Abstract
Although GPC has long been recognized as a degradation product of phosphatidylcholine, only recently is there wide appreciation of its role as a compatible and counteracting osmolyte that protects cells from osmotic stress. GPC is osmotically regulated in renal cells. Its level varies directly with extracellular osmolality. Cells in the kidney medulla in vivo and in renal epithelial cell cultures (MDCK) accumulate large amounts of GPC when exposed to high concentrations of NaCl and urea. Osmotic regulation of GPC requires choline in the medium, presumably as a precursor for synthesis of GPC. Choline transport into the cells, however, is not osmoregulated. The purpose of the present studies was to use MDCK cell cultures as a defined model to distinguish whether osmotically induced accumulation of GPC results from increased GPC synthesis or decreased GPC disappearance. The rate of incorporation of 14C from [14C]choline into GPC, the steady-state GPC synthesis rate, and the activity of phospholipase A2 (which can catalyze a step in the synthesis of GPC from phosphatidylcholine) are not increased by high NaCl and urea. In fact all are decreased by approximately one-third. Therefore, we find no evidence that high NaCl and urea increases the GPC synthesis rate. On the other hand, the rate coefficient for cellular GPC disappearance and the activity of GPC:choline phosphodiesterase (EC 3.1.4.2), which catalyzes degradation of GPC, are decreased by approximately two-thirds by high NaCl and urea. We conclude that high NaCl and urea increase the level of GPC by inhibiting its enzymatic degradation.
Full text
PDF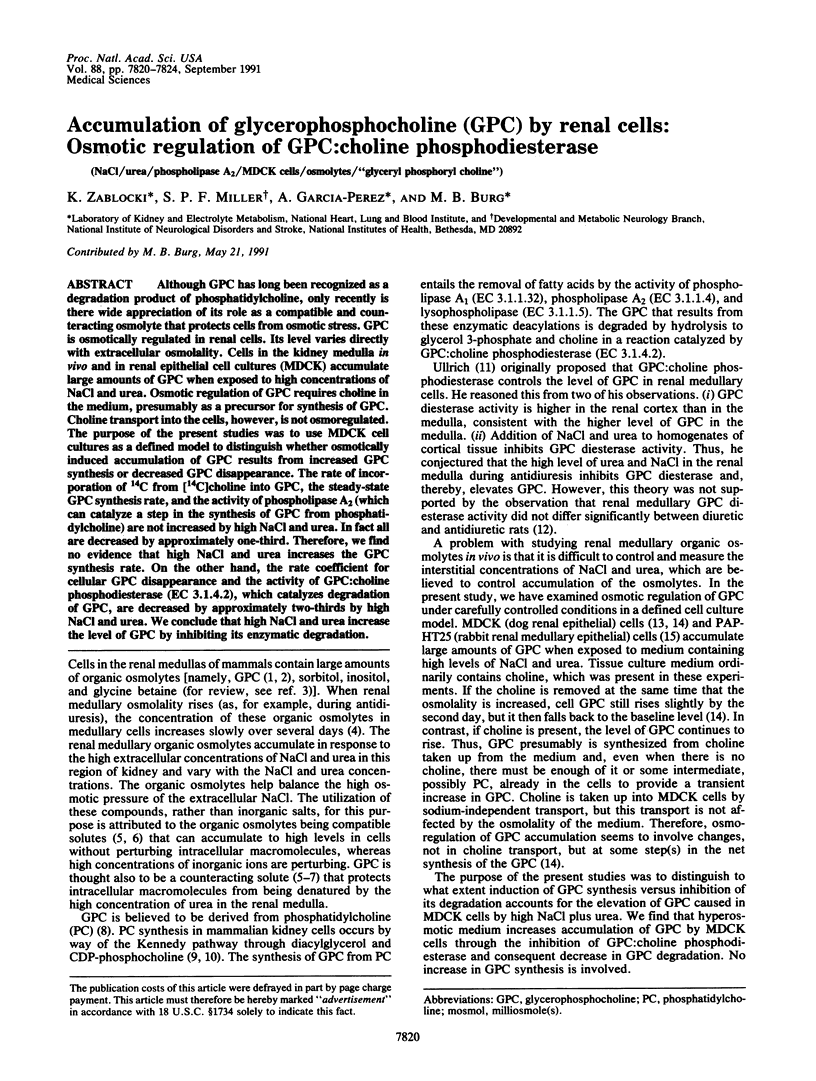
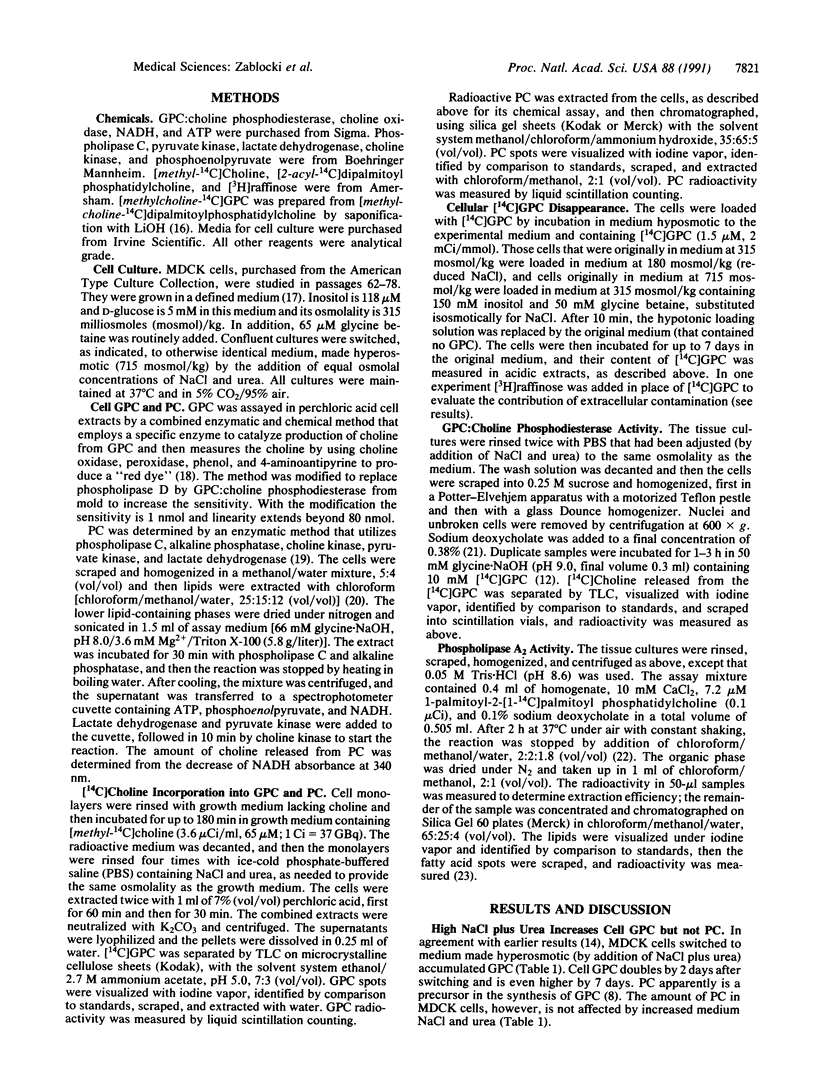
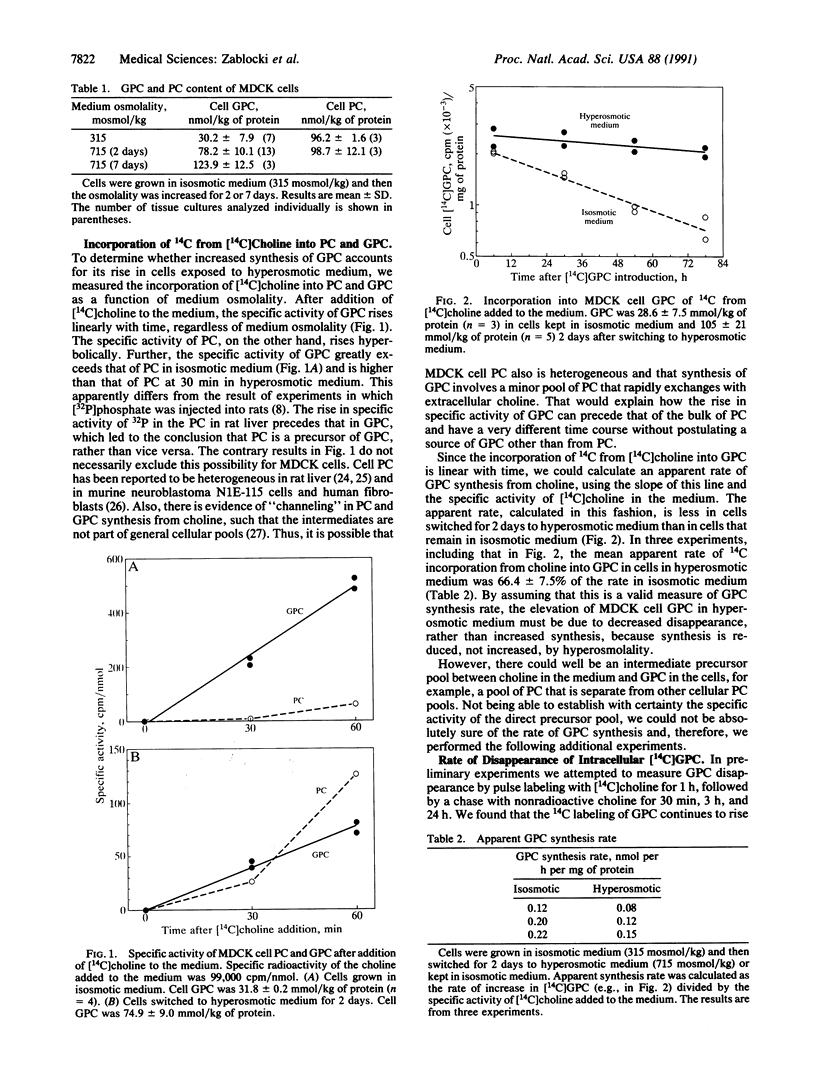
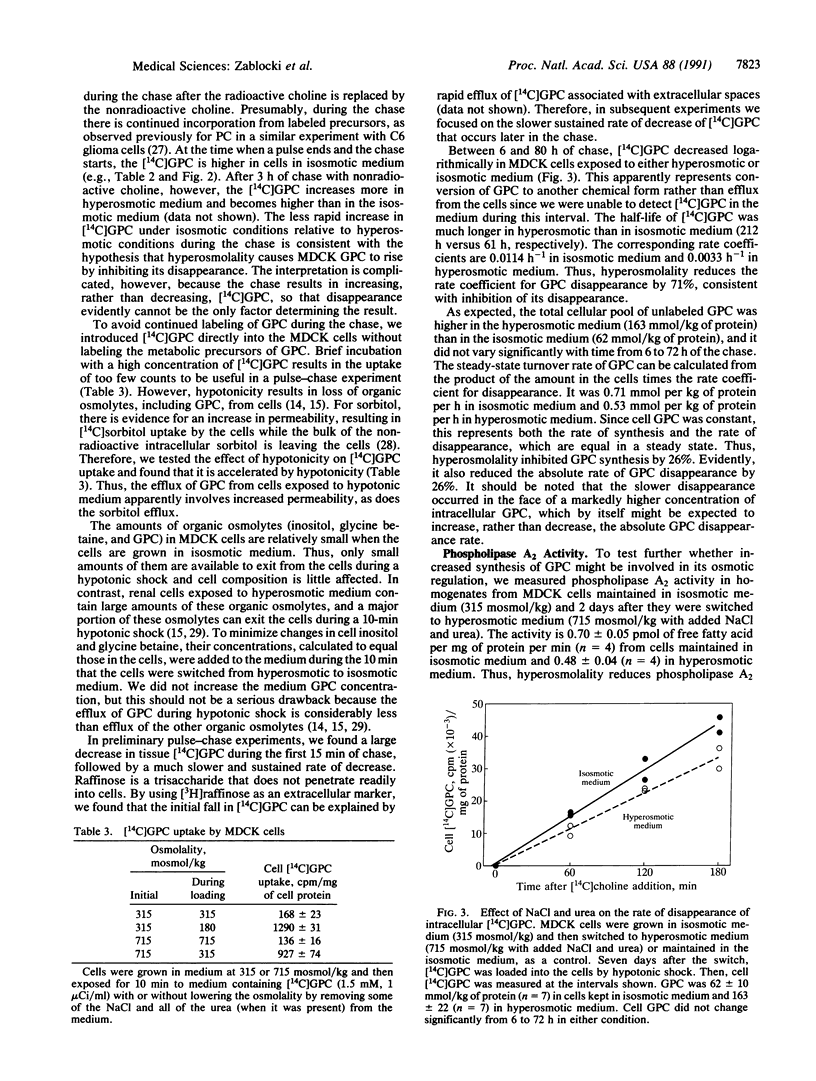
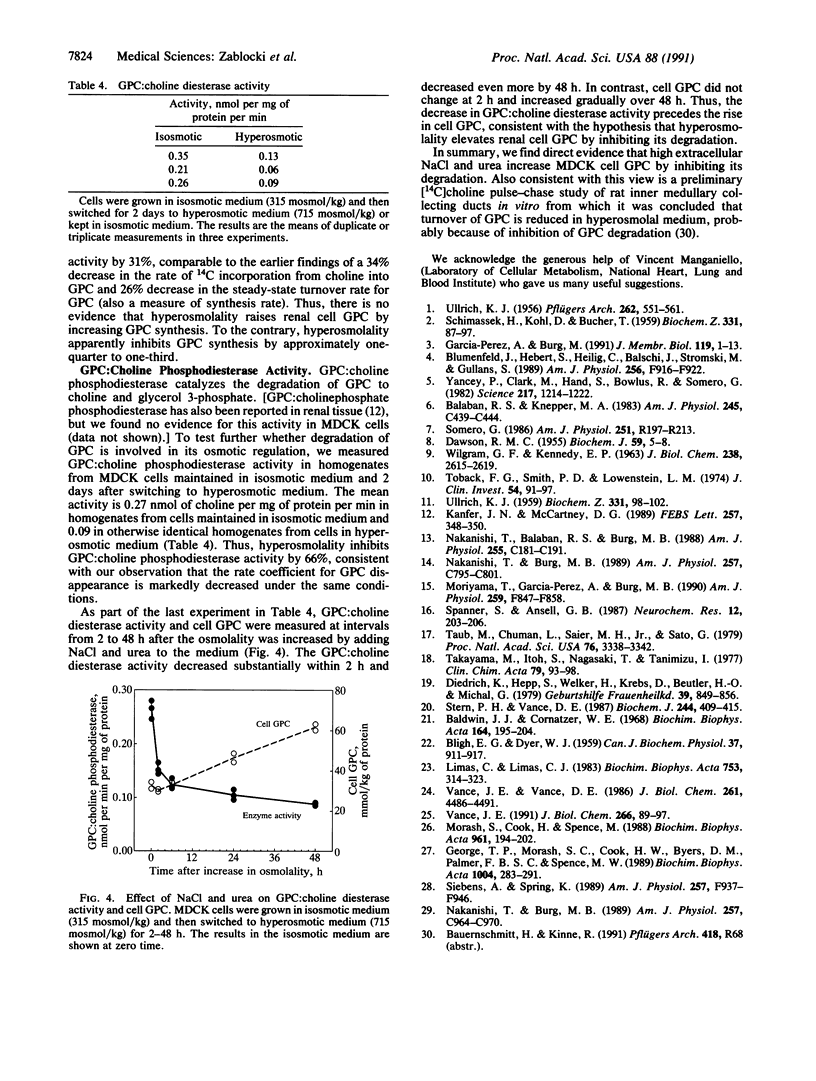
Selected References
These references are in PubMed. This may not be the complete list of references from this article.
- BLIGH E. G., DYER W. J. A rapid method of total lipid extraction and purification. Can J Biochem Physiol. 1959 Aug;37(8):911–917. doi: 10.1139/o59-099. [DOI] [PubMed] [Google Scholar]
- Balaban R. S., Knepper M. A. Nitrogen-14 nuclear magnetic resonance spectroscopy of mammalian tissues. Am J Physiol. 1983 Nov;245(5 Pt 1):C439–C444. doi: 10.1152/ajpcell.1983.245.5.C439. [DOI] [PubMed] [Google Scholar]
- Baldwin J. J., Cornatzer W. E. Rat kidney glycerylphosphorylcholine diesterase. Biochim Biophys Acta. 1968 Oct 22;164(2):195–204. doi: 10.1016/0005-2760(68)90146-x. [DOI] [PubMed] [Google Scholar]
- Blumenfeld J. D., Hebert S. C., Heilig C. W., Balschi J. A., Stromski M. E., Gullans S. R. Organic osmolytes in inner medulla of Brattleboro rat: effects of ADH and dehydration. Am J Physiol. 1989 May;256(5 Pt 2):F916–F922. doi: 10.1152/ajprenal.1989.256.5.F916. [DOI] [PubMed] [Google Scholar]
- DAWSON R. M. The role of glycerylphosphorylcholine and glycerylphosphorylethanolamine in liver phospholipid metabolism. Biochem J. 1955 Jan;59(1):5–8. doi: 10.1042/bj0590005. [DOI] [PMC free article] [PubMed] [Google Scholar]
- Diedrich K., Hepp S., Welker H., Krebs D., Beutler H. O., Michal G. Die enzymatische Lecithinbestimmung im Fruchtwasser zur Beurteilung der fetalen Lungenreife. Geburtshilfe Frauenheilkd. 1979 Oct;39(10):849–856. [PubMed] [Google Scholar]
- Garcia-Perez A., Burg M. B. Role of organic osmolytes in adaptation of renal cells to high osmolality. J Membr Biol. 1991 Jan;119(1):1–13. doi: 10.1007/BF01868535. [DOI] [PubMed] [Google Scholar]
- George T. P., Morash S. C., Cook H. W., Byers D. M., Palmer F. B., Spence M. W. Phosphatidylcholine biosynthesis in cultured glioma cells: evidence for channeling of intermediates. Biochim Biophys Acta. 1989 Aug 22;1004(3):283–291. doi: 10.1016/0005-2760(89)90075-1. [DOI] [PubMed] [Google Scholar]
- Kanfer J. N., McCartney D. G. GPC phosphodiesterase and phosphomonoesterase activities of renal cortex and medulla of control, antidiuresis and diuresis rats. FEBS Lett. 1989 Nov 6;257(2):348–350. doi: 10.1016/0014-5793(89)81568-6. [DOI] [PubMed] [Google Scholar]
- Limas C., Limas C. J. Phospholipid metabolism in the rat renal inner medulla. Biochim Biophys Acta. 1983 Oct 11;753(3):314–323. doi: 10.1016/0005-2760(83)90054-1. [DOI] [PubMed] [Google Scholar]
- Morash S. C., Cook H. W., Spence M. W. Phosphatidylcholine metabolism in cultured cells: catabolism via glycerophosphocholine. Biochim Biophys Acta. 1988 Jul 22;961(2):194–202. doi: 10.1016/0005-2760(88)90114-2. [DOI] [PubMed] [Google Scholar]
- Moriyama T., Garcia-Perez A., Burg M. B. Factors affecting the ratio of different organic osmolytes in renal medullary cells. Am J Physiol. 1990 Nov;259(5 Pt 2):F847–F858. doi: 10.1152/ajprenal.1990.259.5.F847. [DOI] [PubMed] [Google Scholar]
- Nakanishi T., Balaban R. S., Burg M. B. Survey of osmolytes in renal cell lines. Am J Physiol. 1988 Aug;255(2 Pt 1):C181–C191. doi: 10.1152/ajpcell.1988.255.2.C181. [DOI] [PubMed] [Google Scholar]
- Nakanishi T., Burg M. B. Osmoregulation of glycerophosphorylcholine content of mammalian renal cells. Am J Physiol. 1989 Oct;257(4 Pt 1):C795–C801. doi: 10.1152/ajpcell.1989.257.4.C795. [DOI] [PubMed] [Google Scholar]
- Nakanishi T., Burg M. B. Osmoregulatory fluxes of myo-inositol and betaine in renal cells. Am J Physiol. 1989 Nov;257(5 Pt 1):C964–C970. doi: 10.1152/ajpcell.1989.257.5.C964. [DOI] [PubMed] [Google Scholar]
- PEHLING G., ULLRICH K. J. Uber das Vorkommen von Phosphorverbindungen in verschiedenen Nierenabschnitten und Anderungen ihrer Konzentration in Abhängigkeit vom Diuresezustand. Pflugers Arch. 1956;262(6):551–561. doi: 10.1007/BF00362117. [DOI] [PubMed] [Google Scholar]
- Siebens A. W., Spring K. R. A novel sorbitol transport mechanism in cultured renal papillary epithelial cells. Am J Physiol. 1989 Dec;257(6 Pt 2):F937–F946. doi: 10.1152/ajprenal.1989.257.6.F937. [DOI] [PubMed] [Google Scholar]
- Somero G. N. Protons, osmolytes, and fitness of internal milieu for protein function. Am J Physiol. 1986 Aug;251(2 Pt 2):R197–R213. doi: 10.1152/ajpregu.1986.251.2.R197. [DOI] [PubMed] [Google Scholar]
- Spanner S., Ansell G. B. The hydrolysis of glycerophosphocholine by rat brain microsomes: activation and inhibition. Neurochem Res. 1987 Feb;12(2):203–206. doi: 10.1007/BF00979538. [DOI] [PubMed] [Google Scholar]
- Stern P. H., Vance D. E. Phosphatidylcholine metabolism in neonatal mouse calvaria. Biochem J. 1987 Jun 1;244(2):409–415. doi: 10.1042/bj2440409. [DOI] [PMC free article] [PubMed] [Google Scholar]
- Takayama M., Itoh S., Nagasaki T., Tanimizu I. A new enzymatic method for determination of serum choline-containing phospholipids. Clin Chim Acta. 1977 Aug 15;79(1):93–98. doi: 10.1016/0009-8981(77)90465-x. [DOI] [PubMed] [Google Scholar]
- Taub M., Chuman L., Saier M. H., Jr, Sato G. Growth of Madin-Darby canine kidney epithelial cell (MDCK) line in hormone-supplemented, serum-free medium. Proc Natl Acad Sci U S A. 1979 Jul;76(7):3338–3342. doi: 10.1073/pnas.76.7.3338. [DOI] [PMC free article] [PubMed] [Google Scholar]
- Toback F. G., Smith P. D., Lowenstein L. M. Phospholipid metabolism in the initiation of renal compensatory growth after acute reduction of renal mass. J Clin Invest. 1974 Jul;54(1):91–97. doi: 10.1172/JCI107754. [DOI] [PMC free article] [PubMed] [Google Scholar]
- Vance J. E. Newly made phosphatidylserine and phosphatidylethanolamine are preferentially translocated between rat liver mitochondria and endoplasmic reticulum. J Biol Chem. 1991 Jan 5;266(1):89–97. [PubMed] [Google Scholar]
- Vance J. E., Vance D. E. Specific pools of phospholipids are used for lipoprotein secretion by cultured rat hepatocytes. J Biol Chem. 1986 Apr 5;261(10):4486–4491. [PubMed] [Google Scholar]
- WILGRAM G. F., KENNEDY E. P. INTRACELLULAR DISTRIBUTION OF SOME ENZYMES CATALYZING REACTIONS IN THE BIOSYNTHESIS OF COMPLEX LIPIDS. J Biol Chem. 1963 Aug;238:2615–2619. [PubMed] [Google Scholar]
- Yancey P. H., Clark M. E., Hand S. C., Bowlus R. D., Somero G. N. Living with water stress: evolution of osmolyte systems. Science. 1982 Sep 24;217(4566):1214–1222. doi: 10.1126/science.7112124. [DOI] [PubMed] [Google Scholar]