Abstract
The charge-stabilization effect associated with alpha-helices in proteins has been reexamined by microscopic calculations without any a priori assumptions about the dielectric constant of the protein. The calculations reproduce the observed charge stabilization effect of a helix in two well-defined test cases: a histidine residue situated at the C-terminal end of a helix in barnase and the sulfate ligand located near the N-terminal end of a helix of the sulfate-binding protein. They also show that the effective dielectric constant for helix-charge interactions is much larger than previously assumed and that the stabilizing effect of the helix is not associated with the helix macrodipole but rather with a few localized dipoles confined mostly to the first turn of the helix. It is predicted that mutations at one end of the helix should have very small effects on the stabilization of charges at the opposite terminus. It is pointed out that the relatively short-ranged effect of the helix is essentially similar to other cases in which localized dipoles play key roles in electrostatic stabilization.
Full text
PDF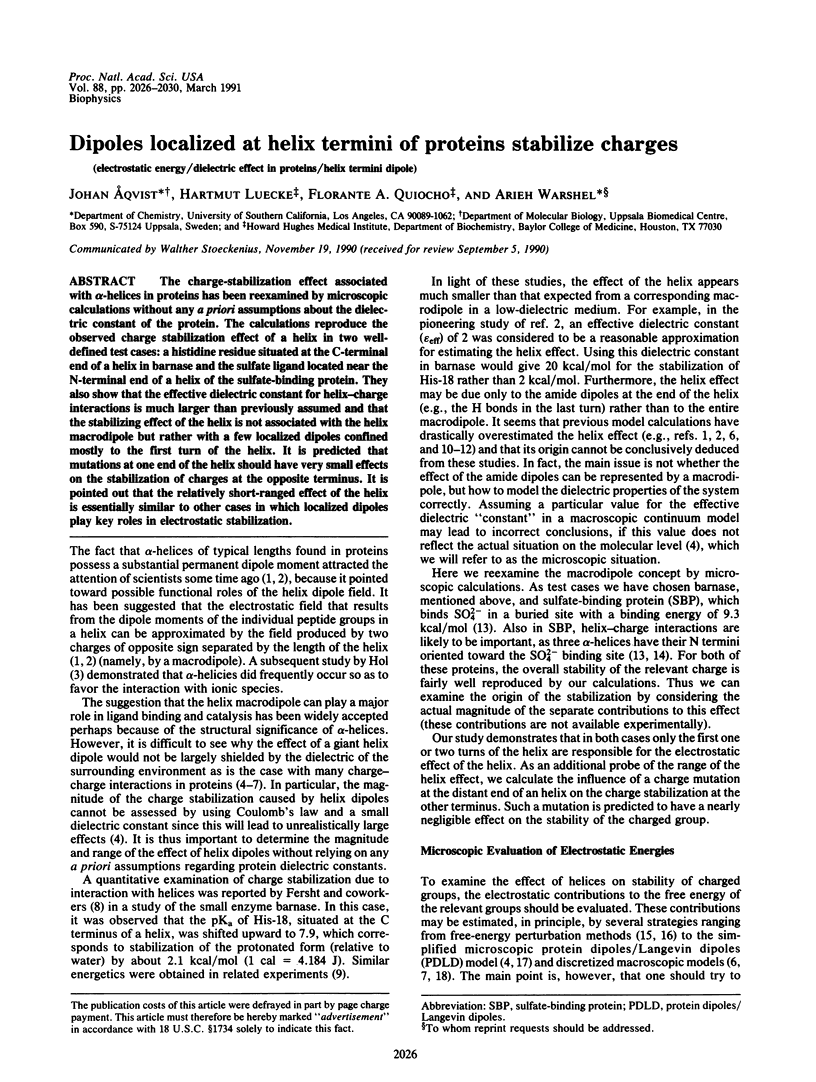
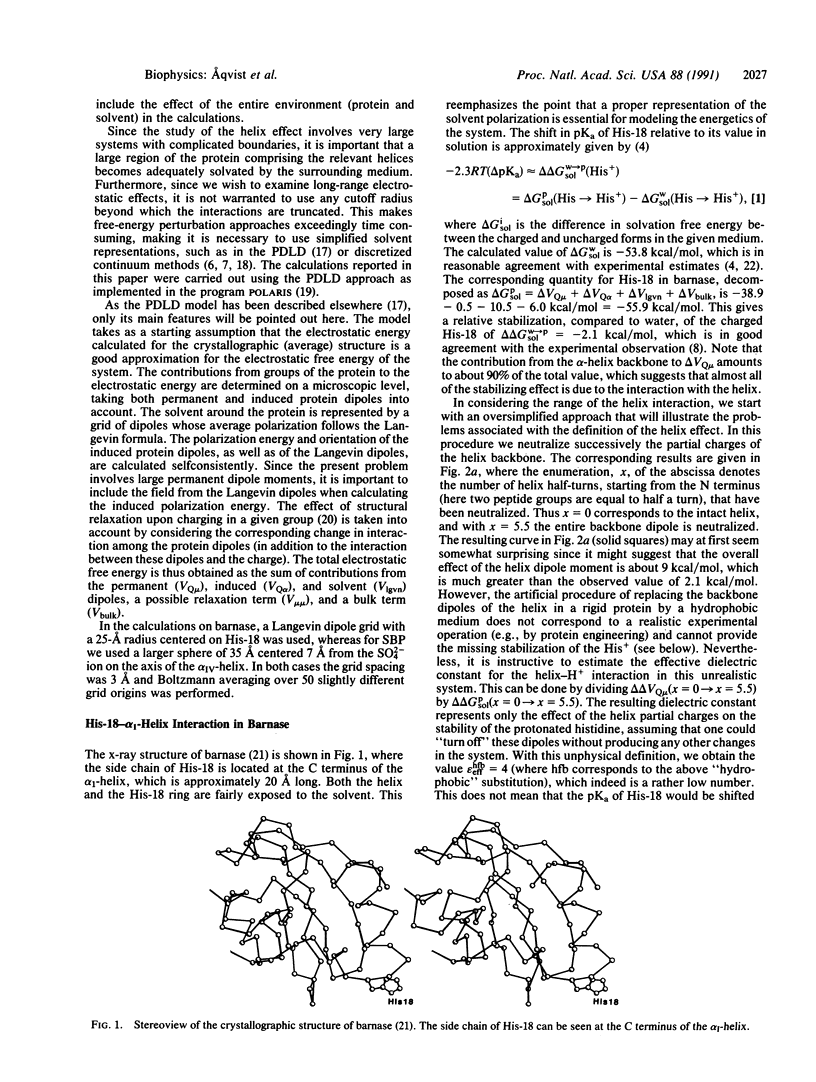
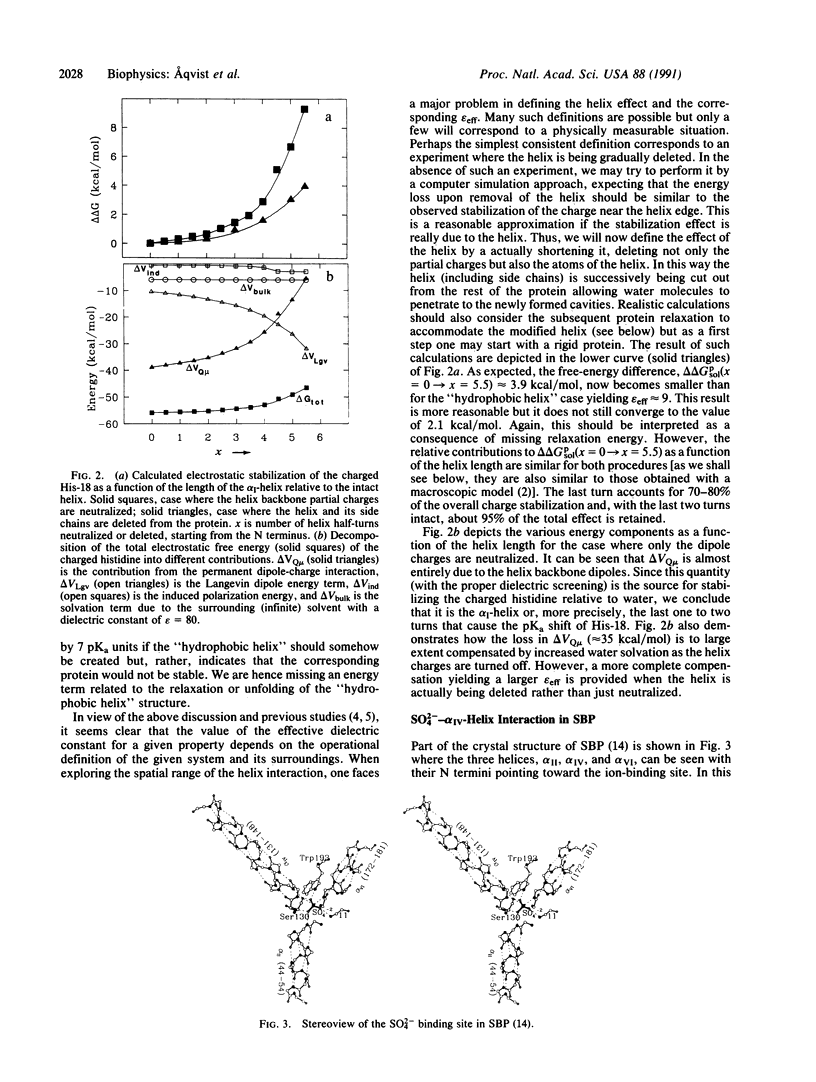
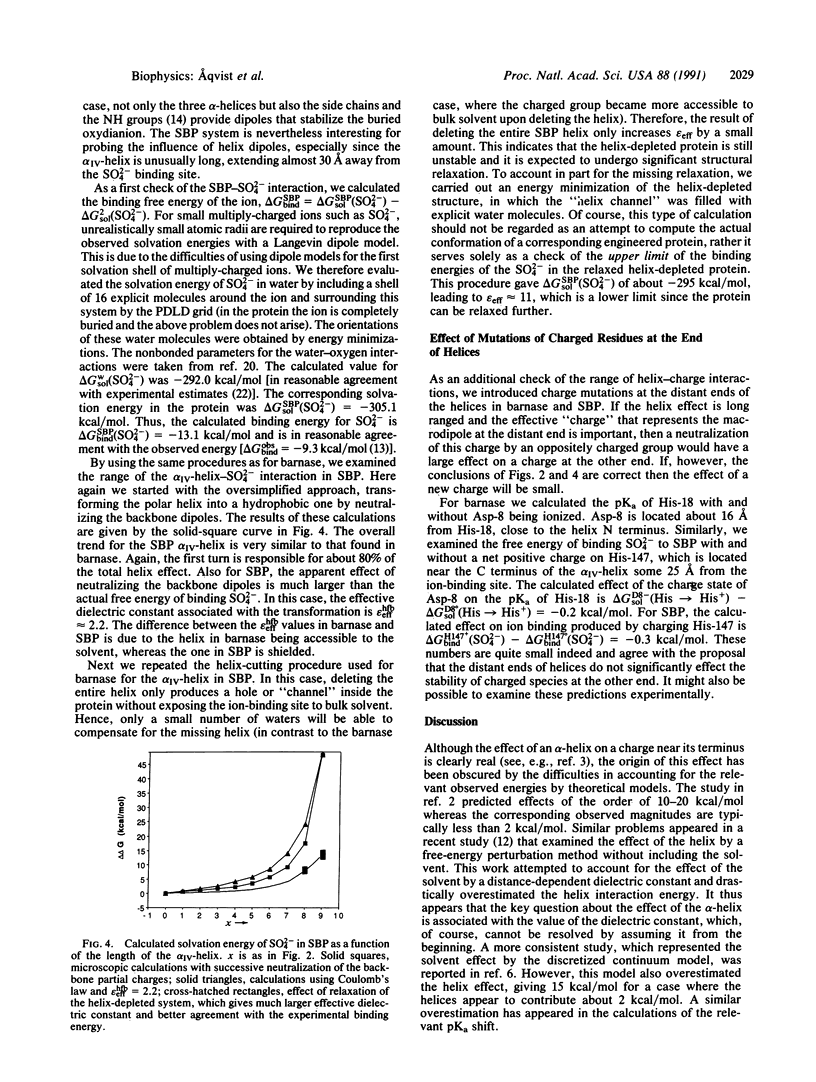
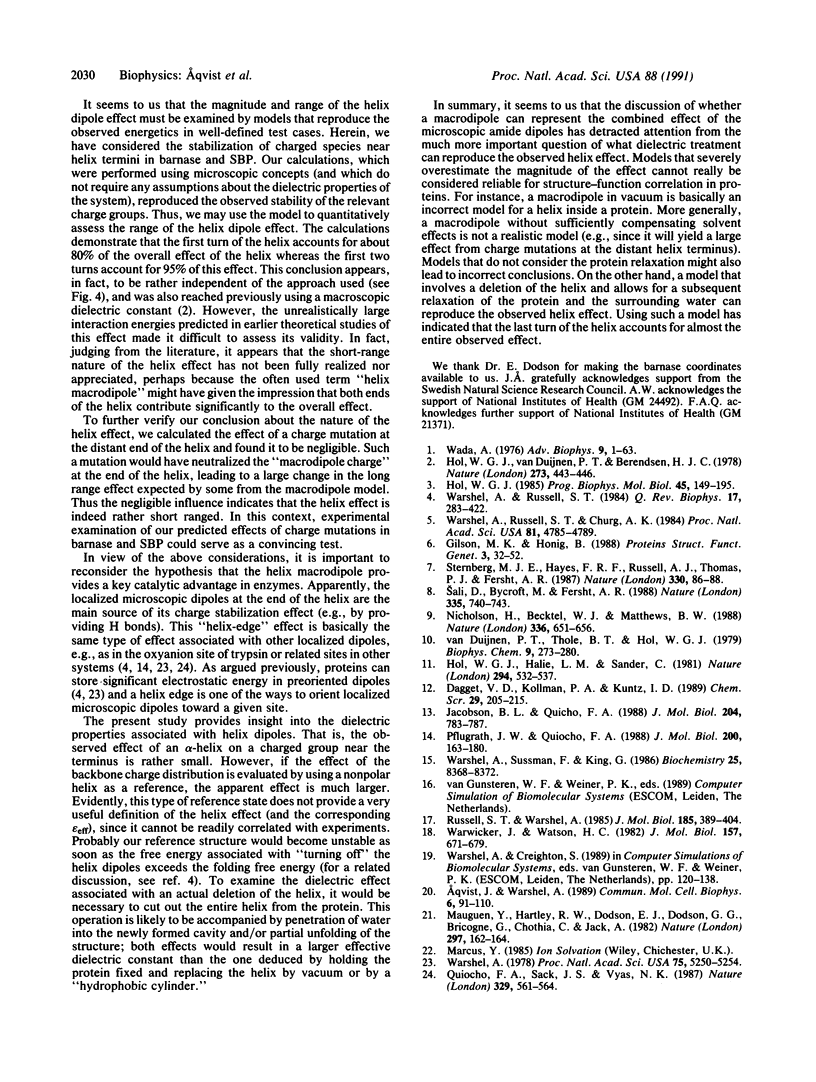
Selected References
These references are in PubMed. This may not be the complete list of references from this article.
- Gilson M. K., Honig B. H. Energetics of charge-charge interactions in proteins. Proteins. 1988;3(1):32–52. doi: 10.1002/prot.340030104. [DOI] [PubMed] [Google Scholar]
- Hol W. G., Halie L. M., Sander C. Dipoles of the alpha-helix and beta-sheet: their role in protein folding. Nature. 1981 Dec 10;294(5841):532–536. doi: 10.1038/294532a0. [DOI] [PubMed] [Google Scholar]
- Hol W. G. The role of the alpha-helix dipole in protein function and structure. Prog Biophys Mol Biol. 1985;45(3):149–195. doi: 10.1016/0079-6107(85)90001-x. [DOI] [PubMed] [Google Scholar]
- Hol W. G., van Duijnen P. T., Berendsen H. J. The alpha-helix dipole and the properties of proteins. Nature. 1978 Jun 8;273(5662):443–446. doi: 10.1038/273443a0. [DOI] [PubMed] [Google Scholar]
- Jacobson B. L., Quiocho F. A. Sulfate-binding protein dislikes protonated oxyacids. A molecular explanation. J Mol Biol. 1988 Dec 5;204(3):783–787. doi: 10.1016/0022-2836(88)90369-5. [DOI] [PubMed] [Google Scholar]
- Mauguen Y., Hartley R. W., Dodson E. J., Dodson G. G., Bricogne G., Chothia C., Jack A. Molecular structure of a new family of ribonucleases. Nature. 1982 May 13;297(5862):162–164. doi: 10.1038/297162a0. [DOI] [PubMed] [Google Scholar]
- Nicholson H., Becktel W. J., Matthews B. W. Enhanced protein thermostability from designed mutations that interact with alpha-helix dipoles. Nature. 1988 Dec 15;336(6200):651–656. doi: 10.1038/336651a0. [DOI] [PubMed] [Google Scholar]
- Pflugrath J. W., Quiocho F. A. The 2 A resolution structure of the sulfate-binding protein involved in active transport in Salmonella typhimurium. J Mol Biol. 1988 Mar 5;200(1):163–180. doi: 10.1016/0022-2836(88)90341-5. [DOI] [PubMed] [Google Scholar]
- Quiocho F. A., Sack J. S., Vyas N. K. Stabilization of charges on isolated ionic groups sequestered in proteins by polarized peptide units. Nature. 1987 Oct 8;329(6139):561–564. doi: 10.1038/329561a0. [DOI] [PubMed] [Google Scholar]
- Russell S. T., Warshel A. Calculations of electrostatic energies in proteins. The energetics of ionized groups in bovine pancreatic trypsin inhibitor. J Mol Biol. 1985 Sep 20;185(2):389–404. doi: 10.1016/0022-2836(85)90411-5. [DOI] [PubMed] [Google Scholar]
- Sali D., Bycroft M., Fersht A. R. Stabilization of protein structure by interaction of alpha-helix dipole with a charged side chain. Nature. 1988 Oct 20;335(6192):740–743. doi: 10.1038/335740a0. [DOI] [PubMed] [Google Scholar]
- Sternberg M. J., Hayes F. R., Russell A. J., Thomas P. G., Fersht A. R. Prediction of electrostatic effects of engineering of protein charges. Nature. 1987 Nov 5;330(6143):86–88. doi: 10.1038/330086a0. [DOI] [PubMed] [Google Scholar]
- Wada A. The alpha-helix as an electric macro-dipole. Adv Biophys. 1976:1–63. [PubMed] [Google Scholar]
- Warshel A. Energetics of enzyme catalysis. Proc Natl Acad Sci U S A. 1978 Nov;75(11):5250–5254. doi: 10.1073/pnas.75.11.5250. [DOI] [PMC free article] [PubMed] [Google Scholar]
- Warshel A., Russell S. T. Calculations of electrostatic interactions in biological systems and in solutions. Q Rev Biophys. 1984 Aug;17(3):283–422. doi: 10.1017/s0033583500005333. [DOI] [PubMed] [Google Scholar]
- Warshel A., Russell S. T., Churg A. K. Macroscopic models for studies of electrostatic interactions in proteins: limitations and applicability. Proc Natl Acad Sci U S A. 1984 Aug;81(15):4785–4789. doi: 10.1073/pnas.81.15.4785. [DOI] [PMC free article] [PubMed] [Google Scholar]
- Warshel A., Sussman F., King G. Free energy of charges in solvated proteins: microscopic calculations using a reversible charging process. Biochemistry. 1986 Dec 30;25(26):8368–8372. doi: 10.1021/bi00374a006. [DOI] [PubMed] [Google Scholar]
- Warwicker J., Watson H. C. Calculation of the electric potential in the active site cleft due to alpha-helix dipoles. J Mol Biol. 1982 Jun 5;157(4):671–679. doi: 10.1016/0022-2836(82)90505-8. [DOI] [PubMed] [Google Scholar]
- van Duijnen P. T., Thole B. T., Hol W. G. On the role of the active site helix in papain, an ab initio molecular orbital study. Biophys Chem. 1979 Mar;9(3):273–280. doi: 10.1016/0301-4622(79)85010-3. [DOI] [PubMed] [Google Scholar]