Abstract
Searches of ribosomal RNA sequences for compensatory base changes preserving Watson-Crick base pairing have led to detailed models of the conserved secondary structures of these RNAs. In principle, tertiary interactions can also be detected by searches for phylogenetically covariant bases. Within a highly conserved region of the large subunit ribosomal RNA termed the "GTPase center," the bases G-1056-U-1082.A-1086 are found in all eubacteria (Escherichia coli numbering), while A-1056.C-1082.G-1086 are found at the homologous positions in eukaryotes; archaebacteria fall into either category with some exceptions. Either sequence can potentially form a similar set of hydrogen bonds connecting the 3 bases. To determine the contribution of these 3 bases to RNA tertiary structure, sequence variants were made in RNA fragments covering the GTPase center. Correct folding of the RNA fragments was assayed by measuring the binding affinities of two different ligands that recognize the RNA tertiary structure: the highly conserved ribosomal protein L11, which is normally associated with the GTPase center RNA, and the peptide antibiotic thiostrepton, which inhibits the GTPase activity of eubacterial and some archaebacterial ribosomes. The results strongly support the existence of a base pair between positions 1082 and 1086: single mutations at either position weaken both L11 and thiostrepton binding by approximately 10-fold or more, while compensatory double mutations bind the ligands nearly as well as the wild-type E. coli sequence. Variants at position 1056 have little effect on either L11 or thiostrepton binding; a 3-base interaction is therefore not supported by these experiments. A base pair between positions 1082 and 1086 strongly constrains the geometry with which three helical segments join in the middle of the GTPase center.
Full text
PDF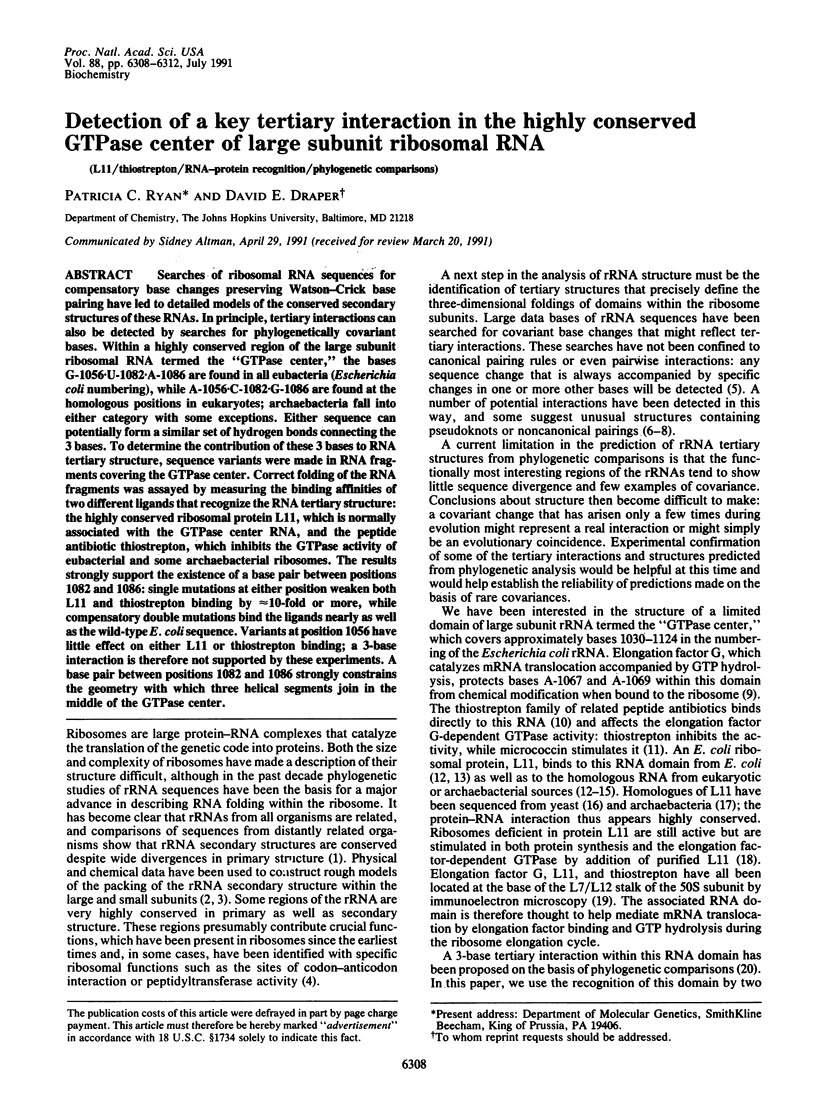
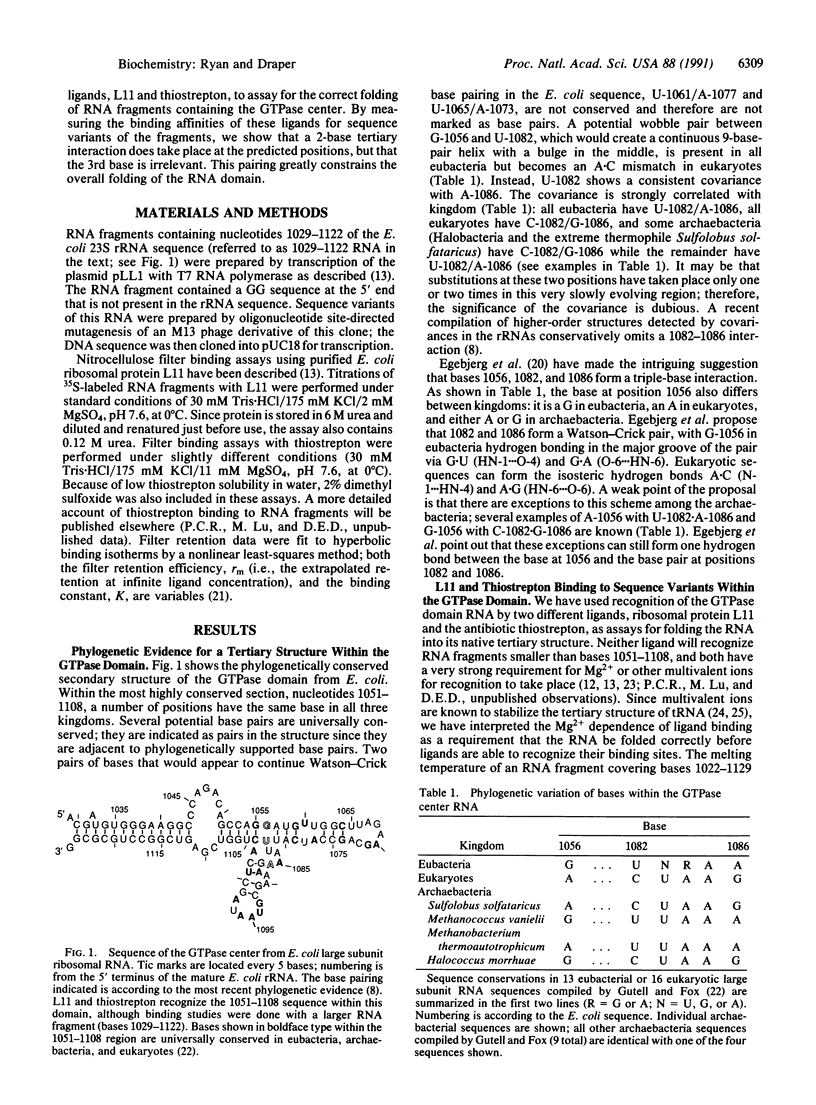
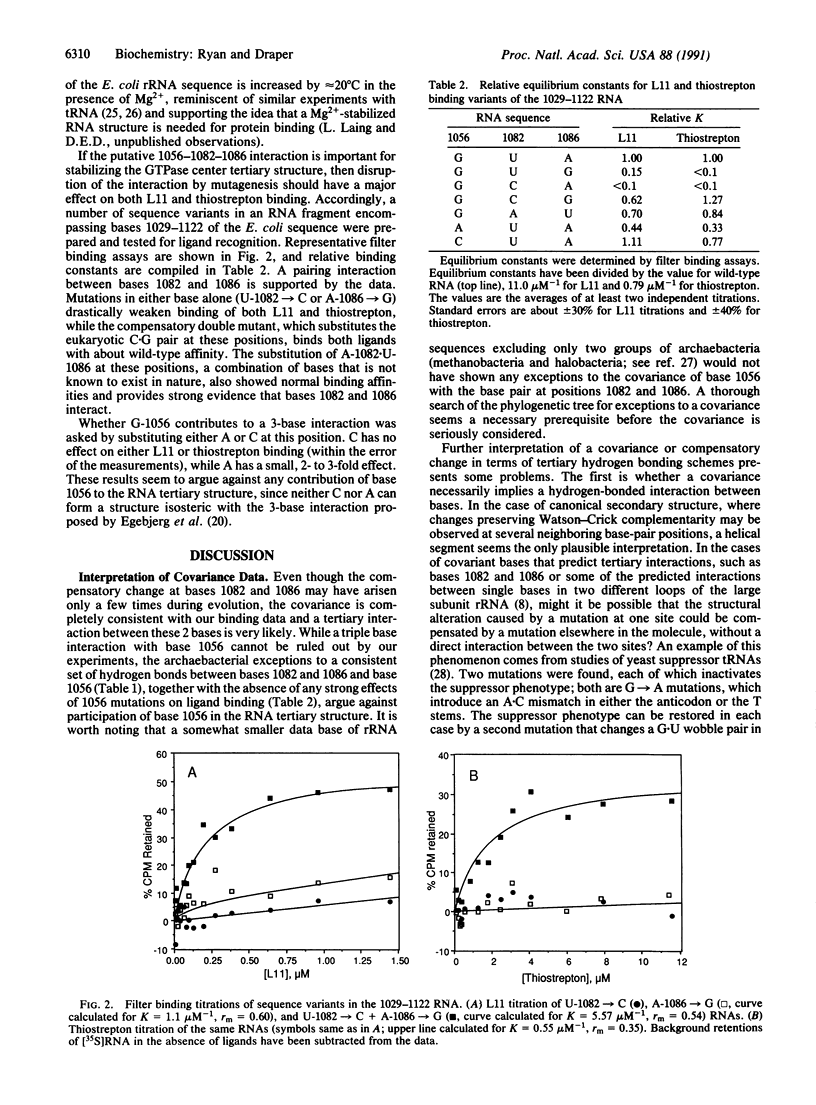
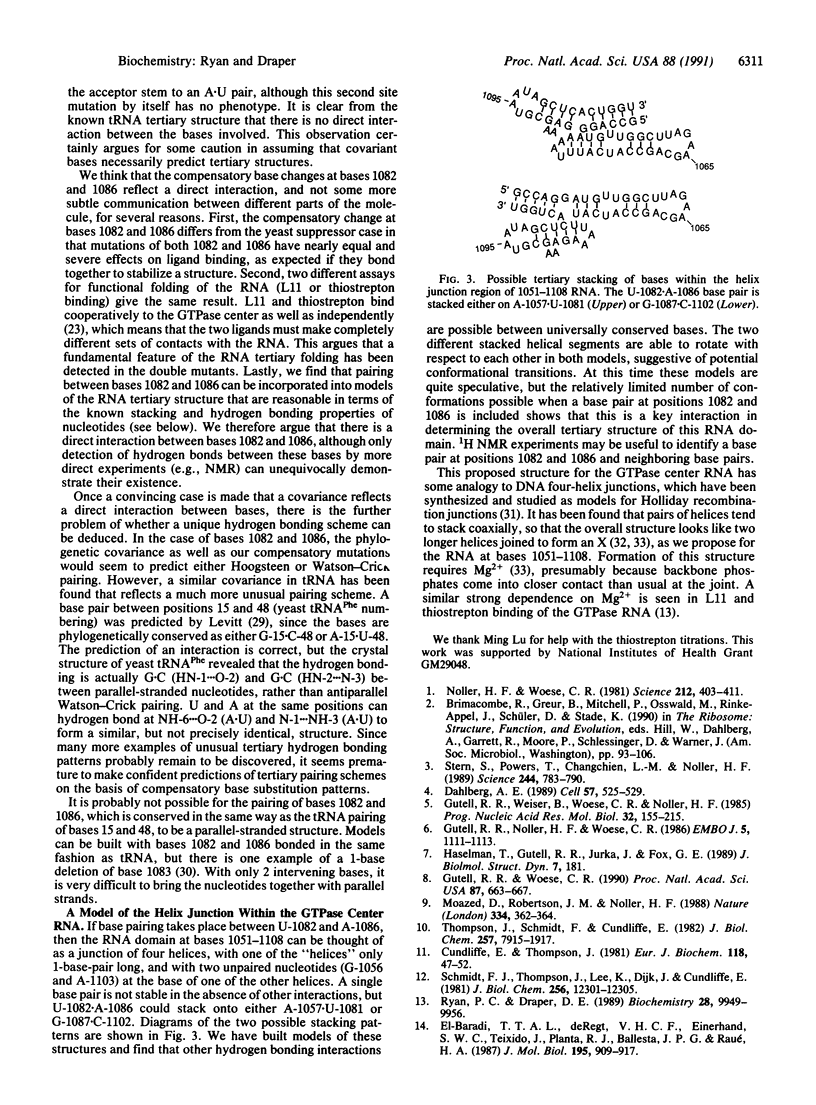
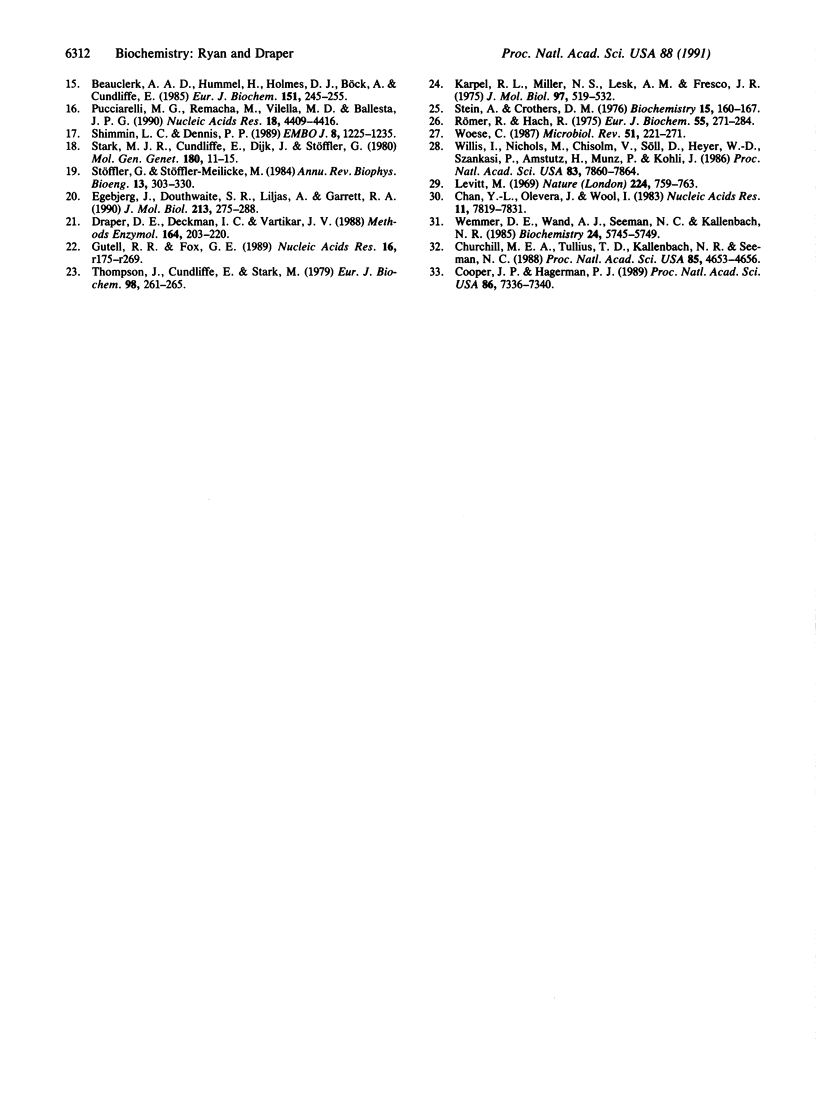
Selected References
These references are in PubMed. This may not be the complete list of references from this article.
- Beauclerk A. A., Hummel H., Holmes D. J., Böck A., Cundliffe E. Studies of the GTPase domain of archaebacterial ribosomes. Eur J Biochem. 1985 Sep 2;151(2):245–255. doi: 10.1111/j.1432-1033.1985.tb09095.x. [DOI] [PubMed] [Google Scholar]
- Chan Y. L., Olvera J., Wool I. G. The structure of rat 28S ribosomal ribonucleic acid inferred from the sequence of nucleotides in a gene. Nucleic Acids Res. 1983 Nov 25;11(22):7819–7831. doi: 10.1093/nar/11.22.7819. [DOI] [PMC free article] [PubMed] [Google Scholar]
- Churchill M. E., Tullius T. D., Kallenbach N. R., Seeman N. C. A Holliday recombination intermediate is twofold symmetric. Proc Natl Acad Sci U S A. 1988 Jul;85(13):4653–4656. doi: 10.1073/pnas.85.13.4653. [DOI] [PMC free article] [PubMed] [Google Scholar]
- Cooper J. P., Hagerman P. J. Geometry of a branched DNA structure in solution. Proc Natl Acad Sci U S A. 1989 Oct;86(19):7336–7340. doi: 10.1073/pnas.86.19.7336. [DOI] [PMC free article] [PubMed] [Google Scholar]
- Cundliffe E., Thompson J. Concerning the mode of action of micrococcin upon bacterial protein synthesis. Eur J Biochem. 1981 Aug;118(1):47–52. doi: 10.1111/j.1432-1033.1981.tb05484.x. [DOI] [PubMed] [Google Scholar]
- Dahlberg A. E. The functional role of ribosomal RNA in protein synthesis. Cell. 1989 May 19;57(4):525–529. doi: 10.1016/0092-8674(89)90122-0. [DOI] [PubMed] [Google Scholar]
- Draper D. E., Deckman I. C., Vartikar J. V. Physical studies of ribosomal protein-RNA interactions. Methods Enzymol. 1988;164:203–220. doi: 10.1016/s0076-6879(88)64044-4. [DOI] [PubMed] [Google Scholar]
- Egebjerg J., Douthwaite S. R., Liljas A., Garrett R. A. Characterization of the binding sites of protein L11 and the L10.(L12)4 pentameric complex in the GTPase domain of 23 S ribosomal RNA from Escherichia coli. J Mol Biol. 1990 May 20;213(2):275–288. doi: 10.1016/S0022-2836(05)80190-1. [DOI] [PubMed] [Google Scholar]
- Gutell R. R., Fox G. E. A compilation of large subunit RNA sequences presented in a structural format. Nucleic Acids Res. 1988;16 (Suppl):r175–r269. doi: 10.1093/nar/16.suppl.r175. [DOI] [PMC free article] [PubMed] [Google Scholar]
- Gutell R. R., Noller H. F., Woese C. R. Higher order structure in ribosomal RNA. EMBO J. 1986 May;5(5):1111–1113. doi: 10.1002/j.1460-2075.1986.tb04330.x. [DOI] [PMC free article] [PubMed] [Google Scholar]
- Gutell R. R., Weiser B., Woese C. R., Noller H. F. Comparative anatomy of 16-S-like ribosomal RNA. Prog Nucleic Acid Res Mol Biol. 1985;32:155–216. doi: 10.1016/s0079-6603(08)60348-7. [DOI] [PubMed] [Google Scholar]
- Gutell R. R., Woese C. R. Higher order structural elements in ribosomal RNAs: pseudo-knots and the use of noncanonical pairs. Proc Natl Acad Sci U S A. 1990 Jan;87(2):663–667. doi: 10.1073/pnas.87.2.663. [DOI] [PMC free article] [PubMed] [Google Scholar]
- Haselman T., Gutell R. R., Jurka J., Fox G. E. Additional Watson-Crick interactions suggest a structural core in large subunit ribosomal RNA. J Biomol Struct Dyn. 1989 Aug;7(1):181–186. doi: 10.1080/07391102.1989.10507759. [DOI] [PubMed] [Google Scholar]
- Karpel R. L., Miller N. S., Lesk A. M., Fresco J. R. Stabilization of the native tertiary stucture of yeast tRNALeu3 by cationic metal complexes. J Mol Biol. 1975 Oct 5;97(4):519–532. doi: 10.1016/s0022-2836(75)80056-8. [DOI] [PubMed] [Google Scholar]
- Levitt M. Detailed molecular model for transfer ribonucleic acid. Nature. 1969 Nov 22;224(5221):759–763. doi: 10.1038/224759a0. [DOI] [PubMed] [Google Scholar]
- Moazed D., Robertson J. M., Noller H. F. Interaction of elongation factors EF-G and EF-Tu with a conserved loop in 23S RNA. Nature. 1988 Jul 28;334(6180):362–364. doi: 10.1038/334362a0. [DOI] [PubMed] [Google Scholar]
- Noller H. F., Woese C. R. Secondary structure of 16S ribosomal RNA. Science. 1981 Apr 24;212(4493):403–411. doi: 10.1126/science.6163215. [DOI] [PubMed] [Google Scholar]
- Pucciarelli M. G., Remacha M., Vilella M. D., Ballesta J. P. The 26S rRNA binding ribosomal protein equivalent to bacterial protein L11 is encoded by unspliced duplicated genes in Saccharomyces cerevisiae. Nucleic Acids Res. 1990 Aug 11;18(15):4409–4416. doi: 10.1093/nar/18.15.4409. [DOI] [PMC free article] [PubMed] [Google Scholar]
- Ryan P. C., Draper D. E. Thermodynamics of protein-RNA recognition in a highly conserved region of the large-subunit ribosomal RNA. Biochemistry. 1989 Dec 26;28(26):9949–9956. doi: 10.1021/bi00452a012. [DOI] [PubMed] [Google Scholar]
- Römer R., Hach R. tRNA conformation and magnesium binding. A study of a yeast phenylalanine-specific tRNA by a fluorescent indicator and differential melting curves. Eur J Biochem. 1975 Jun 16;55(1):271–284. doi: 10.1111/j.1432-1033.1975.tb02160.x. [DOI] [PubMed] [Google Scholar]
- Schmidt F. J., Thompson J., Lee K., Dijk J., Cundliffe E. The binding site for ribosomal protein L11 within 23 S ribosomal RNA of Escherichia coli. J Biol Chem. 1981 Dec 10;256(23):12301–12305. [PubMed] [Google Scholar]
- Shimmin L. C., Dennis P. P. Characterization of the L11, L1, L10 and L12 equivalent ribosomal protein gene cluster of the halophilic archaebacterium Halobacterium cutirubrum. EMBO J. 1989 Apr;8(4):1225–1235. doi: 10.1002/j.1460-2075.1989.tb03496.x. [DOI] [PMC free article] [PubMed] [Google Scholar]
- Stark M. J., Cundliffe E., Dijk J., Stöffler G. Functional homology between E. coli ribosomal protein L11 and B. megaterium protein BM-L11. Mol Gen Genet. 1980;180(1):11–15. doi: 10.1007/BF00267346. [DOI] [PubMed] [Google Scholar]
- Stein A., Crothers D. M. Conformational changes of transfer RNA. The role of magnesium(II). Biochemistry. 1976 Jan 13;15(1):160–168. doi: 10.1021/bi00646a025. [DOI] [PubMed] [Google Scholar]
- Stern S., Powers T., Changchien L. M., Noller H. F. RNA-protein interactions in 30S ribosomal subunits: folding and function of 16S rRNA. Science. 1989 May 19;244(4906):783–790. doi: 10.1126/science.2658053. [DOI] [PubMed] [Google Scholar]
- Stöffler G., Stöffler-Meilicke M. Immunoelectron microscopy of ribosomes. Annu Rev Biophys Bioeng. 1984;13:303–330. doi: 10.1146/annurev.bb.13.060184.001511. [DOI] [PubMed] [Google Scholar]
- Thompson J., Cundliffe E., Stark M. Binding of thiostrepton to a complex of 23-S rRNA with ribosomal protein L11. Eur J Biochem. 1979 Jul;98(1):261–265. doi: 10.1111/j.1432-1033.1979.tb13184.x. [DOI] [PubMed] [Google Scholar]
- Thompson J., Schmidt F., Cundliffe E. Site of action of a ribosomal RNA methylase conferring resistance to thiostrepton. J Biol Chem. 1982 Jul 25;257(14):7915–7917. [PubMed] [Google Scholar]
- Wemmer D. E., Wand A. J., Seeman N. C., Kallenbach N. R. NMR analysis of DNA junctions: imino proton NMR studies of individual arms and intact junction. Biochemistry. 1985 Oct 8;24(21):5745–5749. doi: 10.1021/bi00342a009. [DOI] [PubMed] [Google Scholar]
- Willis I., Nichols M., Chisholm V., Söll D., Heyer W. D., Szankasi P., Amstutz H., Munz P., Kohli J. Functional complementation between mutations in a yeast suppressor tRNA gene reveals potential for evolution of tRNA sequences. Proc Natl Acad Sci U S A. 1986 Oct;83(20):7860–7864. doi: 10.1073/pnas.83.20.7860. [DOI] [PMC free article] [PubMed] [Google Scholar]
- Woese C. R. Bacterial evolution. Microbiol Rev. 1987 Jun;51(2):221–271. doi: 10.1128/mr.51.2.221-271.1987. [DOI] [PMC free article] [PubMed] [Google Scholar]
- el-Baradi T. T., de Regt V. C., Einerhand S. W., Teixido J., Planta R. J., Ballesta J. P., Raué H. A. Ribosomal proteins EL11 from Escherichia coli and L15 from Saccharomyces cerevisiae bind to the same site in both yeast 26 S and mouse 28 S rRNA. J Mol Biol. 1987 Jun 20;195(4):909–917. doi: 10.1016/0022-2836(87)90494-3. [DOI] [PubMed] [Google Scholar]