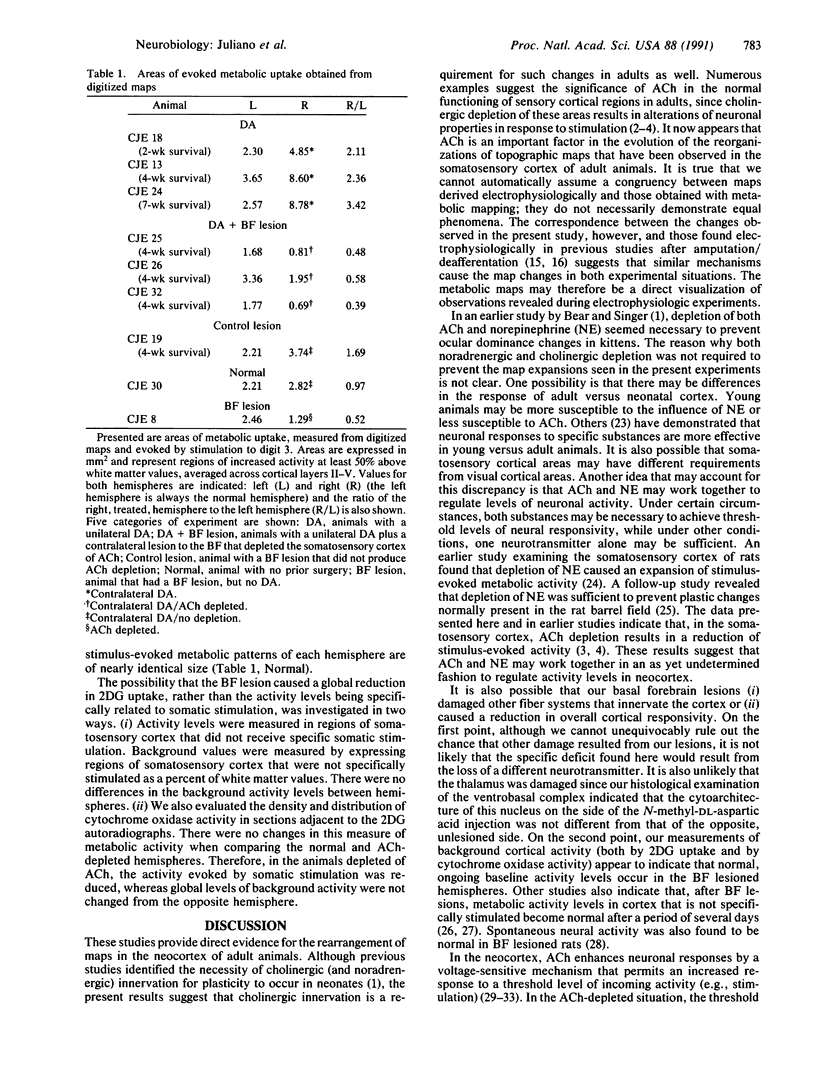
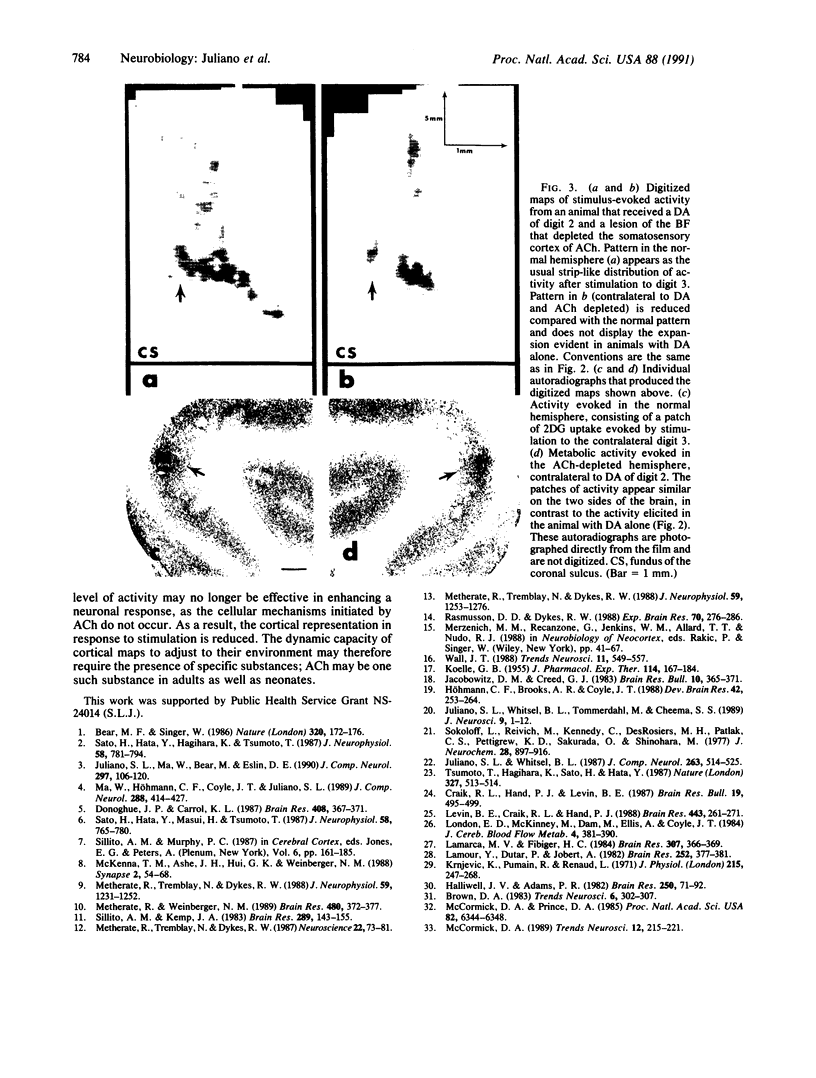
Images in this article
Selected References
These references are in PubMed. This may not be the complete list of references from this article.
- Bear M. F., Singer W. Modulation of visual cortical plasticity by acetylcholine and noradrenaline. Nature. 1986 Mar 13;320(6058):172–176. doi: 10.1038/320172a0. [DOI] [PubMed] [Google Scholar]
- Craik R. L., Hand P. J., Levin B. E. Locus coeruleus input affects glucose metabolism in activated rat barrel cortex. Brain Res Bull. 1987 Oct;19(4):495–499. doi: 10.1016/0361-9230(87)90154-7. [DOI] [PubMed] [Google Scholar]
- Donoghue J. P., Carroll K. L. Cholinergic modulation of sensory responses in rat primary somatic sensory cortex. Brain Res. 1987 Apr 7;408(1-2):367–371. doi: 10.1016/0006-8993(87)90407-0. [DOI] [PubMed] [Google Scholar]
- Halliwell J. V., Adams P. R. Voltage-clamp analysis of muscarinic excitation in hippocampal neurons. Brain Res. 1982 Oct 28;250(1):71–92. doi: 10.1016/0006-8993(82)90954-4. [DOI] [PubMed] [Google Scholar]
- Hohmann C. F., Brooks A. R., Coyle J. T. Neonatal lesions of the basal forebrain cholinergic neurons result in abnormal cortical development. Brain Res. 1988 Aug 1;470(2):253–264. doi: 10.1016/0165-3806(88)90244-1. [DOI] [PubMed] [Google Scholar]
- Jacobowitz D. M., Creed G. J. Cholinergic projection sites of the nucleus of tractus diagonalis. Brain Res Bull. 1983 Mar;10(3):365–371. doi: 10.1016/0361-9230(83)90105-3. [DOI] [PubMed] [Google Scholar]
- Juliano S. L., Ma W., Bear M. F., Eslin D. Cholinergic manipulation alters stimulus-evoked metabolic activity in cat somatosensory cortex. J Comp Neurol. 1990 Jul 1;297(1):106–120. doi: 10.1002/cne.902970108. [DOI] [PubMed] [Google Scholar]
- Juliano S. L., Whitsel B. L. A combined 2-deoxyglucose and neurophysiological study of primate somatosensory cortex. J Comp Neurol. 1987 Sep 22;263(4):514–525. doi: 10.1002/cne.902630405. [DOI] [PubMed] [Google Scholar]
- Juliano S. L., Whitsel B. L., Tommerdahl M., Cheema S. S. Determinants of patchy metabolic labeling in the somatosensory cortex of cats: a possible role for intrinsic inhibitory circuitry. J Neurosci. 1989 Jan;9(1):1–12. doi: 10.1523/JNEUROSCI.09-01-00001.1989. [DOI] [PMC free article] [PubMed] [Google Scholar]
- KOELLE G. B. The histochemical identification of acetylcholinesterase in cholinergic, adrenergic and sensory neurons. J Pharmacol Exp Ther. 1955 Jun;114(2):167–184. [PubMed] [Google Scholar]
- Krnjević K., Pumain R., Renaud L. The mechanism of excitation by acetylcholine in the cerebral cortex. J Physiol. 1971 May;215(1):247–268. doi: 10.1113/jphysiol.1971.sp009467. [DOI] [PMC free article] [PubMed] [Google Scholar]
- Lamarca M. V., Fibiger H. C. Deoxyglucose uptake and choline acetyltransferase activity in cerebral cortex following lesions of the nucleus basalis magnocellularis. Brain Res. 1984 Jul 30;307(1-2):366–369. doi: 10.1016/0006-8993(84)90497-9. [DOI] [PubMed] [Google Scholar]
- Lamour Y., Dutar P., Jobert A. Spread of acetylcholine sensitivity in the neocortex following lesion of the nucleus basalis. Brain Res. 1982 Dec 9;252(2):377–381. doi: 10.1016/0006-8993(82)90406-1. [DOI] [PubMed] [Google Scholar]
- Levin B. E., Craik R. L., Hand P. J. The role of norepinephrine in adult rat somatosensory (SmI) cortical metabolism and plasticity. Brain Res. 1988 Mar 8;443(1-2):261–271. doi: 10.1016/0006-8993(88)91620-4. [DOI] [PubMed] [Google Scholar]
- London E. D., McKinney M., Dam M., Ellis A., Coyle J. T. Decreased cortical glucose utilization after ibotenate lesion of the rat ventromedial globus pallidus. J Cereb Blood Flow Metab. 1984 Sep;4(3):381–390. doi: 10.1038/jcbfm.1984.56. [DOI] [PubMed] [Google Scholar]
- Ma W., Höhmann C. F., Coyle J. T., Juliano S. L. Lesions of the basal forebrain alter stimulus-evoked metabolic activity in mouse somatosensory cortex. J Comp Neurol. 1989 Oct 15;288(3):414–427. doi: 10.1002/cne.902880305. [DOI] [PubMed] [Google Scholar]
- McCormick D. A. Cholinergic and noradrenergic modulation of thalamocortical processing. Trends Neurosci. 1989 Jun;12(6):215–221. doi: 10.1016/0166-2236(89)90125-2. [DOI] [PubMed] [Google Scholar]
- McCormick D. A., Prince D. A. Two types of muscarinic response to acetylcholine in mammalian cortical neurons. Proc Natl Acad Sci U S A. 1985 Sep;82(18):6344–6348. doi: 10.1073/pnas.82.18.6344. [DOI] [PMC free article] [PubMed] [Google Scholar]
- McKenna T. M., Ashe J. H., Hui G. K., Weinberger N. M. Muscarinic agonists modulate spontaneous and evoked unit discharge in auditory cortex of cat. Synapse. 1988;2(1):54–68. doi: 10.1002/syn.890020109. [DOI] [PubMed] [Google Scholar]
- Metherate R., Tremblay N., Dykes R. W. Acetylcholine permits long-term enhancement of neuronal responsiveness in cat primary somatosensory cortex. Neuroscience. 1987 Jul;22(1):75–81. doi: 10.1016/0306-4522(87)90198-9. [DOI] [PubMed] [Google Scholar]
- Metherate R., Tremblay N., Dykes R. W. The effects of acetylcholine on response properties of cat somatosensory cortical neurons. J Neurophysiol. 1988 Apr;59(4):1231–1252. doi: 10.1152/jn.1988.59.4.1231. [DOI] [PubMed] [Google Scholar]
- Metherate R., Tremblay N., Dykes R. W. Transient and prolonged effects of acetylcholine on responsiveness of cat somatosensory cortical neurons. J Neurophysiol. 1988 Apr;59(4):1253–1276. doi: 10.1152/jn.1988.59.4.1253. [DOI] [PubMed] [Google Scholar]
- Metherate R., Weinberger N. M. Acetylcholine produces stimulus-specific receptive field alterations in cat auditory cortex. Brain Res. 1989 Feb 20;480(1-2):372–377. doi: 10.1016/0006-8993(89)90210-2. [DOI] [PubMed] [Google Scholar]
- Rasmusson D. D., Dykes R. W. Long-term enhancement of evoked potentials in cat somatosensory cortex produced by co-activation of the basal forebrain and cutaneous receptors. Exp Brain Res. 1988;70(2):276–286. doi: 10.1007/BF00248353. [DOI] [PubMed] [Google Scholar]
- Sato H., Hata Y., Hagihara K., Tsumoto T. Effects of cholinergic depletion on neuron activities in the cat visual cortex. J Neurophysiol. 1987 Oct;58(4):781–794. doi: 10.1152/jn.1987.58.4.781. [DOI] [PubMed] [Google Scholar]
- Sato H., Hata Y., Masui H., Tsumoto T. A functional role of cholinergic innervation to neurons in the cat visual cortex. J Neurophysiol. 1987 Oct;58(4):765–780. doi: 10.1152/jn.1987.58.4.765. [DOI] [PubMed] [Google Scholar]
- Sillito A. M., Kemp J. A. Cholinergic modulation of the functional organization of the cat visual cortex. Brain Res. 1983 Dec 19;289(1-2):143–155. doi: 10.1016/0006-8993(83)90015-x. [DOI] [PubMed] [Google Scholar]
- Sokoloff L., Reivich M., Kennedy C., Des Rosiers M. H., Patlak C. S., Pettigrew K. D., Sakurada O., Shinohara M. The [14C]deoxyglucose method for the measurement of local cerebral glucose utilization: theory, procedure, and normal values in the conscious and anesthetized albino rat. J Neurochem. 1977 May;28(5):897–916. doi: 10.1111/j.1471-4159.1977.tb10649.x. [DOI] [PubMed] [Google Scholar]
- Tsumoto T., Hagihara K., Sato H., Hata Y. NMDA receptors in the visual cortex of young kittens are more effective than those of adult cats. Nature. 1987 Jun 11;327(6122):513–514. doi: 10.1038/327513a0. [DOI] [PubMed] [Google Scholar]
- Wall J. T. Variable organization in cortical maps of the skin as an indication of the lifelong adaptive capacities of circuits in the mammalian brain. Trends Neurosci. 1988 Dec;11(12):549–557. doi: 10.1016/0166-2236(88)90184-1. [DOI] [PubMed] [Google Scholar]