Abstract
Sequence segments of about 140 amino acids in length, each containing a selected consensus region, were used in alignments of the aminoacyl-tRNA synthetases with the aim of discerning their evolutionary relationships. In all cases tested, enzymes specific for the same amino acid from a variety of organisms grouped together, reinforcing the supposition that the aminoacyl-tRNA synthetases are very ancient enzymes that evolved to include the full complement of 20 amino acids long before the divergence leading to prokaryotes and eukaryotes. The enzymes are divided into two mutually exclusive groups that appear to have evolved from independent roots. Group I, for which two sequence segments were analyzed, contains the enzymes specific for glutamic acid, glutamine, tryptophan, tyrosine, valine, leucine, isoleucine, methionine, and arginine. Group II enzymes include those activating threonine, proline, serine, lysine, aspartic acid, asparagine, histidine, alanine, glycine, and phenylalanine. Both groups contain a spectrum of amino acid types, suggesting the possibility that each could have once supported an independent system for protein synthesis. Within each group, enzymes specific for chemically similar amino acids tend to cluster together, indicating that a major theme of synthetase evolution involved the adaptation of binding sites to accommodate related amino acids with subsequent specialization to a single amino acid. In a few cases, however, synthetases activating dissimilar amino acids are grouped together.
Full text
PDF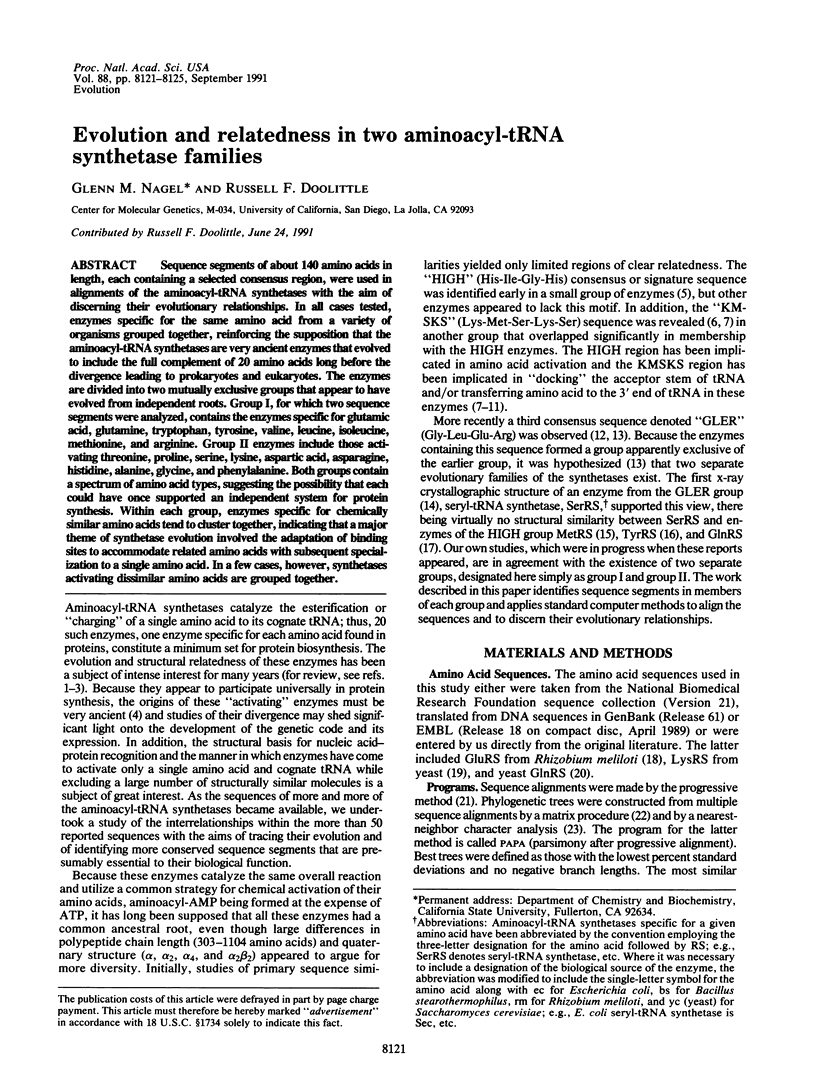
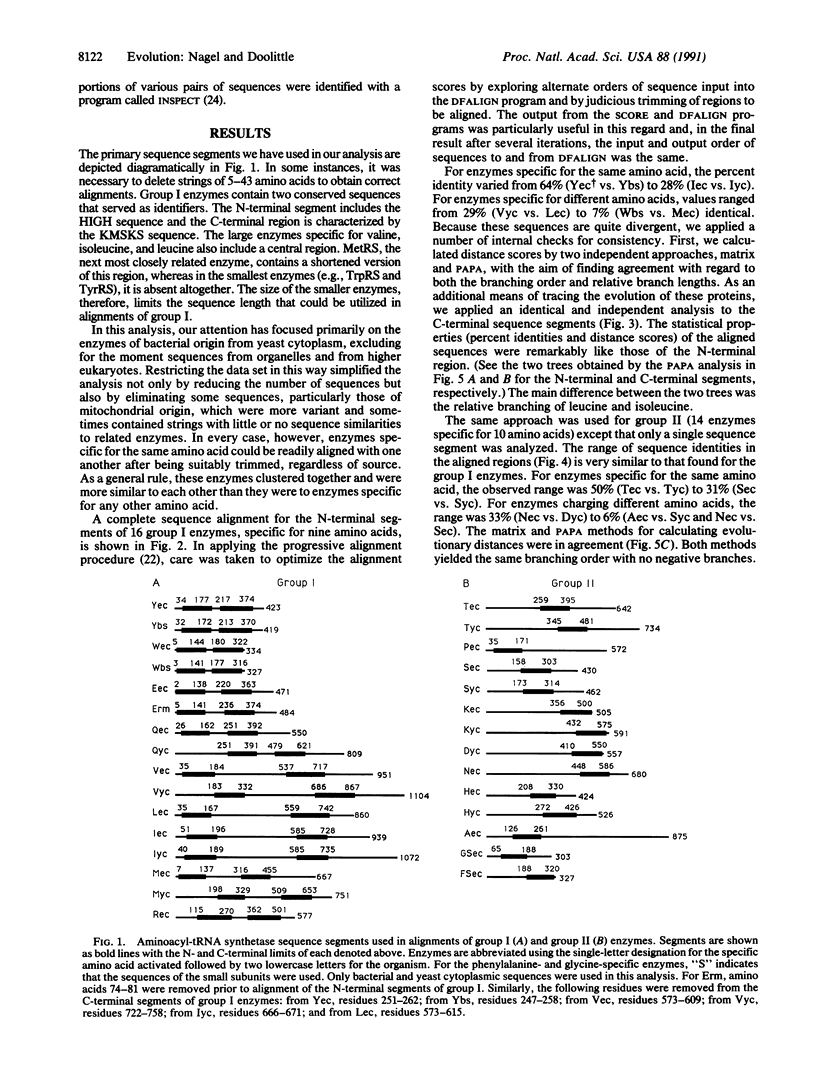
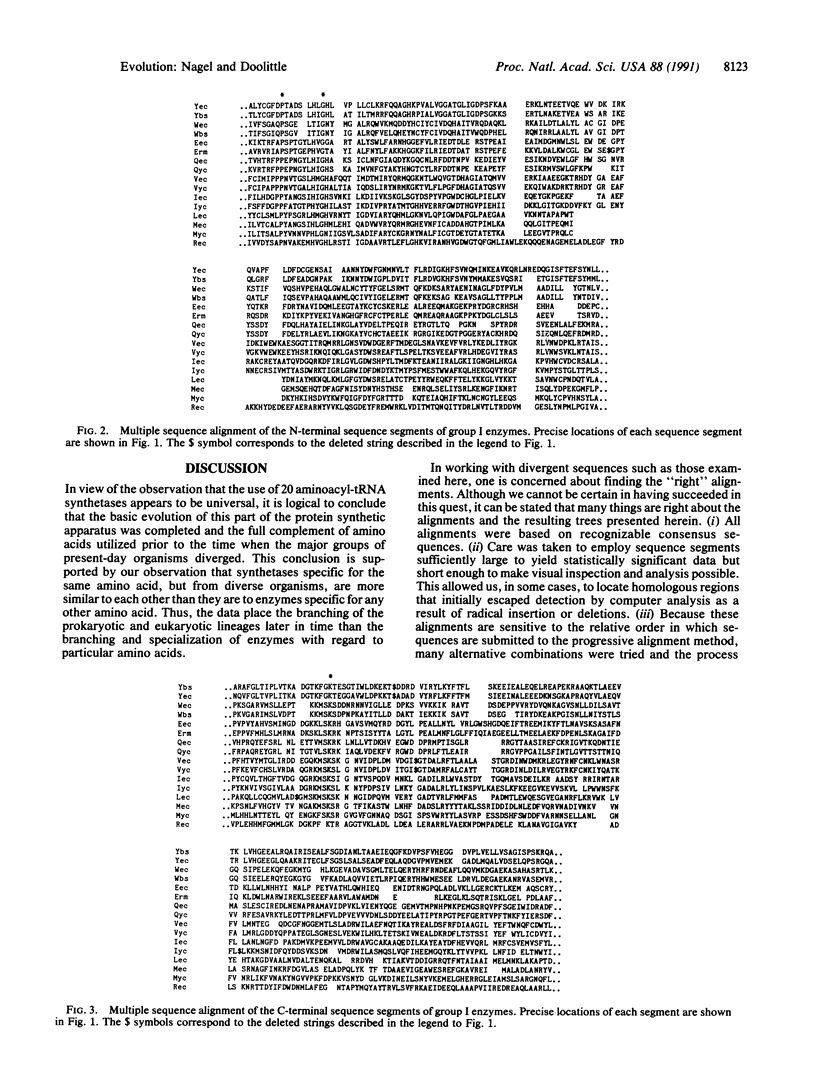
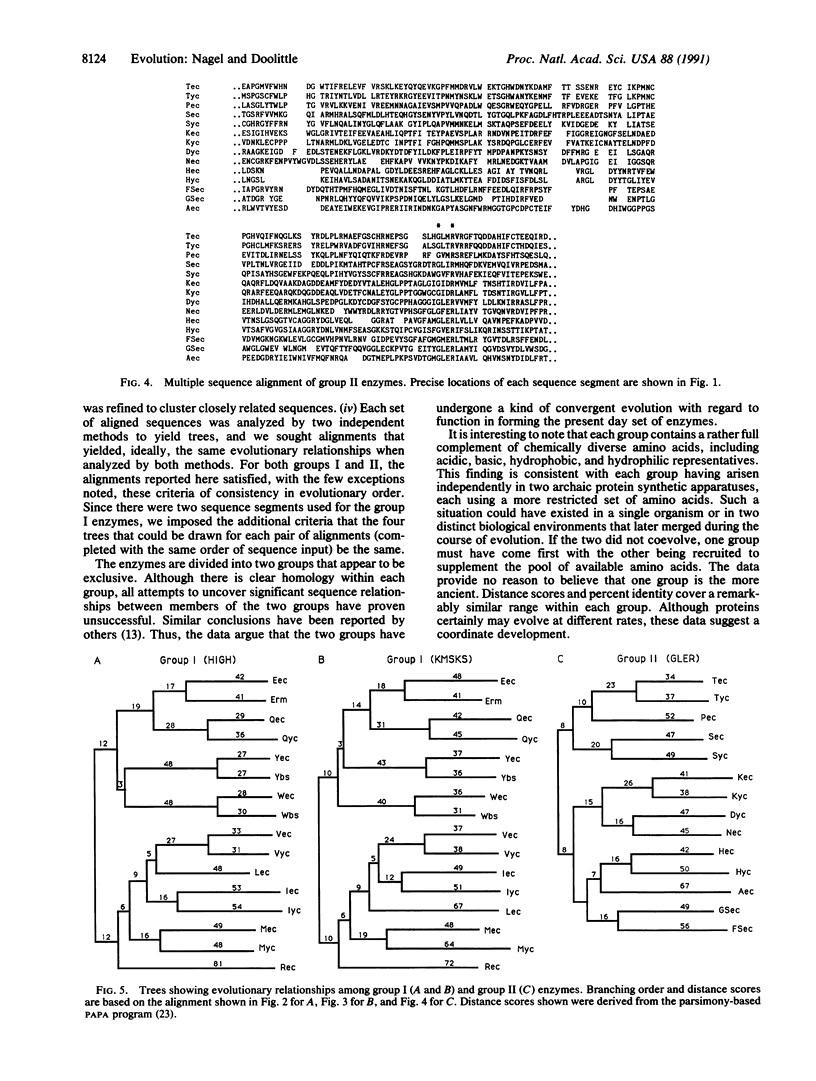
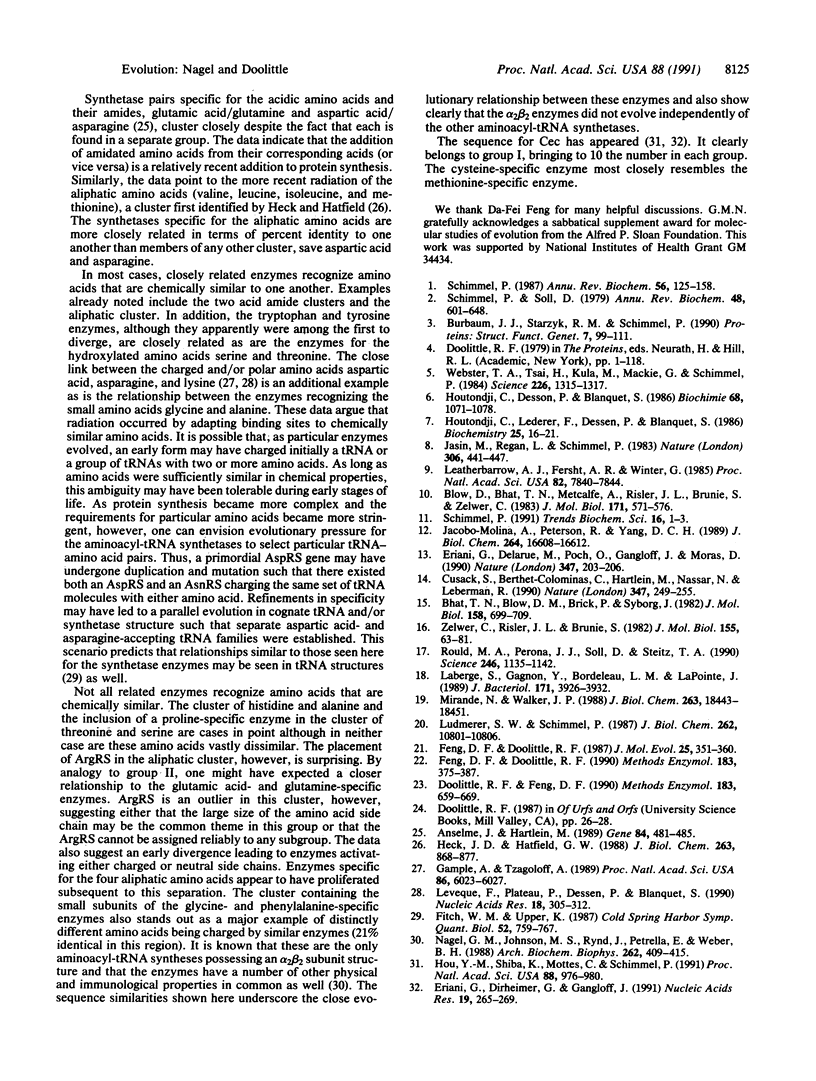
Selected References
These references are in PubMed. This may not be the complete list of references from this article.
- Anselme J., Härtlein M. Asparaginyl-tRNA synthetase from Escherichia coli has significant sequence homologies with yeast aspartyl-tRNA synthetase. Gene. 1989 Dec 14;84(2):481–485. doi: 10.1016/0378-1119(89)90524-6. [DOI] [PubMed] [Google Scholar]
- Bhat T. N., Blow D. M., Brick P., Nyborg J. Tyrosyl-tRNA synthetase forms a mononucleotide-binding fold. J Mol Biol. 1982 Jul 15;158(4):699–709. doi: 10.1016/0022-2836(82)90255-8. [DOI] [PubMed] [Google Scholar]
- Blow D. M., Bhat T. N., Metcalfe A., Risler J. L., Brunie S., Zelwer C. Structural homology in the amino-terminal domains of two aminoacyl-tRNA synthetases. J Mol Biol. 1983 Dec 25;171(4):571–576. doi: 10.1016/0022-2836(83)90044-x. [DOI] [PubMed] [Google Scholar]
- Burbaum J. J., Starzyk R. M., Schimmel P. Understanding structural relationships in proteins of unsolved three-dimensional structure. Proteins. 1990;7(2):99–111. doi: 10.1002/prot.340070202. [DOI] [PubMed] [Google Scholar]
- Cusack S., Berthet-Colominas C., Härtlein M., Nassar N., Leberman R. A second class of synthetase structure revealed by X-ray analysis of Escherichia coli seryl-tRNA synthetase at 2.5 A. Nature. 1990 Sep 20;347(6290):249–255. doi: 10.1038/347249a0. [DOI] [PubMed] [Google Scholar]
- Doolittle R. F., Feng D. F. Nearest neighbor procedure for relating progressively aligned amino acid sequences. Methods Enzymol. 1990;183:659–669. doi: 10.1016/0076-6879(90)83043-9. [DOI] [PubMed] [Google Scholar]
- Eriani G., Delarue M., Poch O., Gangloff J., Moras D. Partition of tRNA synthetases into two classes based on mutually exclusive sets of sequence motifs. Nature. 1990 Sep 13;347(6289):203–206. doi: 10.1038/347203a0. [DOI] [PubMed] [Google Scholar]
- Eriani G., Dirheimer G., Gangloff J. Cysteinyl-tRNA synthetase: determination of the last E. coli aminoacyl-tRNA synthetase primary structure. Nucleic Acids Res. 1991 Jan 25;19(2):265–269. doi: 10.1093/nar/19.2.265. [DOI] [PMC free article] [PubMed] [Google Scholar]
- Feng D. F., Doolittle R. F. Progressive alignment and phylogenetic tree construction of protein sequences. Methods Enzymol. 1990;183:375–387. doi: 10.1016/0076-6879(90)83025-5. [DOI] [PubMed] [Google Scholar]
- Feng D. F., Doolittle R. F. Progressive sequence alignment as a prerequisite to correct phylogenetic trees. J Mol Evol. 1987;25(4):351–360. doi: 10.1007/BF02603120. [DOI] [PubMed] [Google Scholar]
- Fitch W. M., Upper K. The phylogeny of tRNA sequences provides evidence for ambiguity reduction in the origin of the genetic code. Cold Spring Harb Symp Quant Biol. 1987;52:759–767. doi: 10.1101/sqb.1987.052.01.085. [DOI] [PubMed] [Google Scholar]
- Gampel A., Tzagoloff A. Homology of aspartyl- and lysyl-tRNA synthetases. Proc Natl Acad Sci U S A. 1989 Aug;86(16):6023–6027. doi: 10.1073/pnas.86.16.6023. [DOI] [PMC free article] [PubMed] [Google Scholar]
- Heck J. D., Hatfield G. W. Valyl-tRNA synthetase gene of Escherichia coli K12. Primary structure and homology within a family of aminoacyl-TRNA synthetases. J Biol Chem. 1988 Jan 15;263(2):868–877. [PubMed] [Google Scholar]
- Hou Y. M., Shiba K., Mottes C., Schimmel P. Sequence determination and modeling of structural motifs for the smallest monomeric aminoacyl-tRNA synthetase. Proc Natl Acad Sci U S A. 1991 Feb 1;88(3):976–980. doi: 10.1073/pnas.88.3.976. [DOI] [PMC free article] [PubMed] [Google Scholar]
- Hountondji C., Dessen P., Blanquet S. Sequence similarities among the family of aminoacyl-tRNA synthetases. Biochimie. 1986 Sep;68(9):1071–1078. doi: 10.1016/s0300-9084(86)80181-x. [DOI] [PubMed] [Google Scholar]
- Hountondji C., Lederer F., Dessen P., Blanquet S. Escherichia coli tyrosyl- and methionyl-tRNA synthetases display sequence similarity at the binding site for the 3'-end of tRNA. Biochemistry. 1986 Jan 14;25(1):16–21. doi: 10.1021/bi00349a003. [DOI] [PubMed] [Google Scholar]
- Jacobo-Molina A., Peterson R., Yang D. C. cDNA sequence, predicted primary structure, and evolving amphiphilic helix of human aspartyl-tRNA synthetase. J Biol Chem. 1989 Oct 5;264(28):16608–16612. [PubMed] [Google Scholar]
- Jasin M., Regan L., Schimmel P. Modular arrangement of functional domains along the sequence of an aminoacyl tRNA synthetase. Nature. 1983 Dec 1;306(5942):441–447. doi: 10.1038/306441a0. [DOI] [PubMed] [Google Scholar]
- Laberge S., Gagnon Y., Bordeleau L. M., Lapointe J. Cloning and sequencing of the gltX gene, encoding the glutamyl-tRNA synthetase of Rhizobium meliloti A2. J Bacteriol. 1989 Jul;171(7):3926–3932. doi: 10.1128/jb.171.7.3926-3932.1989. [DOI] [PMC free article] [PubMed] [Google Scholar]
- Leatherbarrow R. J., Fersht A. R., Winter G. Transition-state stabilization in the mechanism of tyrosyl-tRNA synthetase revealed by protein engineering. Proc Natl Acad Sci U S A. 1985 Dec;82(23):7840–7844. doi: 10.1073/pnas.82.23.7840. [DOI] [PMC free article] [PubMed] [Google Scholar]
- Ludmerer S. W., Schimmel P. Gene for yeast glutamine tRNA synthetase encodes a large amino-terminal extension and provides a strong confirmation of the signature sequence for a group of the aminoacyl-tRNA synthetases. J Biol Chem. 1987 Aug 5;262(22):10801–10806. [PubMed] [Google Scholar]
- Lévêque F., Plateau P., Dessen P., Blanquet S. Homology of lysS and lysU, the two Escherichia coli genes encoding distinct lysyl-tRNA synthetase species. Nucleic Acids Res. 1990 Jan 25;18(2):305–312. doi: 10.1093/nar/18.2.305. [DOI] [PMC free article] [PubMed] [Google Scholar]
- Mirande M., Waller J. P. The yeast lysyl-tRNA synthetase gene. Evidence for general amino acid control of its expression and domain structure of the encoded protein. J Biol Chem. 1988 Dec 5;263(34):18443–18451. [PubMed] [Google Scholar]
- Nagel G. M., Johnson M. S., Rynd J., Petrella E., Weber B. H. Glycyl-tRNA synthetase of Escherichia coli: immunological homology with phenylalanyl-tRNA synthetase. Arch Biochem Biophys. 1988 May 1;262(2):409–415. doi: 10.1016/0003-9861(88)90391-8. [DOI] [PubMed] [Google Scholar]
- Rould M. A., Perona J. J., Söll D., Steitz T. A. Structure of E. coli glutaminyl-tRNA synthetase complexed with tRNA(Gln) and ATP at 2.8 A resolution. Science. 1989 Dec 1;246(4934):1135–1142. doi: 10.1126/science.2479982. [DOI] [PubMed] [Google Scholar]
- Schimmel P. R., Söll D. Aminoacyl-tRNA synthetases: general features and recognition of transfer RNAs. Annu Rev Biochem. 1979;48:601–648. doi: 10.1146/annurev.bi.48.070179.003125. [DOI] [PubMed] [Google Scholar]
- Schimmel P. Aminoacyl tRNA synthetases: general scheme of structure-function relationships in the polypeptides and recognition of transfer RNAs. Annu Rev Biochem. 1987;56:125–158. doi: 10.1146/annurev.bi.56.070187.001013. [DOI] [PubMed] [Google Scholar]
- Schimmel P. Classes of aminoacyl-tRNA synthetases and the establishment of the genetic code. Trends Biochem Sci. 1991 Jan;16(1):1–3. doi: 10.1016/0968-0004(91)90002-d. [DOI] [PubMed] [Google Scholar]
- Webster T., Tsai H., Kula M., Mackie G. A., Schimmel P. Specific sequence homology and three-dimensional structure of an aminoacyl transfer RNA synthetase. Science. 1984 Dec 14;226(4680):1315–1317. doi: 10.1126/science.6390679. [DOI] [PubMed] [Google Scholar]
- Zelwer C., Risler J. L., Brunie S. Crystal structure of Escherichia coli methionyl-tRNA synthetase at 2.5 A resolution. J Mol Biol. 1982 Feb 15;155(1):63–81. doi: 10.1016/0022-2836(82)90492-2. [DOI] [PubMed] [Google Scholar]