Abstract
The rapid synthesis and breakdown of mRNA in prokaryotes can impose a significant energy drain on these cells. Previous in vivo studies [Duffy, J. J., Chaney, S. G. & Boyer, P. D. (1972) J. Mol. Biol. 64, 565-579; Chaney, S. G. & Boyer, P. D. (1972) J. Mol. Biol. 64, 581-591] indicated that while RNA turnover in Escherichia coli was hydrolytic, it was nonhydrolytic in Bacillus subtilis. Here we provide an explanation for these observations based on enzymatic analysis of extracts of these two organisms. RNA degradation to the mononucleotide level in E. coli extracts is due solely to two active ribonucleases, RNase II and polynucleotide phosphorylase, which act hydrolytically and phosphorolytically, respectively. RNase II activity represents close to 90% of the total activity of the extract, as expected for predominantly hydrolytic degradation in this organism. In contrast, RNase II is absent from B. subtilis extracts, and the primary mode of RNA degradation is phosphorolytic, employing the Bacillus equivalent of polynucleotide phosphorylase and releases nucleoside diphosphates as products. A low level of a Mn2(+)-stimulated, hydrolytic ribonuclease is also detectable in B. subtilis extracts. Overall, E. coli and B. subtilis extracts differ by about 20- to 100-fold, depending on the substrate, in their relative use of hydrolytic and phosphorolytic routes of RNA degradation. The relation of the mode of mRNA degradation to the environment of the cell is discussed.
Full text
PDF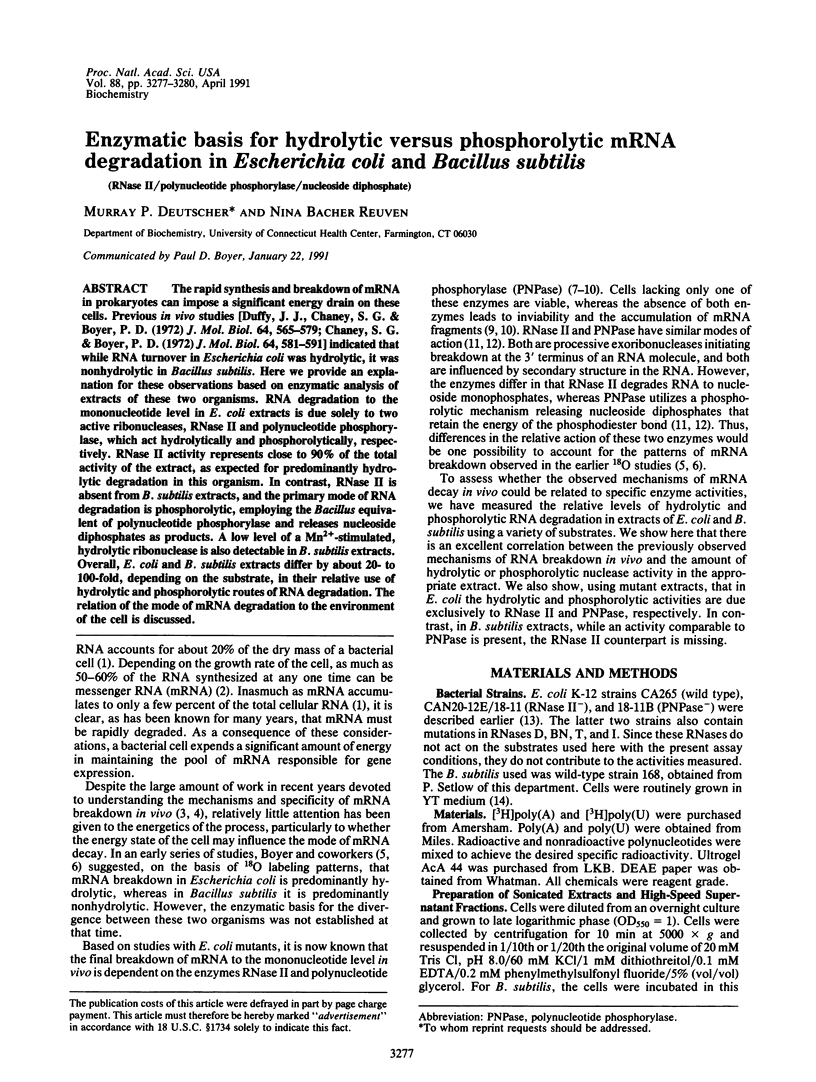
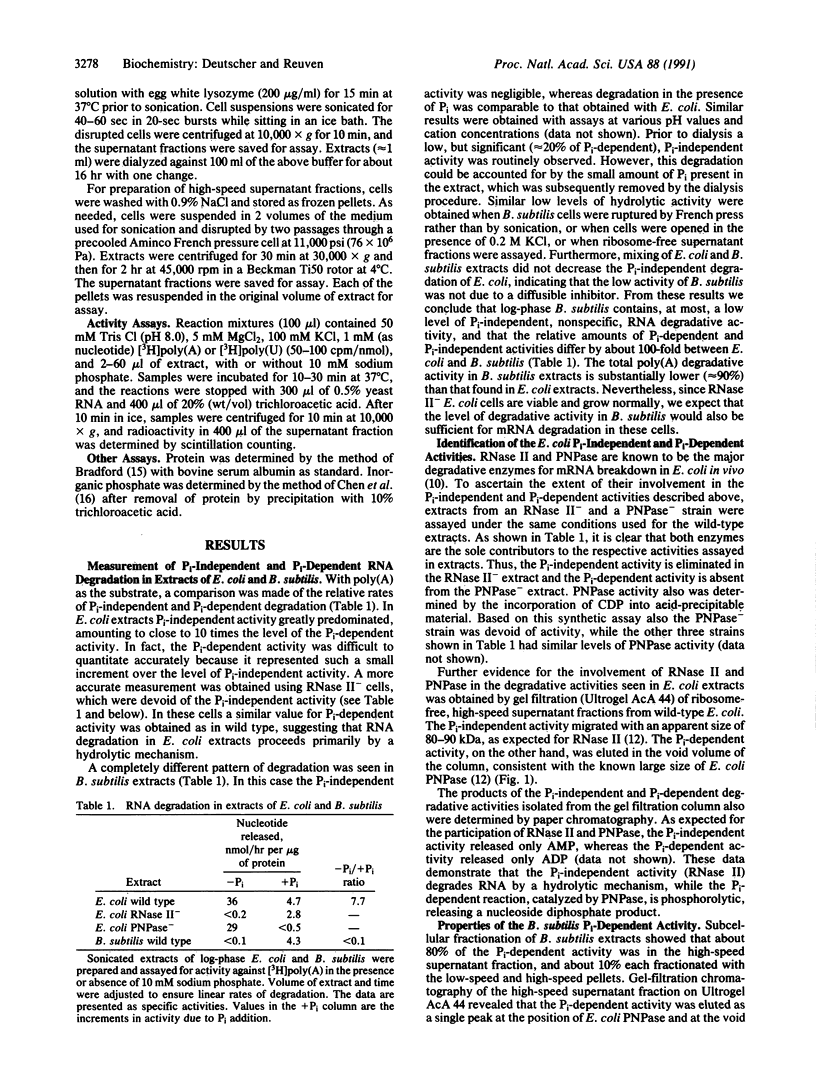
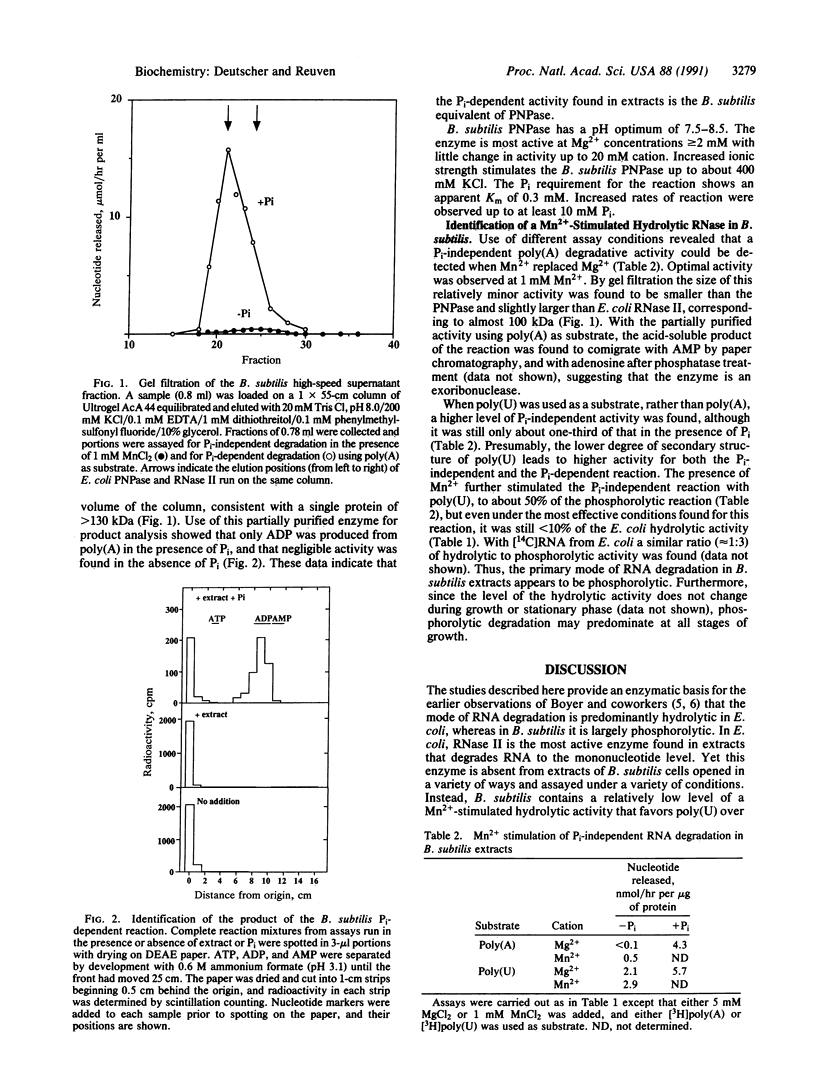
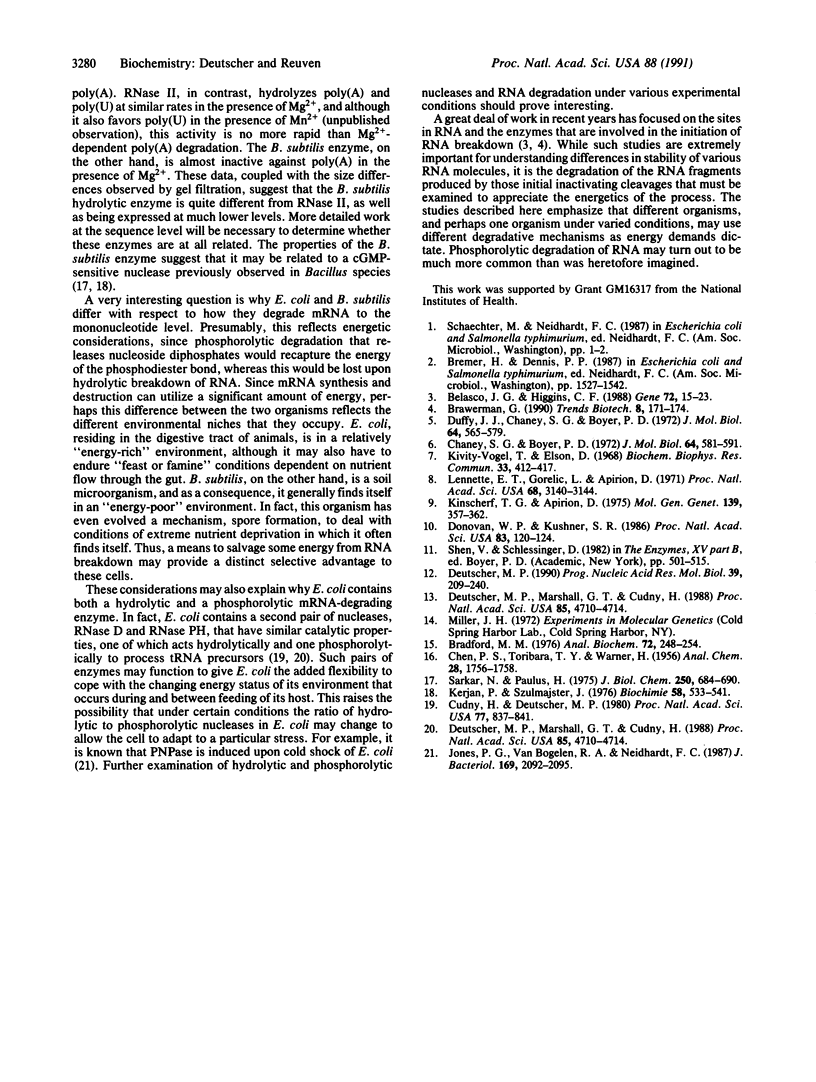
Selected References
These references are in PubMed. This may not be the complete list of references from this article.
- Belasco J. G., Higgins C. F. Mechanisms of mRNA decay in bacteria: a perspective. Gene. 1988 Dec 10;72(1-2):15–23. doi: 10.1016/0378-1119(88)90123-0. [DOI] [PubMed] [Google Scholar]
- Bradford M. M. A rapid and sensitive method for the quantitation of microgram quantities of protein utilizing the principle of protein-dye binding. Anal Biochem. 1976 May 7;72:248–254. doi: 10.1006/abio.1976.9999. [DOI] [PubMed] [Google Scholar]
- Brawerman G. Mechanisms of mRNA decay. Trends Biotechnol. 1990 Jul;8(7):171–174. doi: 10.1016/0167-7799(90)90167-v. [DOI] [PubMed] [Google Scholar]
- Chaney S. G., Boyer P. D. Incorporation of water oxygens into intracellular nucleotides and RNA. II. Predominantly hydrolytic RNA turnover in Escherichia coli. J Mol Biol. 1972 Mar 14;64(3):581–591. doi: 10.1016/0022-2836(72)90084-8. [DOI] [PubMed] [Google Scholar]
- Cudny H., Deutscher M. P. Apparent involvement of ribonuclease D in the 3' processing of tRNA precursors. Proc Natl Acad Sci U S A. 1980 Feb;77(2):837–841. doi: 10.1073/pnas.77.2.837. [DOI] [PMC free article] [PubMed] [Google Scholar]
- Deutscher M. P., Marshall G. T., Cudny H. RNase PH: an Escherichia coli phosphate-dependent nuclease distinct from polynucleotide phosphorylase. Proc Natl Acad Sci U S A. 1988 Jul;85(13):4710–4714. doi: 10.1073/pnas.85.13.4710. [DOI] [PMC free article] [PubMed] [Google Scholar]
- Deutscher M. P., Marshall G. T., Cudny H. RNase PH: an Escherichia coli phosphate-dependent nuclease distinct from polynucleotide phosphorylase. Proc Natl Acad Sci U S A. 1988 Jul;85(13):4710–4714. doi: 10.1073/pnas.85.13.4710. [DOI] [PMC free article] [PubMed] [Google Scholar]
- Deutscher M. P. Ribonucleases, tRNA nucleotidyltransferase, and the 3' processing of tRNA. Prog Nucleic Acid Res Mol Biol. 1990;39:209–240. doi: 10.1016/s0079-6603(08)60628-5. [DOI] [PubMed] [Google Scholar]
- Donovan W. P., Kushner S. R. Polynucleotide phosphorylase and ribonuclease II are required for cell viability and mRNA turnover in Escherichia coli K-12. Proc Natl Acad Sci U S A. 1986 Jan;83(1):120–124. doi: 10.1073/pnas.83.1.120. [DOI] [PMC free article] [PubMed] [Google Scholar]
- Duffy J. J., Chaney S. G., Boyer P. D. Incorporation of water oxygens into intracellular nucleotides and RNA. I. Predominantly non-hydrolytic RNA turnover in Bacillus subtilis. J Mol Biol. 1972 Mar 14;64(3):565–579. doi: 10.1016/0022-2836(72)90083-6. [DOI] [PubMed] [Google Scholar]
- Jones P. G., VanBogelen R. A., Neidhardt F. C. Induction of proteins in response to low temperature in Escherichia coli. J Bacteriol. 1987 May;169(5):2092–2095. doi: 10.1128/jb.169.5.2092-2095.1987. [DOI] [PMC free article] [PubMed] [Google Scholar]
- Kerjan P., Szulmajster J. Isolation and properties of a cyclic guanosine-monophosphate sensitive intracellular ribonuclease from Bacillus subtilis. Biochimie. 1976;58(5):533–541. doi: 10.1016/s0300-9084(76)80223-4. [DOI] [PubMed] [Google Scholar]
- Kinscherf T. G., Apirion D. Polynucleotide phosphorylase can participate in decay of mRNA in Escherichia coli in the absence of ribonuclease II. Mol Gen Genet. 1975 Sep 8;139(4):357–362. doi: 10.1007/BF00267975. [DOI] [PubMed] [Google Scholar]
- Kivity-Vogel T., Elson D. A correlation between ribonuclease II and the in vivo inactivation of messenger RNA in E. coli. Biochem Biophys Res Commun. 1968 Nov 8;33(3):412–417. doi: 10.1016/0006-291x(68)90587-1. [DOI] [PubMed] [Google Scholar]
- Lennette E. T., Gorelic L., Apirion D. An Escherichia coli mutant with increased messenger ribonuclease activity. Proc Natl Acad Sci U S A. 1971 Dec;68(12):3140–3144. doi: 10.1073/pnas.68.12.3140. [DOI] [PMC free article] [PubMed] [Google Scholar]
- Sarkar N., Paulus H. A guanosine 3':5'-monophosphate-sensitive nuclease from Bacillus brevis. J Biol Chem. 1975 Jan 25;250(2):684–690. [PubMed] [Google Scholar]