Abstract
The standard enthalpy or entropy change upon transfer of a small nonpolar molecule from a nonaqueous phase into water at a given temperature is generally different for different solute species. However, if the heat capacity change is independent of temperature, there exists a temperature at which the enthalpy or the entropy change becomes the same for all solute species within a given class. Similarly, the enthalpy or the entropy change of protein denaturation, when extrapolated to high temperature assuming a temperature-independent heat capacity change, shows a temperature at which its value becomes the same for many different globular proteins on a per weight basis. It is shown that the existence of these temperatures can be explained from a common formalism based on a linear relationship between the thermodynamic quantity and a temperature-independent molecular property that characterizes the solute or the protein. For the small nonpolar molecule transfer processes, this property is the surface area or the number of groups that are brought in contact with water. For protein denaturation, it is suggested that this property measures the polar/nonpolar mix of the internal interaction within the protein interior. Under a certain set of assumptions, this model leads to the conclusion that the nonpolar and the polar groups of the protein contribute roughly equally to the stability of the folded state of the molecule and that the solvent-accessible surface area of the denatured form of a protein is no more than about two-thirds that of the fully extended form.
Full text
PDF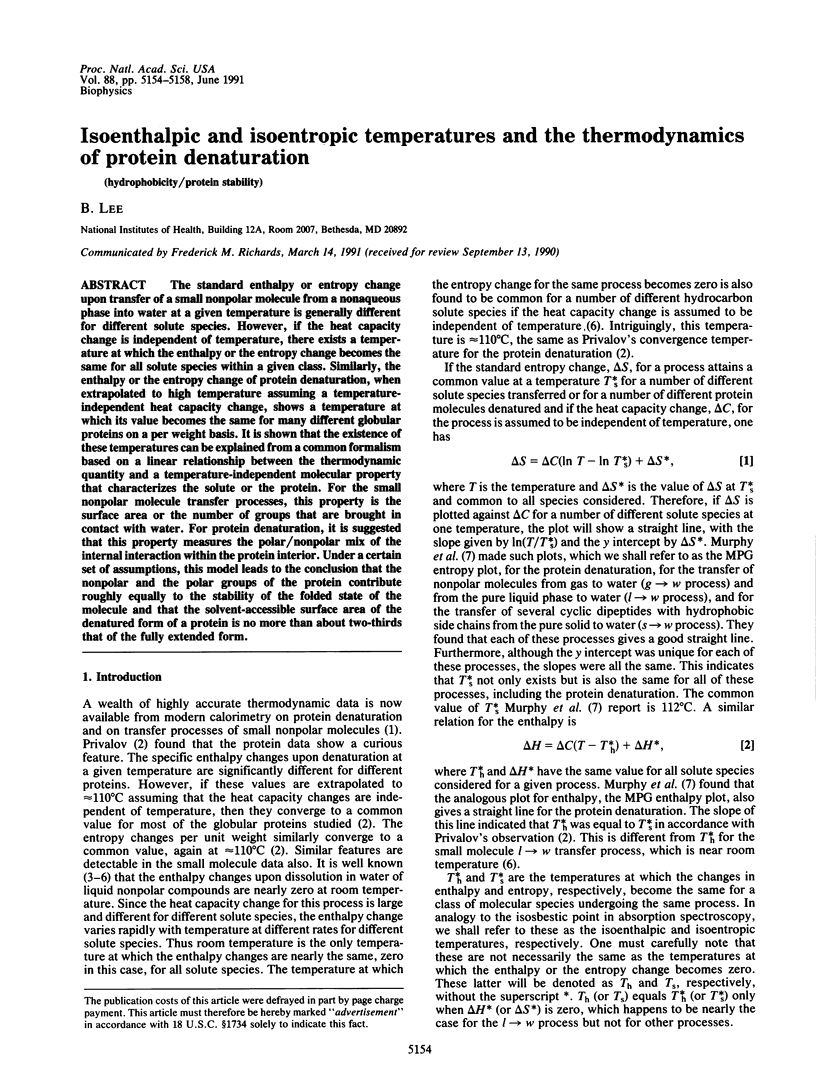
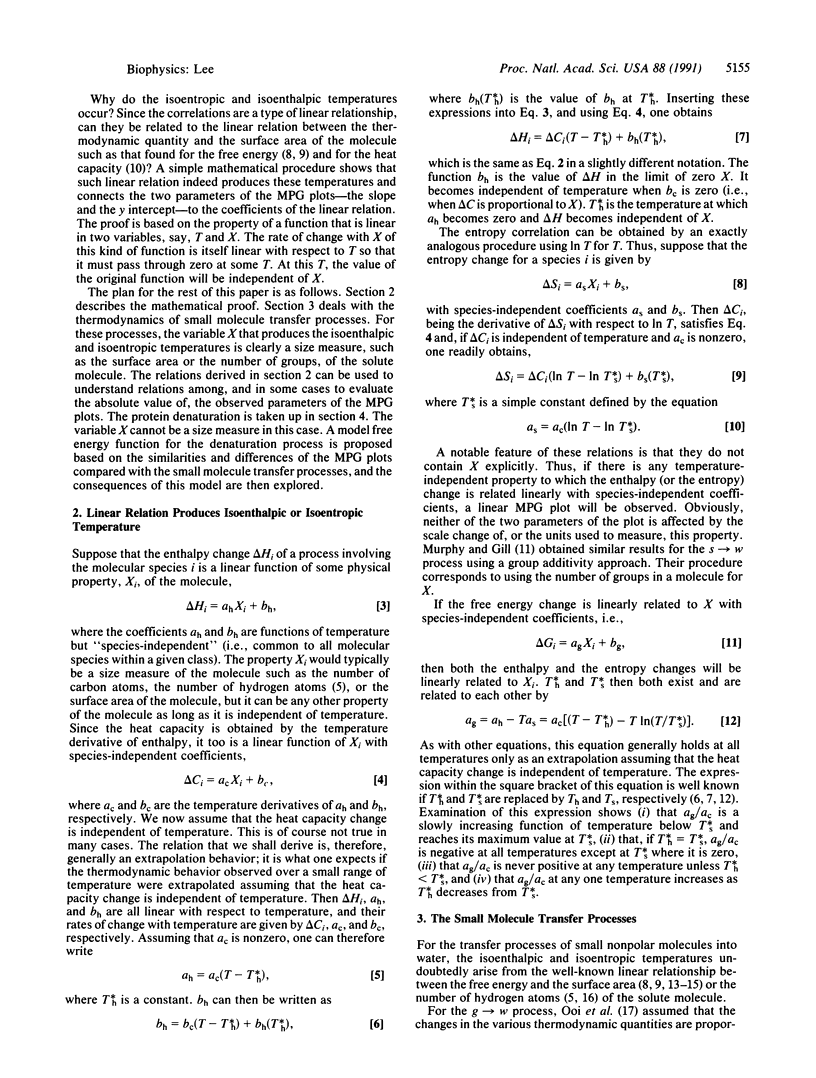
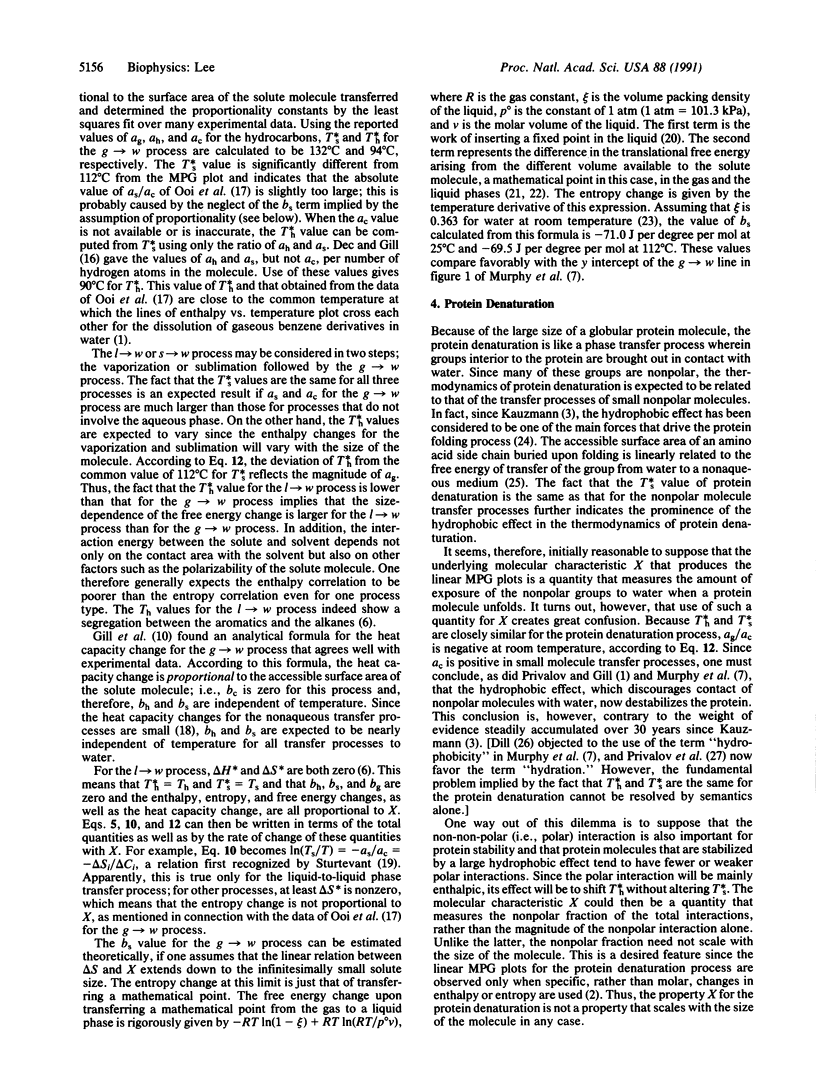
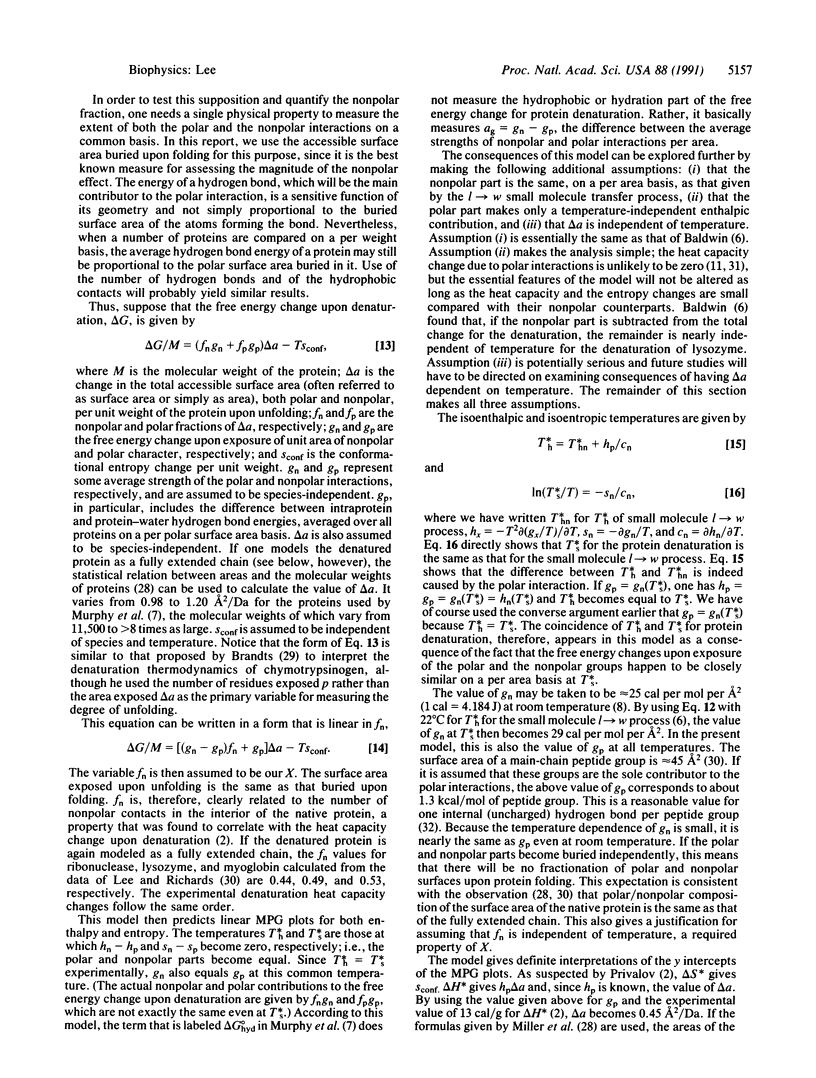
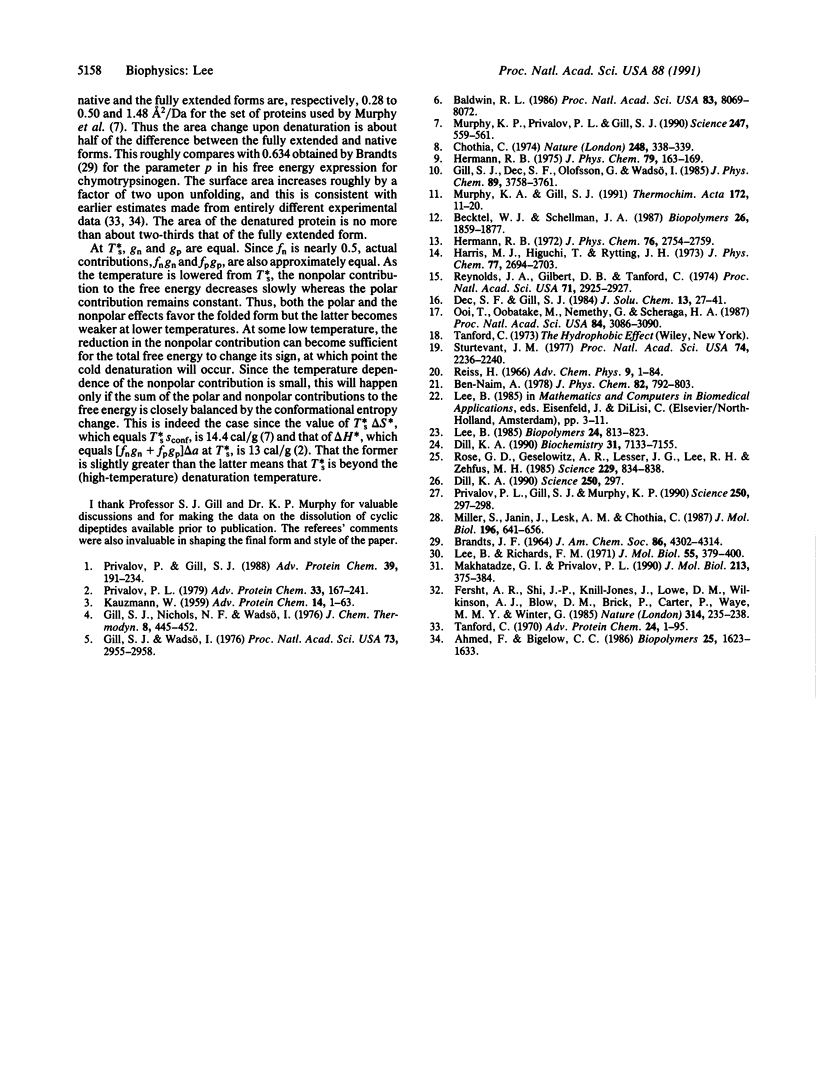
Selected References
These references are in PubMed. This may not be the complete list of references from this article.
- Baldwin R. L. Temperature dependence of the hydrophobic interaction in protein folding. Proc Natl Acad Sci U S A. 1986 Nov;83(21):8069–8072. doi: 10.1073/pnas.83.21.8069. [DOI] [PMC free article] [PubMed] [Google Scholar]
- Becktel W. J., Schellman J. A. Protein stability curves. Biopolymers. 1987 Nov;26(11):1859–1877. doi: 10.1002/bip.360261104. [DOI] [PubMed] [Google Scholar]
- Chothia C. Hydrophobic bonding and accessible surface area in proteins. Nature. 1974 Mar 22;248(446):338–339. doi: 10.1038/248338a0. [DOI] [PubMed] [Google Scholar]
- Dill K. A. Dominant forces in protein folding. Biochemistry. 1990 Aug 7;29(31):7133–7155. doi: 10.1021/bi00483a001. [DOI] [PubMed] [Google Scholar]
- Dill K. A. The meaning of hydrophobicity. Science. 1990 Oct 12;250(4978):297–298. doi: 10.1126/science.2218535. [DOI] [PubMed] [Google Scholar]
- Dill K. A. The meaning of hydrophobicity. Science. 1990 Oct 12;250(4978):297–298. doi: 10.1126/science.2218535. [DOI] [PubMed] [Google Scholar]
- Fersht A. R., Shi J. P., Knill-Jones J., Lowe D. M., Wilkinson A. J., Blow D. M., Brick P., Carter P., Waye M. M., Winter G. Hydrogen bonding and biological specificity analysed by protein engineering. Nature. 1985 Mar 21;314(6008):235–238. doi: 10.1038/314235a0. [DOI] [PubMed] [Google Scholar]
- Gill S. J., Wadsö I. An equation of state describing hydrophobic interactions. Proc Natl Acad Sci U S A. 1976 Sep;73(9):2955–2958. doi: 10.1073/pnas.73.9.2955. [DOI] [PMC free article] [PubMed] [Google Scholar]
- Hermann R. E. Selective, conservative operations for cancer of the breast. Cleve Clin Q. 1975 Summer;42(2):163–169. doi: 10.3949/ccjm.42.2.163. [DOI] [PubMed] [Google Scholar]
- KAUZMANN W. Some factors in the interpretation of protein denaturation. Adv Protein Chem. 1959;14:1–63. doi: 10.1016/s0065-3233(08)60608-7. [DOI] [PubMed] [Google Scholar]
- Lee B., Richards F. M. The interpretation of protein structures: estimation of static accessibility. J Mol Biol. 1971 Feb 14;55(3):379–400. doi: 10.1016/0022-2836(71)90324-x. [DOI] [PubMed] [Google Scholar]
- Lee B. The physical origin of the low solubility of nonpolar solutes in water. Biopolymers. 1985 May;24(5):813–823. doi: 10.1002/bip.360240507. [DOI] [PubMed] [Google Scholar]
- Makhatadze G. I., Privalov P. L. Heat capacity of proteins. I. Partial molar heat capacity of individual amino acid residues in aqueous solution: hydration effect. J Mol Biol. 1990 May 20;213(2):375–384. doi: 10.1016/S0022-2836(05)80197-4. [DOI] [PubMed] [Google Scholar]
- Miller S., Janin J., Lesk A. M., Chothia C. Interior and surface of monomeric proteins. J Mol Biol. 1987 Aug 5;196(3):641–656. doi: 10.1016/0022-2836(87)90038-6. [DOI] [PubMed] [Google Scholar]
- Murphy K. P., Privalov P. L., Gill S. J. Common features of protein unfolding and dissolution of hydrophobic compounds. Science. 1990 Feb 2;247(4942):559–561. doi: 10.1126/science.2300815. [DOI] [PubMed] [Google Scholar]
- Ooi T., Oobatake M., Némethy G., Scheraga H. A. Accessible surface areas as a measure of the thermodynamic parameters of hydration of peptides. Proc Natl Acad Sci U S A. 1987 May;84(10):3086–3090. doi: 10.1073/pnas.84.10.3086. [DOI] [PMC free article] [PubMed] [Google Scholar]
- Privalov P. L., Gill S. J. Stability of protein structure and hydrophobic interaction. Adv Protein Chem. 1988;39:191–234. doi: 10.1016/s0065-3233(08)60377-0. [DOI] [PubMed] [Google Scholar]
- Privalov P. L. Stability of proteins: small globular proteins. Adv Protein Chem. 1979;33:167–241. doi: 10.1016/s0065-3233(08)60460-x. [DOI] [PubMed] [Google Scholar]
- Reynolds J. A., Gilbert D. B., Tanford C. Empirical correlation between hydrophobic free energy and aqueous cavity surface area. Proc Natl Acad Sci U S A. 1974 Aug;71(8):2925–2927. doi: 10.1073/pnas.71.8.2925. [DOI] [PMC free article] [PubMed] [Google Scholar]
- Rose G. D., Geselowitz A. R., Lesser G. J., Lee R. H., Zehfus M. H. Hydrophobicity of amino acid residues in globular proteins. Science. 1985 Aug 30;229(4716):834–838. doi: 10.1126/science.4023714. [DOI] [PubMed] [Google Scholar]
- Sturtevant J. M. Heat capacity and entropy changes in processes involving proteins. Proc Natl Acad Sci U S A. 1977 Jun;74(6):2236–2240. doi: 10.1073/pnas.74.6.2236. [DOI] [PMC free article] [PubMed] [Google Scholar]
- Tanford C. Protein denaturation. C. Theoretical models for the mechanism of denaturation. Adv Protein Chem. 1970;24:1–95. [PubMed] [Google Scholar]