Abstract
To test whether interactions in the hydrophobic core of a protein can be adequately modeled based on the properties of a liquid hydrocarbon, we measured the unfolding free energies of the wild-type bacteriophage f1 gene V protein and 29 mutants with apolar substitutions at positions 35 and 47. Stability changes arising from identical mutations at these two buried sites are quite different, suggesting that one site is more rigid than the other. Reversals of residues at positions 35 and 47 confirm that their environments are distinct. Mutants containing weakly polar residues at these two sites suggest that the protein interior is more polar than a liquid hydrocarbon. Interactions between residues at the two sites appear to be minimal. These observations are compatible with a view of protein interiors that incorporates properties of liquid hydrocarbons but also includes polar interactions and a site-dependent "packing energy" associated with changes in internal structure.
Full text
PDF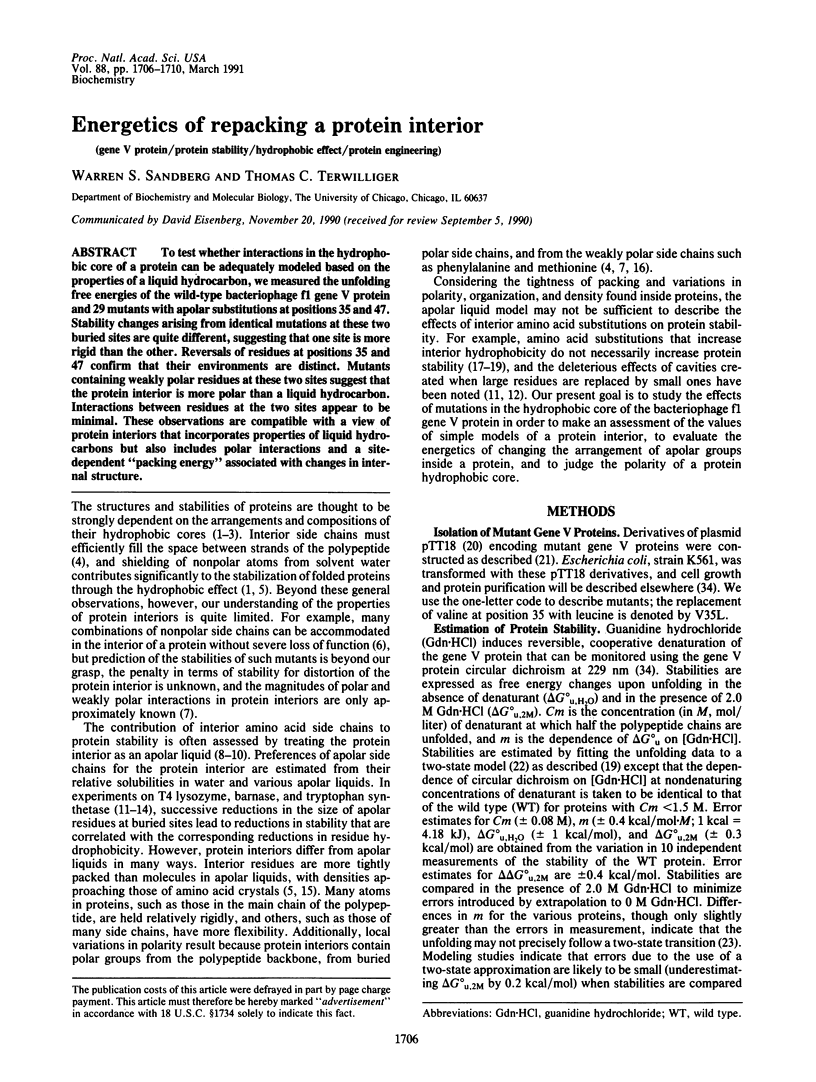
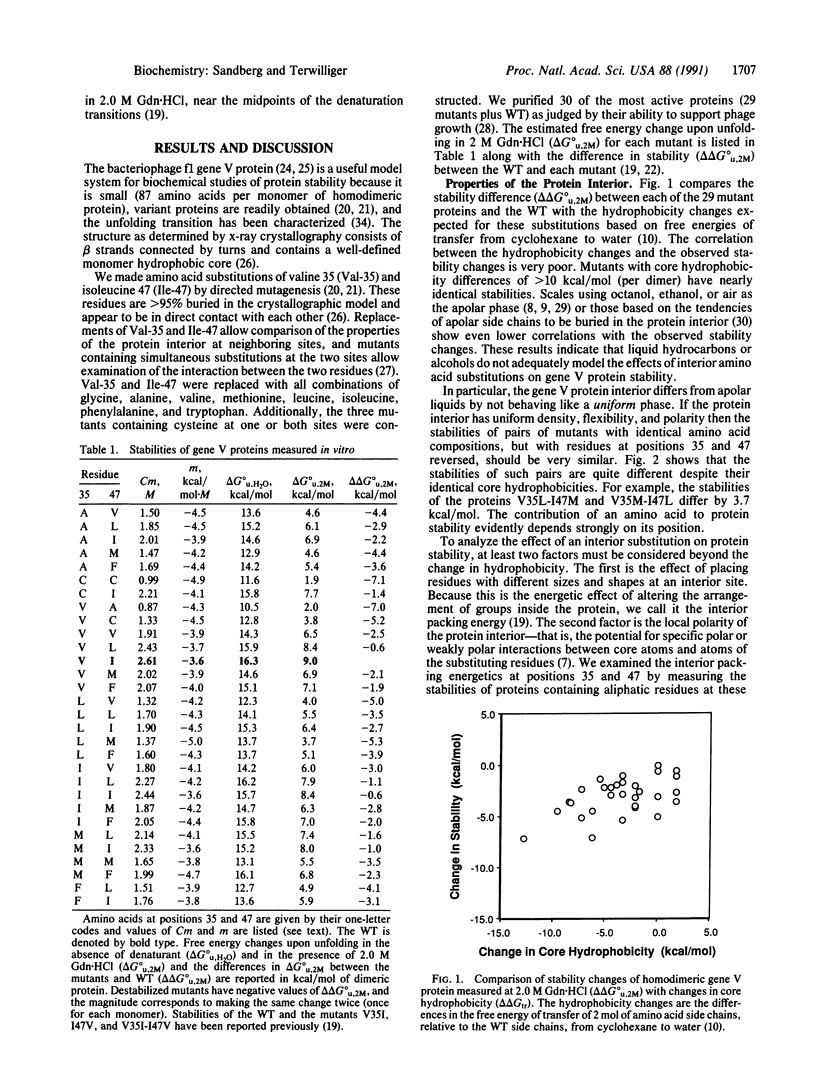
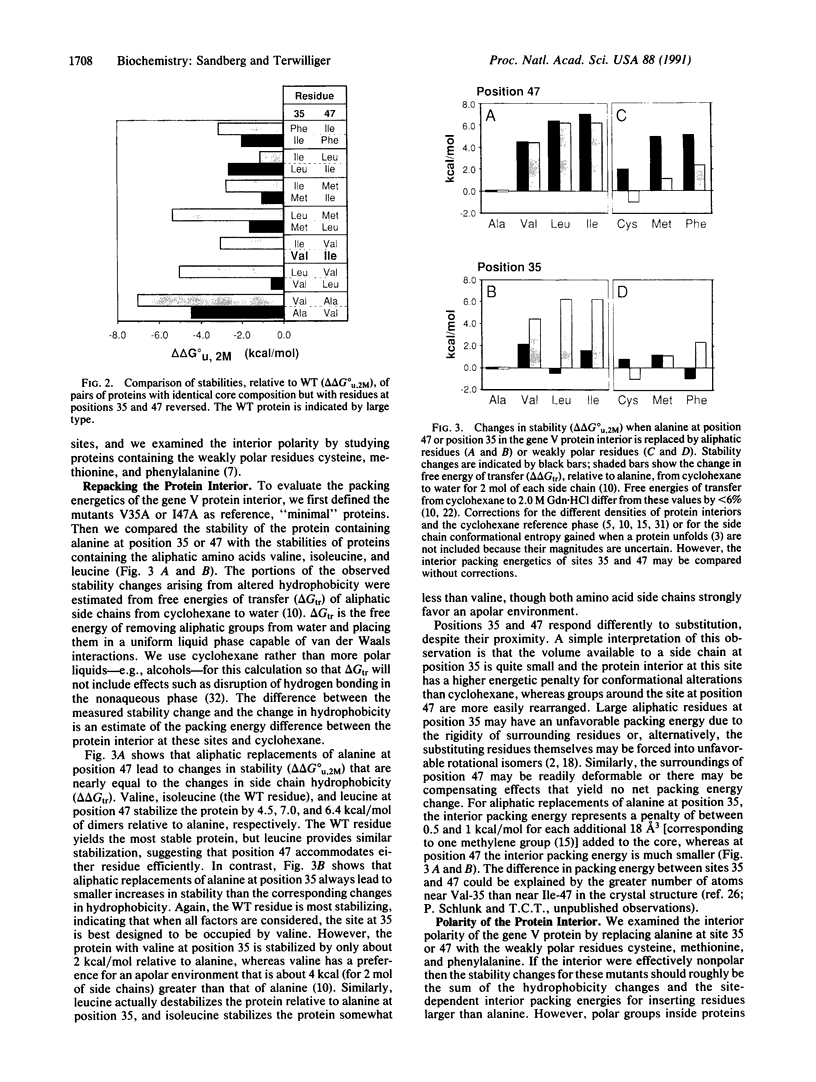
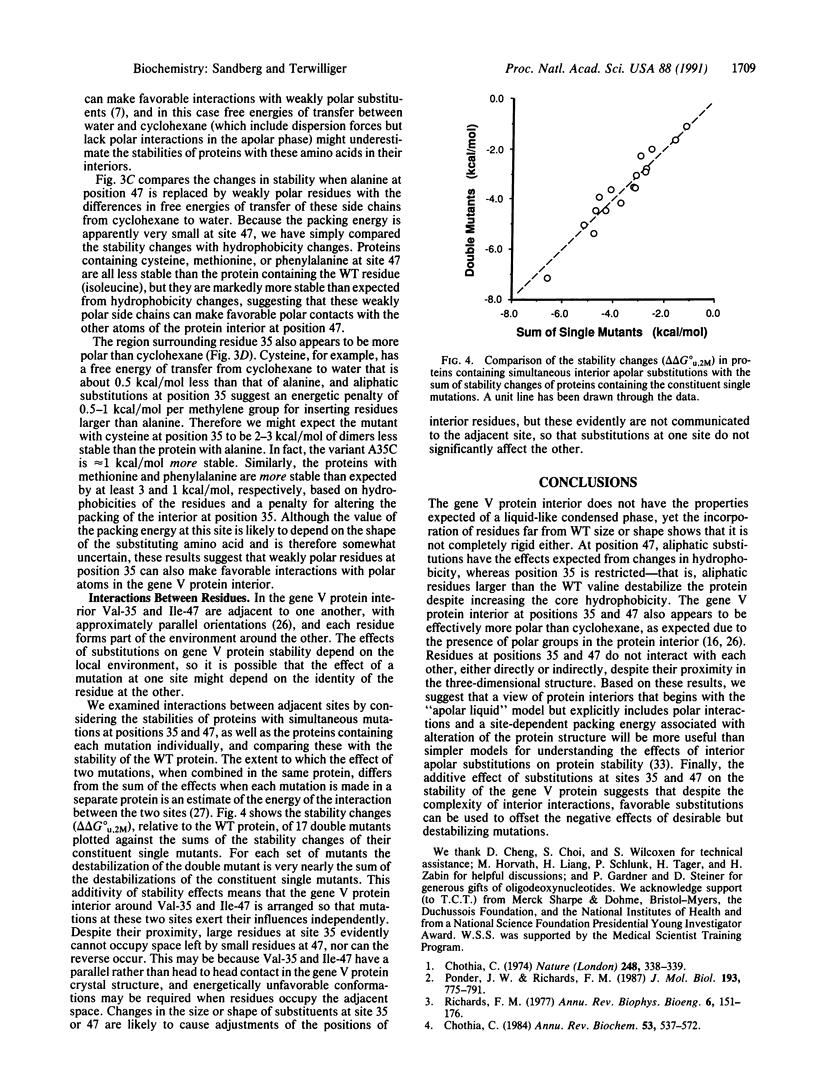
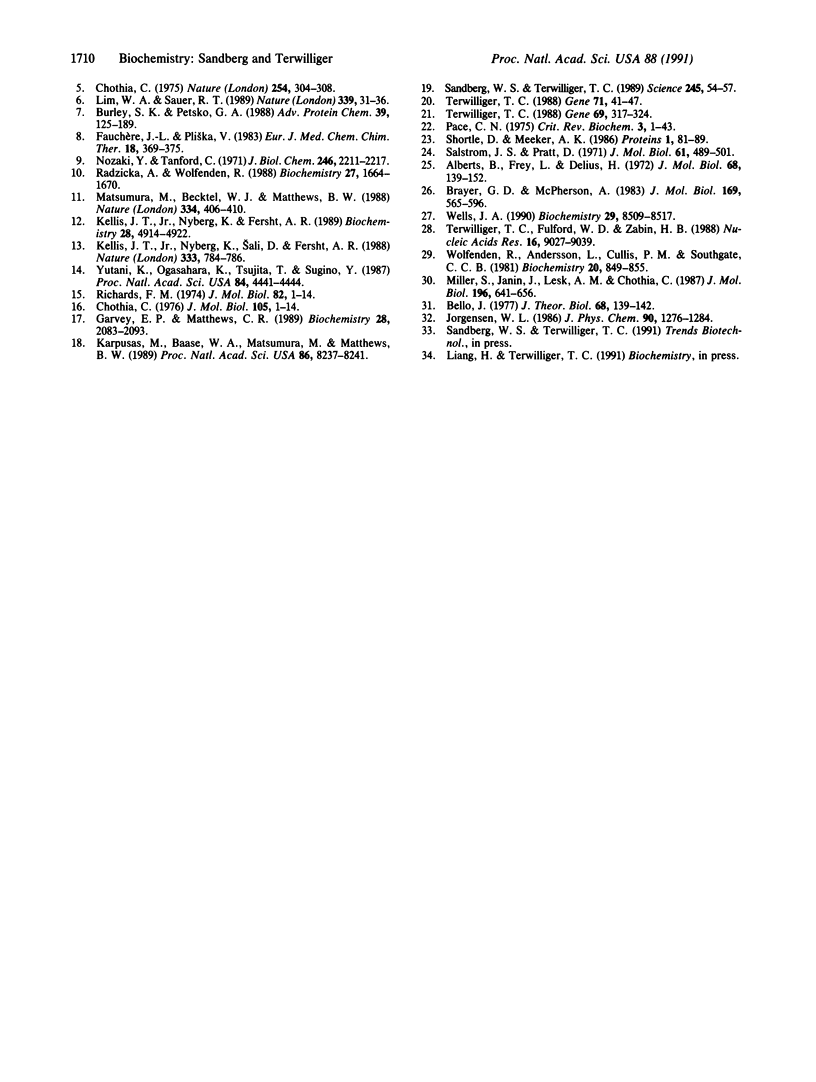
Images in this article
Selected References
These references are in PubMed. This may not be the complete list of references from this article.
- Alberts B., Frey L., Delius H. Isolation and characterization of gene 5 protein of filamentous bacterial viruses. J Mol Biol. 1972 Jul 14;68(1):139–152. doi: 10.1016/0022-2836(72)90269-0. [DOI] [PubMed] [Google Scholar]
- Bello J. Stability of protein conformation: internal packing and enthalpy of fusion of model compounds. J Theor Biol. 1977 Sep 7;68(1):139–142. doi: 10.1016/0022-5193(77)90232-6. [DOI] [PubMed] [Google Scholar]
- Burley S. K., Petsko G. A. Weakly polar interactions in proteins. Adv Protein Chem. 1988;39:125–189. doi: 10.1016/s0065-3233(08)60376-9. [DOI] [PubMed] [Google Scholar]
- Chothia C. Hydrophobic bonding and accessible surface area in proteins. Nature. 1974 Mar 22;248(446):338–339. doi: 10.1038/248338a0. [DOI] [PubMed] [Google Scholar]
- Chothia C. Principles that determine the structure of proteins. Annu Rev Biochem. 1984;53:537–572. doi: 10.1146/annurev.bi.53.070184.002541. [DOI] [PubMed] [Google Scholar]
- Chothia C. Structural invariants in protein folding. Nature. 1975 Mar 27;254(5498):304–308. doi: 10.1038/254304a0. [DOI] [PubMed] [Google Scholar]
- Chothia C. The nature of the accessible and buried surfaces in proteins. J Mol Biol. 1976 Jul 25;105(1):1–12. doi: 10.1016/0022-2836(76)90191-1. [DOI] [PubMed] [Google Scholar]
- Garvey E. P., Matthews C. R. Effects of multiple replacements at a single position on the folding and stability of dihydrofolate reductase from Escherichia coli. Biochemistry. 1989 Mar 7;28(5):2083–2093. doi: 10.1021/bi00431a018. [DOI] [PubMed] [Google Scholar]
- Karpusas M., Baase W. A., Matsumura M., Matthews B. W. Hydrophobic packing in T4 lysozyme probed by cavity-filling mutants. Proc Natl Acad Sci U S A. 1989 Nov;86(21):8237–8241. doi: 10.1073/pnas.86.21.8237. [DOI] [PMC free article] [PubMed] [Google Scholar]
- Kellis J. T., Jr, Nyberg K., Fersht A. R. Energetics of complementary side-chain packing in a protein hydrophobic core. Biochemistry. 1989 May 30;28(11):4914–4922. doi: 10.1021/bi00437a058. [DOI] [PubMed] [Google Scholar]
- Kellis J. T., Jr, Nyberg K., Sali D., Fersht A. R. Contribution of hydrophobic interactions to protein stability. Nature. 1988 Jun 23;333(6175):784–786. doi: 10.1038/333784a0. [DOI] [PubMed] [Google Scholar]
- Lim W. A., Sauer R. T. Alternative packing arrangements in the hydrophobic core of lambda repressor. Nature. 1989 May 4;339(6219):31–36. doi: 10.1038/339031a0. [DOI] [PubMed] [Google Scholar]
- Matsumura M., Becktel W. J., Matthews B. W. Hydrophobic stabilization in T4 lysozyme determined directly by multiple substitutions of Ile 3. Nature. 1988 Aug 4;334(6181):406–410. doi: 10.1038/334406a0. [DOI] [PubMed] [Google Scholar]
- Miller S., Janin J., Lesk A. M., Chothia C. Interior and surface of monomeric proteins. J Mol Biol. 1987 Aug 5;196(3):641–656. doi: 10.1016/0022-2836(87)90038-6. [DOI] [PubMed] [Google Scholar]
- Nozaki Y., Tanford C. The solubility of amino acids and two glycine peptides in aqueous ethanol and dioxane solutions. Establishment of a hydrophobicity scale. J Biol Chem. 1971 Apr 10;246(7):2211–2217. [PubMed] [Google Scholar]
- Pace C. N. The stability of globular proteins. CRC Crit Rev Biochem. 1975 May;3(1):1–43. doi: 10.3109/10409237509102551. [DOI] [PubMed] [Google Scholar]
- Ponder J. W., Richards F. M. Tertiary templates for proteins. Use of packing criteria in the enumeration of allowed sequences for different structural classes. J Mol Biol. 1987 Feb 20;193(4):775–791. doi: 10.1016/0022-2836(87)90358-5. [DOI] [PubMed] [Google Scholar]
- Richards F. M. Areas, volumes, packing and protein structure. Annu Rev Biophys Bioeng. 1977;6:151–176. doi: 10.1146/annurev.bb.06.060177.001055. [DOI] [PubMed] [Google Scholar]
- Richards F. M. The interpretation of protein structures: total volume, group volume distributions and packing density. J Mol Biol. 1974 Jan 5;82(1):1–14. doi: 10.1016/0022-2836(74)90570-1. [DOI] [PubMed] [Google Scholar]
- Salstrom J. S., Pratt D. Role of coliphage M13 gene 5 in single-stranded DNA production. J Mol Biol. 1971 Nov 14;61(3):489–501. doi: 10.1016/0022-2836(71)90061-1. [DOI] [PubMed] [Google Scholar]
- Sandberg W. S., Terwilliger T. C. Influence of interior packing and hydrophobicity on the stability of a protein. Science. 1989 Jul 7;245(4913):54–57. doi: 10.1126/science.2787053. [DOI] [PubMed] [Google Scholar]
- Shortle D., Meeker A. K. Mutant forms of staphylococcal nuclease with altered patterns of guanidine hydrochloride and urea denaturation. Proteins. 1986 Sep;1(1):81–89. doi: 10.1002/prot.340010113. [DOI] [PubMed] [Google Scholar]
- Terwilliger T. C. Construction of a synthetic variant of the bacteriophage f1 gene V by assembling oligodeoxynucleotides corresponding to only one strand of DNA. Gene. 1988 Nov 15;71(1):41–47. doi: 10.1016/0378-1119(88)90075-3. [DOI] [PubMed] [Google Scholar]
- Terwilliger T. C., Fulford W. D., Zabin H. B. A genetic selection for temperature-sensitive variants of the gene V protein of bacteriophage f1. Nucleic Acids Res. 1988 Sep 26;16(18):9027–9039. doi: 10.1093/nar/16.18.9027. [DOI] [PMC free article] [PubMed] [Google Scholar]
- Terwilliger T. C. Simple and highly efficient site-specific mutagenesis, by ligation of an oligodeoxyribonucleotide into gapped heteroduplex DNA in which the template strand contains deoxyuridine. Gene. 1988 Sep 30;69(2):317–324. doi: 10.1016/0378-1119(88)90441-6. [DOI] [PubMed] [Google Scholar]
- Wells J. A. Additivity of mutational effects in proteins. Biochemistry. 1990 Sep 18;29(37):8509–8517. doi: 10.1021/bi00489a001. [DOI] [PubMed] [Google Scholar]
- Wolfenden R., Andersson L., Cullis P. M., Southgate C. C. Affinities of amino acid side chains for solvent water. Biochemistry. 1981 Feb 17;20(4):849–855. doi: 10.1021/bi00507a030. [DOI] [PubMed] [Google Scholar]
- Yutani K., Ogasahara K., Tsujita T., Sugino Y. Dependence of conformational stability on hydrophobicity of the amino acid residue in a series of variant proteins substituted at a unique position of tryptophan synthase alpha subunit. Proc Natl Acad Sci U S A. 1987 Jul;84(13):4441–4444. doi: 10.1073/pnas.84.13.4441. [DOI] [PMC free article] [PubMed] [Google Scholar]