Abstract
The acetogen Clostridium thermoaceticum generates growth-essential CO2 equivalents from carboxylated aromatic compounds (e.g., 4-hydroxybenzoate), and these CO2 equivalents are likely integrated into the acetogenic pathway (T. Hsu, S. L. Daniel, M. F. Lux, and H. L. Drake, J. Bacteriol. 172:212-217, 1990). By using 4-hydroxybenzoate as a model substrate, an assay was developed to study the expression and activity of the decarboxylase involved in the activation of aromatic carboxyl groups. The aromatic-dependent decarboxylase was induced by carboxylated aromatic compounds in the early stages of growth and was not repressed by glucose or other acetogenic substrates; nonutilizable carboxylated aromatic compounds did not induce the decarboxylase. The decarboxylase activity displayed saturation kinetics at both whole-cell and cell extract levels, was sensitive to oxidation, and was not affected by exogenous energy sources. However, at the whole-cell level, metabolic inhibitors decreased the decarboxylase activity. Supplemental biotin or avidin did not significantly affect decarboxylation. The aromatic-dependent decarboxylase was specific for benzoates with a hydroxyl group in the para position of the aromatic ring; the meta position could be occupied by various substituent groups (-H, -OH, -OCH3, -Cl, or -F). The carboxyl carbon from [carboxyl-14C] vanillate went primarily to 14CO2 in short-term decarboxylase assays. During growth, the aromatic carboxyl group went primarily to CO2 under CO2-enriched conditions. However, under CO2-limited conditions, the aromatic carboxyl carbon went nearly totally to acetate, with equal distribution between the carboxyl and methyl carbons, thus demonstrating that acetate could be totally synthesized from aromatic carboxyl groups. In contrast, when cocultivated (i.e., supplemented) with CO under CO2-limited conditions, the aromatic carboxyl group went primarily to the methyl carbon of acetate.
Full text
PDF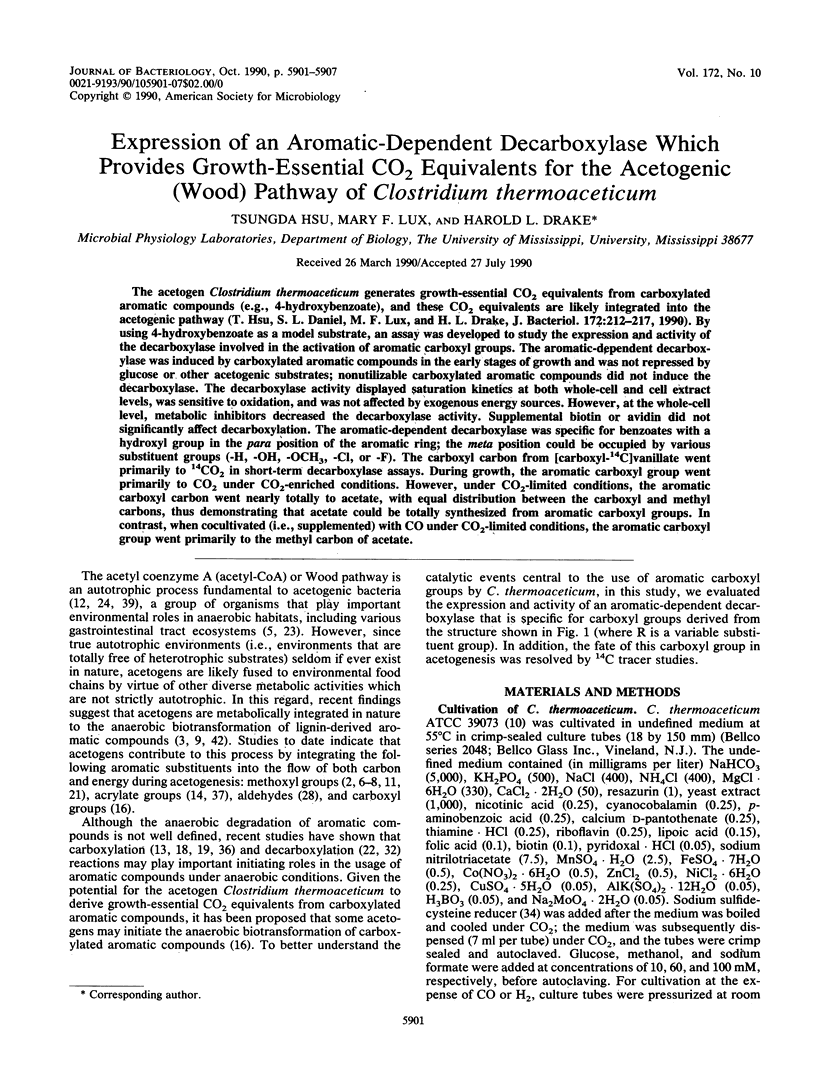
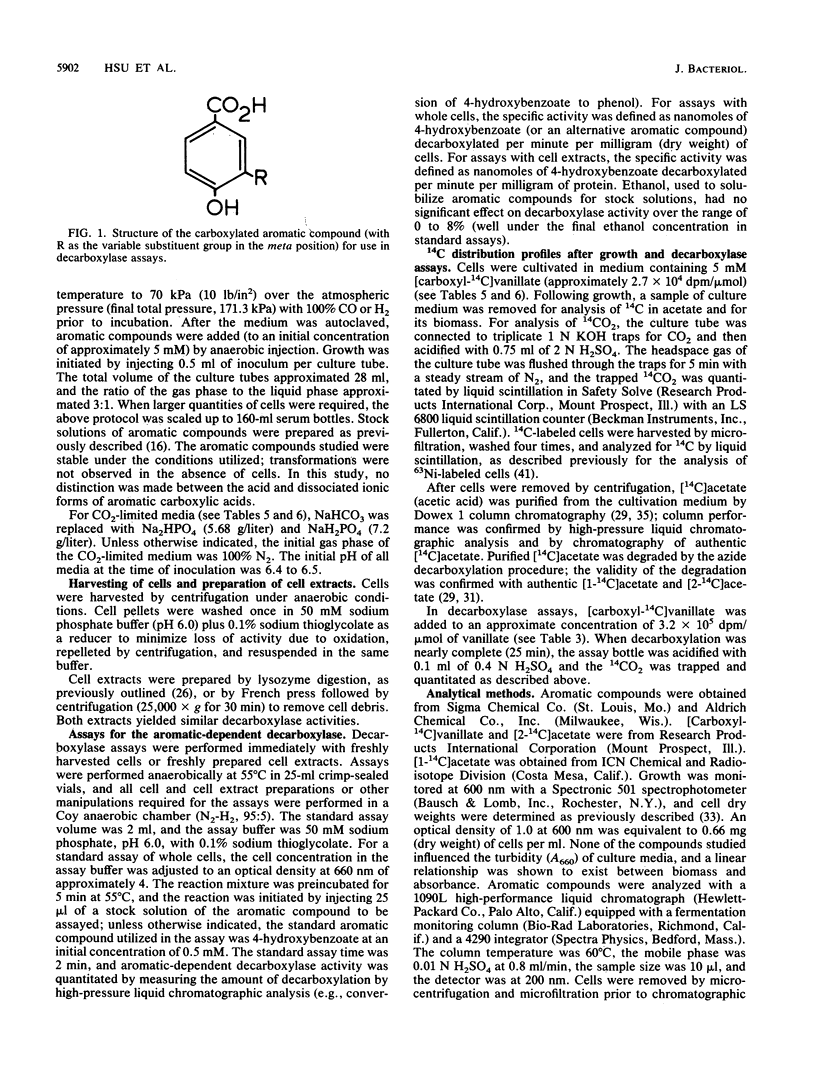
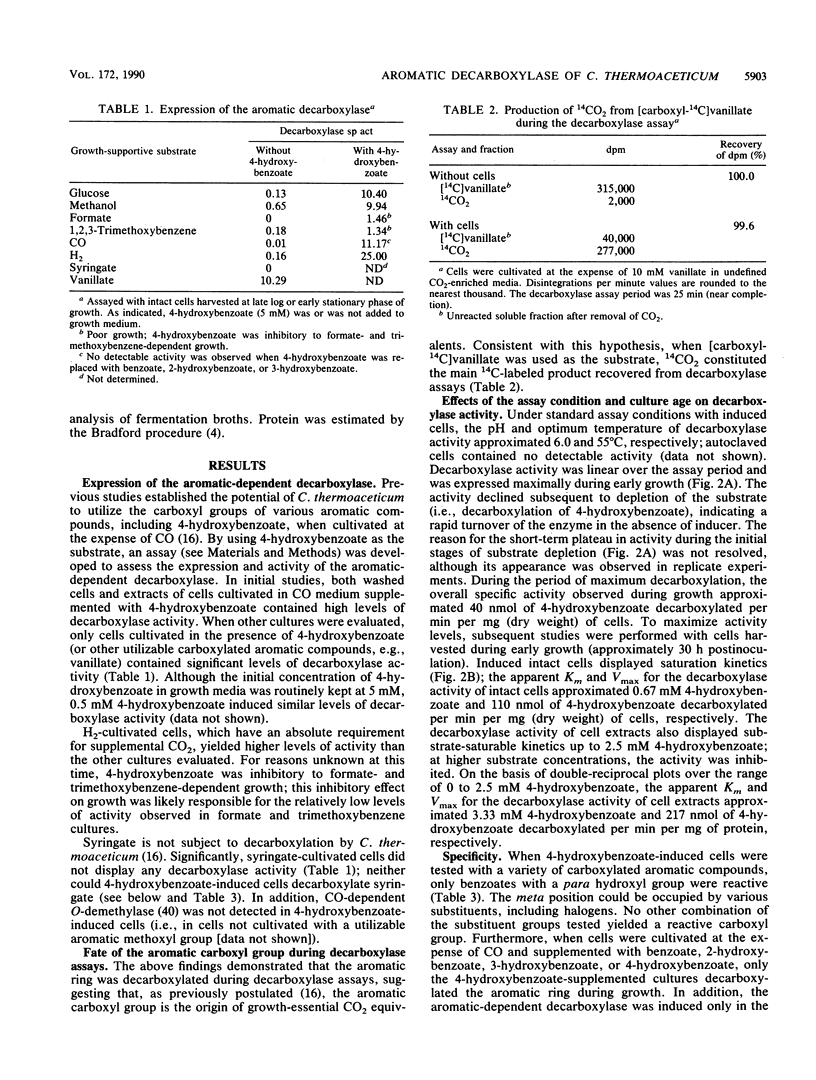
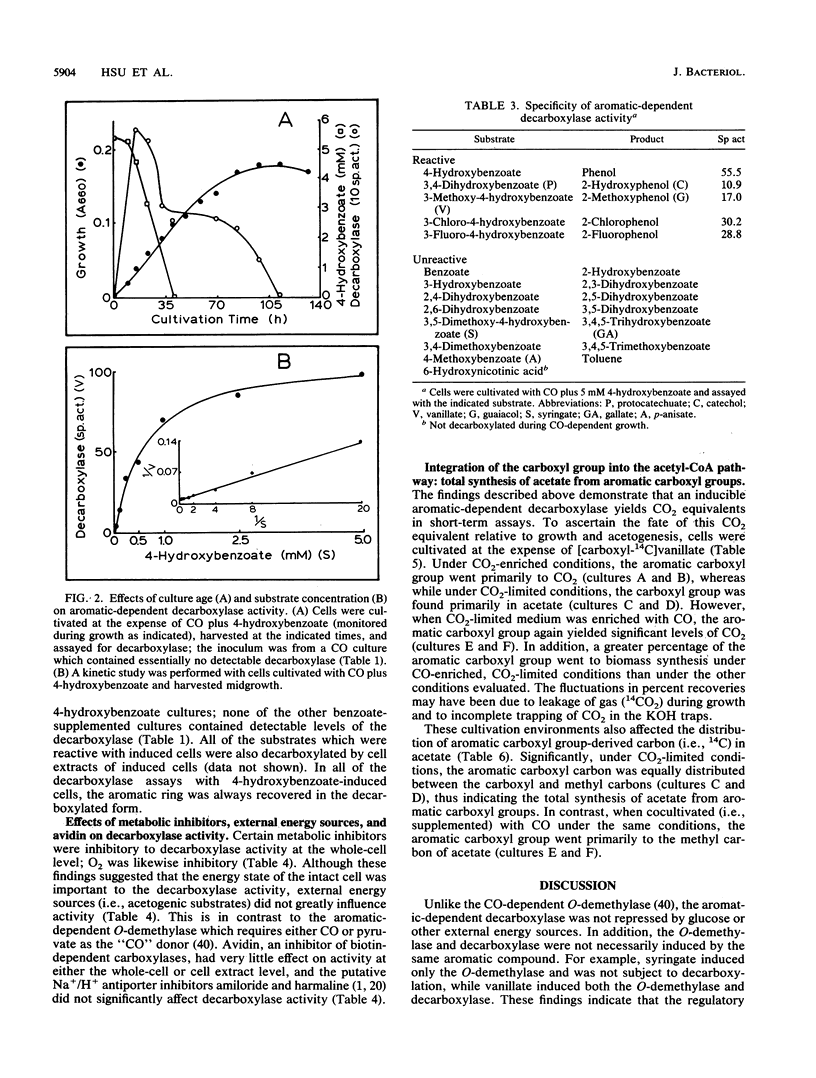
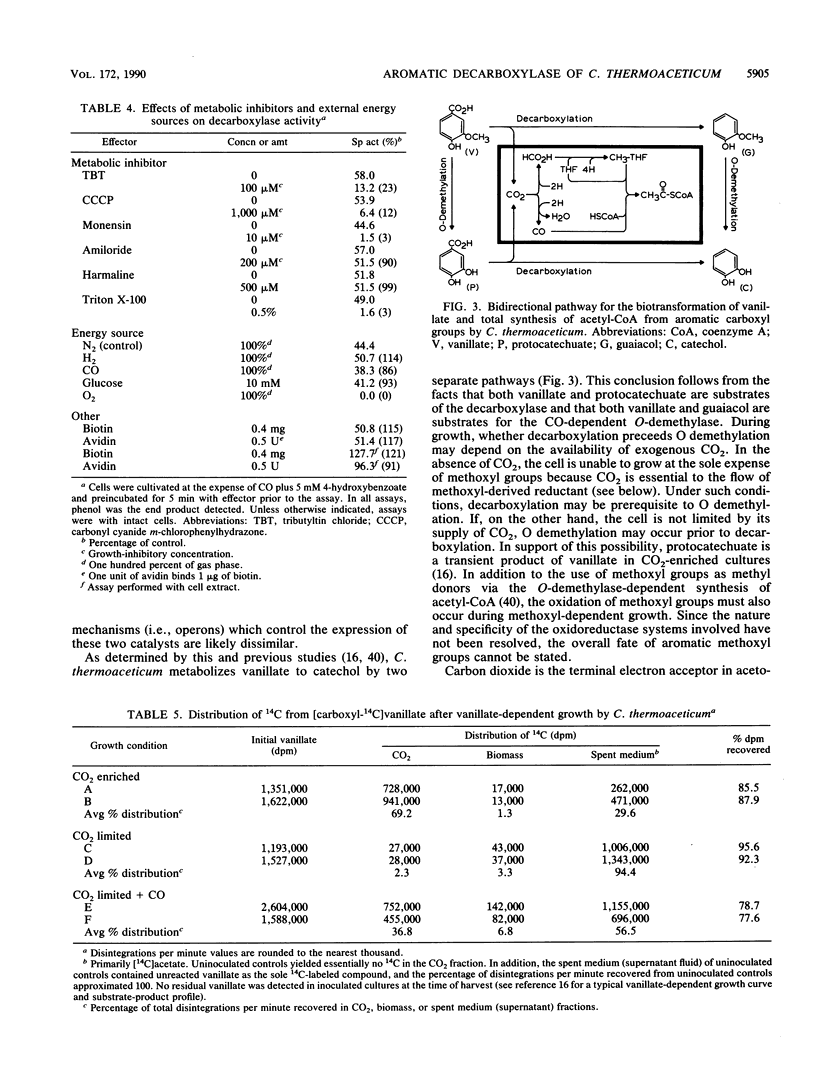
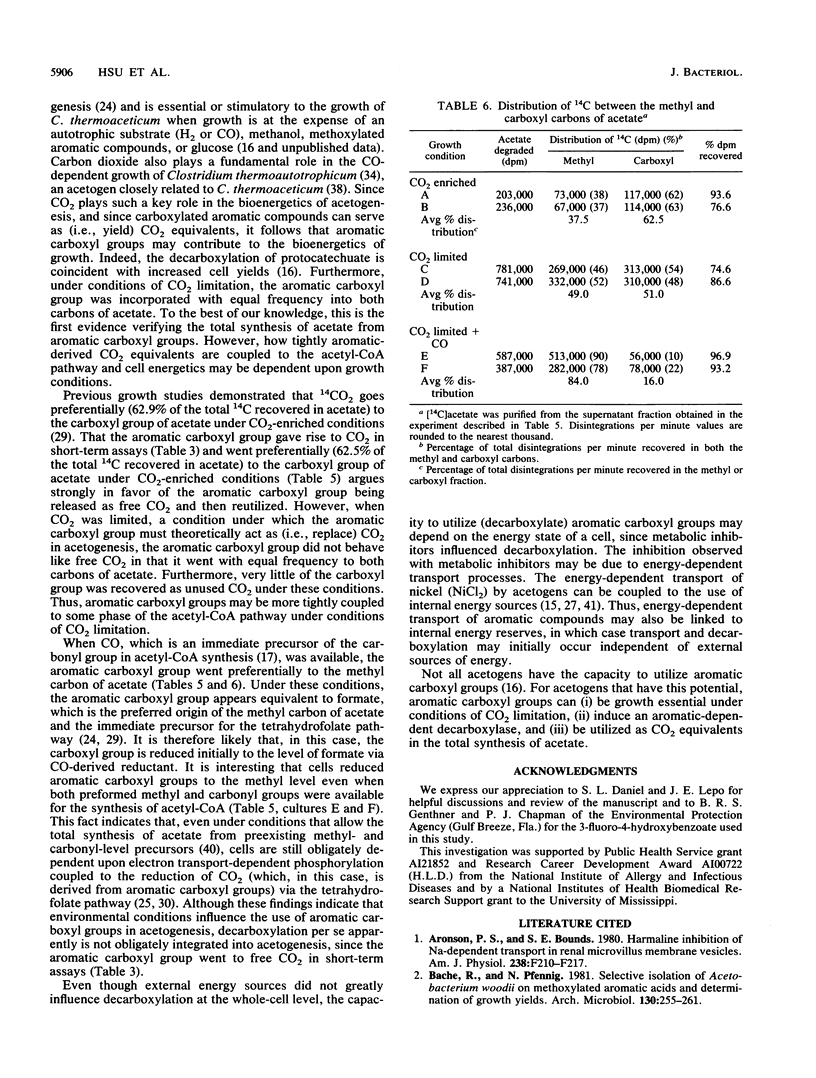
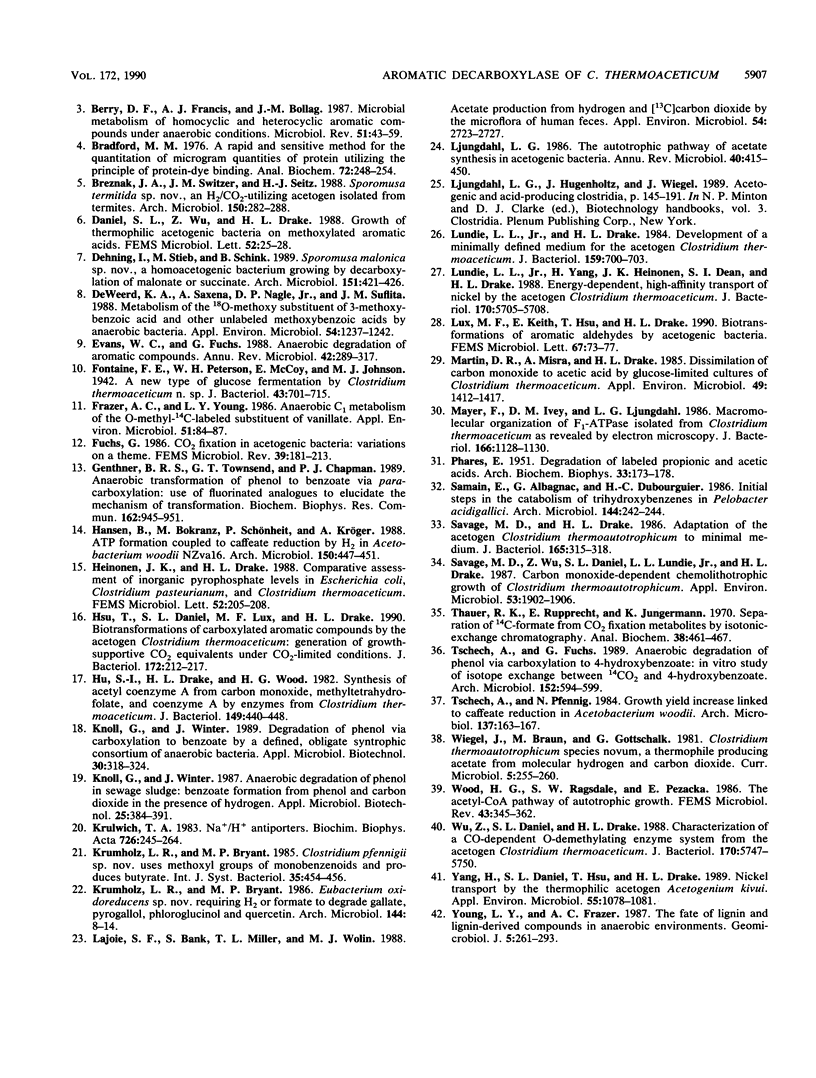
Selected References
These references are in PubMed. This may not be the complete list of references from this article.
- Aronson P. S., Bounds S. E. Harmaline inhibition of Na-dependent transport in renal microvillus membrane vesicles. Am J Physiol. 1980 Mar;238(3):F210–F217. doi: 10.1152/ajprenal.1980.238.3.F210. [DOI] [PubMed] [Google Scholar]
- Berry D. F., Francis A. J., Bollag J. M. Microbial metabolism of homocyclic and heterocyclic aromatic compounds under anaerobic conditions. Microbiol Rev. 1987 Mar;51(1):43–59. doi: 10.1128/mr.51.1.43-59.1987. [DOI] [PMC free article] [PubMed] [Google Scholar]
- Bradford M. M. A rapid and sensitive method for the quantitation of microgram quantities of protein utilizing the principle of protein-dye binding. Anal Biochem. 1976 May 7;72:248–254. doi: 10.1006/abio.1976.9999. [DOI] [PubMed] [Google Scholar]
- DeWeerd K. A., Saxena A., Nagle D. P., Jr, Suflita J. M. Metabolism of the 18O-methoxy substituent of 3-methoxybenzoic acid and other unlabeled methoxybenzoic acids by anaerobic bacteria. Appl Environ Microbiol. 1988 May;54(5):1237–1242. doi: 10.1128/aem.54.5.1237-1242.1988. [DOI] [PMC free article] [PubMed] [Google Scholar]
- Evans W. C., Fuchs G. Anaerobic degradation of aromatic compounds. Annu Rev Microbiol. 1988;42:289–317. doi: 10.1146/annurev.mi.42.100188.001445. [DOI] [PubMed] [Google Scholar]
- Fontaine F. E., Peterson W. H., McCoy E., Johnson M. J., Ritter G. J. A New Type of Glucose Fermentation by Clostridium thermoaceticum. J Bacteriol. 1942 Jun;43(6):701–715. doi: 10.1128/jb.43.6.701-715.1942. [DOI] [PMC free article] [PubMed] [Google Scholar]
- Frazer A. C., Young L. Y. Anaerobic c(1) metabolism of the o-methyl-C-labeled substituent of vanillate. Appl Environ Microbiol. 1986 Jan;51(1):84–87. doi: 10.1128/aem.51.1.84-87.1986. [DOI] [PMC free article] [PubMed] [Google Scholar]
- Genthner B. R., Townsend G. T., Chapman P. J. Anaerobic transformation of phenol to benzoate via para-carboxylation: use of fluorinated analogues to elucidate the mechanism of transformation. Biochem Biophys Res Commun. 1989 Aug 15;162(3):945–951. doi: 10.1016/0006-291x(89)90764-x. [DOI] [PubMed] [Google Scholar]
- Hsu T., Daniel S. L., Lux M. F., Drake H. L. Biotransformations of carboxylated aromatic compounds by the acetogen Clostridium thermoaceticum: generation of growth-supportive CO2 equivalents under CO2-limited conditions. J Bacteriol. 1990 Jan;172(1):212–217. doi: 10.1128/jb.172.1.212-217.1990. [DOI] [PMC free article] [PubMed] [Google Scholar]
- Hu S. I., Drake H. L., Wood H. G. Synthesis of acetyl coenzyme A from carbon monoxide, methyltetrahydrofolate, and coenzyme A by enzymes from Clostridium thermoaceticum. J Bacteriol. 1982 Feb;149(2):440–448. doi: 10.1128/jb.149.2.440-448.1982. [DOI] [PMC free article] [PubMed] [Google Scholar]
- Krulwich T. A. Na+/H+ antiporters. Biochim Biophys Acta. 1983 Dec 30;726(4):245–264. doi: 10.1016/0304-4173(83)90011-3. [DOI] [PubMed] [Google Scholar]
- Lajoie S. F., Bank S., Miller T. L., Wolin M. J. Acetate production from hydrogen and [13C]carbon dioxide by the microflora of human feces. Appl Environ Microbiol. 1988 Nov;54(11):2723–2727. doi: 10.1128/aem.54.11.2723-2727.1988. [DOI] [PMC free article] [PubMed] [Google Scholar]
- Ljungdahl L. G. The autotrophic pathway of acetate synthesis in acetogenic bacteria. Annu Rev Microbiol. 1986;40:415–450. doi: 10.1146/annurev.mi.40.100186.002215. [DOI] [PubMed] [Google Scholar]
- Lundie L. L., Jr, Drake H. L. Development of a minimally defined medium for the acetogen Clostridium thermoaceticum. J Bacteriol. 1984 Aug;159(2):700–703. doi: 10.1128/jb.159.2.700-703.1984. [DOI] [PMC free article] [PubMed] [Google Scholar]
- Lundie L. L., Jr, Yang H. C., Heinonen J. K., Dean S. I., Drake H. L. Energy-dependent, high-affinity transport of nickel by the acetogen Clostridium thermoaceticum. J Bacteriol. 1988 Dec;170(12):5705–5708. doi: 10.1128/jb.170.12.5705-5708.1988. [DOI] [PMC free article] [PubMed] [Google Scholar]
- Lux M. F., Keith E., Hsu T. D., Drake H. L. Biotransformations of aromatic aldehydes by acetogenic bacteria. FEMS Microbiol Lett. 1990 Jan 15;55(1-2):73–77. doi: 10.1016/0378-1097(90)90171-l. [DOI] [PubMed] [Google Scholar]
- Martin D. R., Misra A., Drake H. L. Dissimilation of Carbon Monoxide to Acetic Acid by Glucose-Limited Cultures of Clostridium thermoaceticum. Appl Environ Microbiol. 1985 Jun;49(6):1412–1417. doi: 10.1128/aem.49.6.1412-1417.1985. [DOI] [PMC free article] [PubMed] [Google Scholar]
- Mayer F., Ivey D. M., Ljungdahl L. G. Macromolecular organization of F1-ATPase isolated from Clostridium thermoaceticum as revealed by electron microscopy. J Bacteriol. 1986 Jun;166(3):1128–1130. doi: 10.1128/jb.166.3.1128-1130.1986. [DOI] [PMC free article] [PubMed] [Google Scholar]
- PHARES E. F. Degradation of labeled propionic and acetic acids. Arch Biochem Biophys. 1951 Sep;33(2):173–178. doi: 10.1016/0003-9861(51)90094-x. [DOI] [PubMed] [Google Scholar]
- Savage M. D., Drake H. L. Adaptation of the acetogen Clostridium thermoautotrophicum to minimal medium. J Bacteriol. 1986 Jan;165(1):315–318. doi: 10.1128/jb.165.1.315-318.1986. [DOI] [PMC free article] [PubMed] [Google Scholar]
- Savage M. D., Wu Z. G., Daniel S. L., Lundie L. L., Jr, Drake H. L. Carbon monoxide-dependent chemolithotrophic growth of Clostridium thermoautotrophicum. Appl Environ Microbiol. 1987 Aug;53(8):1902–1906. doi: 10.1128/aem.53.8.1902-1906.1987. [DOI] [PMC free article] [PubMed] [Google Scholar]
- Thauer R. K., Rupprecht E., Jungermann K. Separation of 14C-formate from CO2 fixation metabolites by isoionic-exchange chromatography. Anal Biochem. 1970 Dec;38(2):461–468. doi: 10.1016/0003-2697(70)90471-9. [DOI] [PubMed] [Google Scholar]
- Wu Z. R., Daniel S. L., Drake H. L. Characterization of a CO-dependent O-demethylating enzyme system from the acetogen Clostridium thermoaceticum. J Bacteriol. 1988 Dec;170(12):5747–5750. doi: 10.1128/jb.170.12.5747-5750.1988. [DOI] [PMC free article] [PubMed] [Google Scholar]
- Yang H. C., Daniel S. L., Hsu T. D., Drake H. L. Nickel transport by the thermophilic acetogen Acetogenium kivui. Appl Environ Microbiol. 1989 May;55(5):1078–1081. doi: 10.1128/aem.55.5.1078-1081.1989. [DOI] [PMC free article] [PubMed] [Google Scholar]