Abstract
The solution conformation behavior of complex oligosaccharides was studied by resonance energy transfer, as measured by the time-resolved fluorescence method, to determine the conformational heterogeneity of a triantennary glycopeptide at various temperatures. Groups that acted as a fluorescence donor (naphthyl-2-acetyl, Nap) or acceptor (dansylethylenediamine, Dan) were selectively attached to the N terminus of the peptide and a Gal residue [either 6' (shown below), 6, or 8] of the oligosaccharide, respectively. [formula: see text] Time-resolved fluorescence energy-transfer measurements revealed two populations of conformers when Dan was attached to either Gal-6' or Gal-6. One conformer contained the antenna folded back toward the core region, and a second was in an extended conformation. The two conformations differed in donor-acceptor distance by about 10 A. Systematically increasing the temperature from 0 degrees C to 40 degrees C increased the ratio of extended to folded forms 2-fold for the Gal-6 isomer and 4-fold for the Gal-6' isomer, whereas the Gal-8 isomer showed only a single distance population throughout this temperature range. From these data, delta H and delta S for the reversible conformational change were calculated to be 3.1 kcal/mol and 10.8 cal/(mol.K) for the Gal-6 isomer and 7.1 kcal/mol and 25.8 cal/(mol.K) for the Gal-6' isomer. In addition to the structural microheterogeneity commonly associated with glycoproteins, the differential flexibilities of the different branches in the oligosaccharides contribute conformational heterogeneity and should be considered in conformational analysis. The data are discussed in terms of the most probable linkages that contribute to the observed flexibility of the individual triantennary branches, and the biological significance of flexible linkages in complex carbohydrates is considered.
Full text
PDF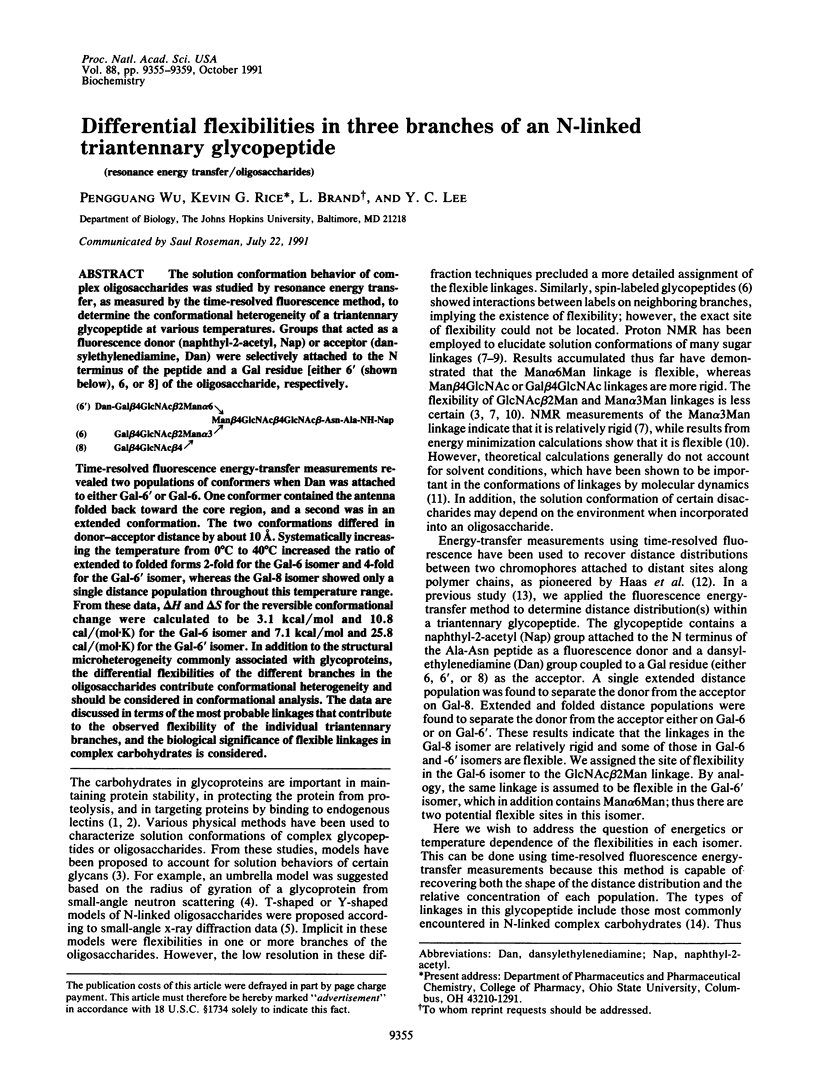
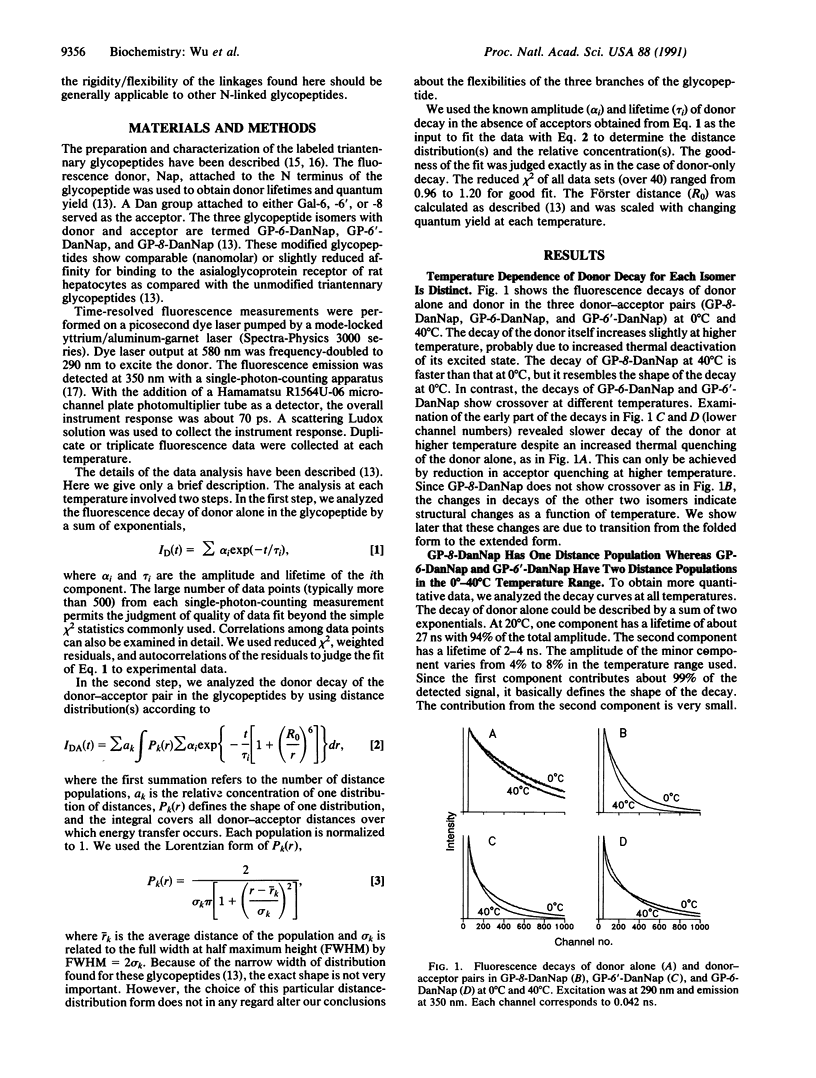
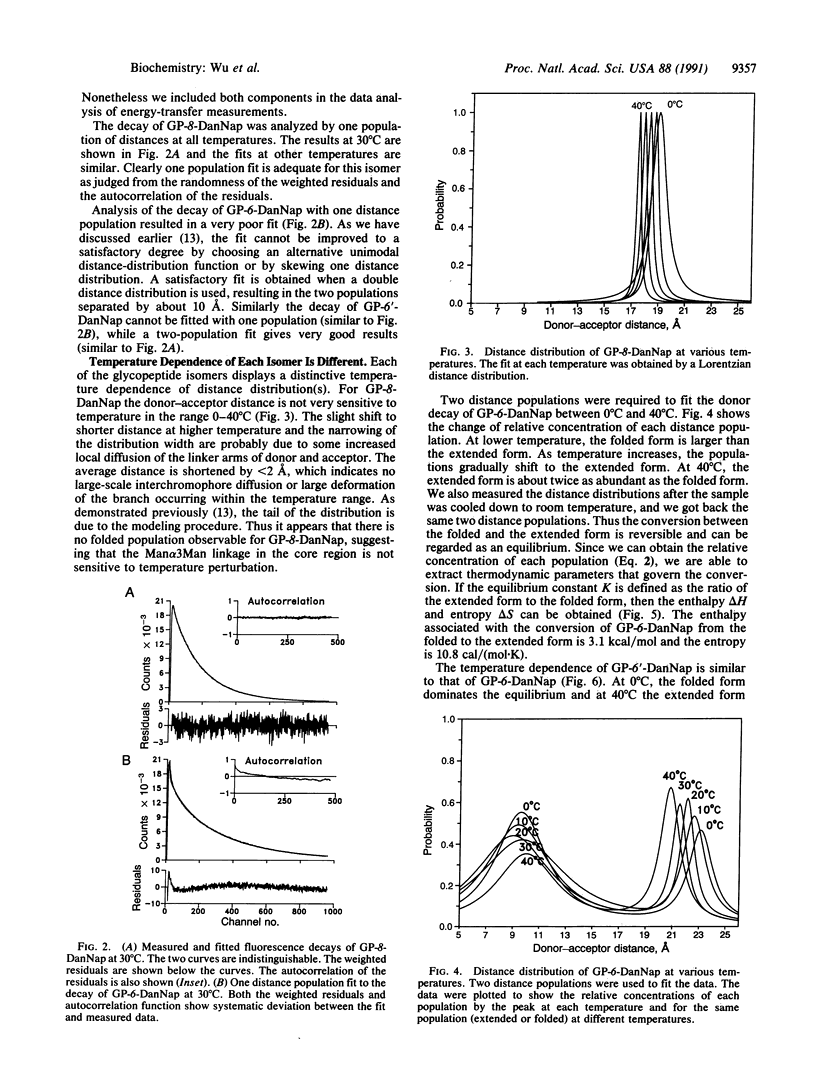
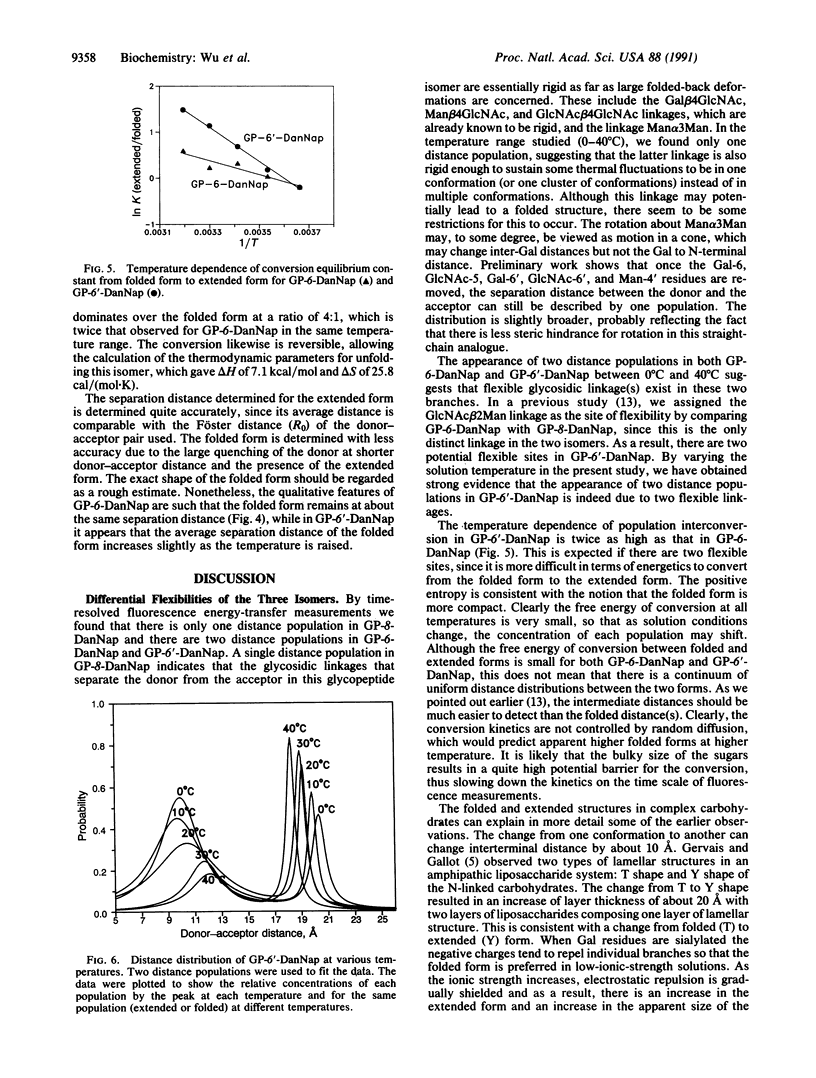
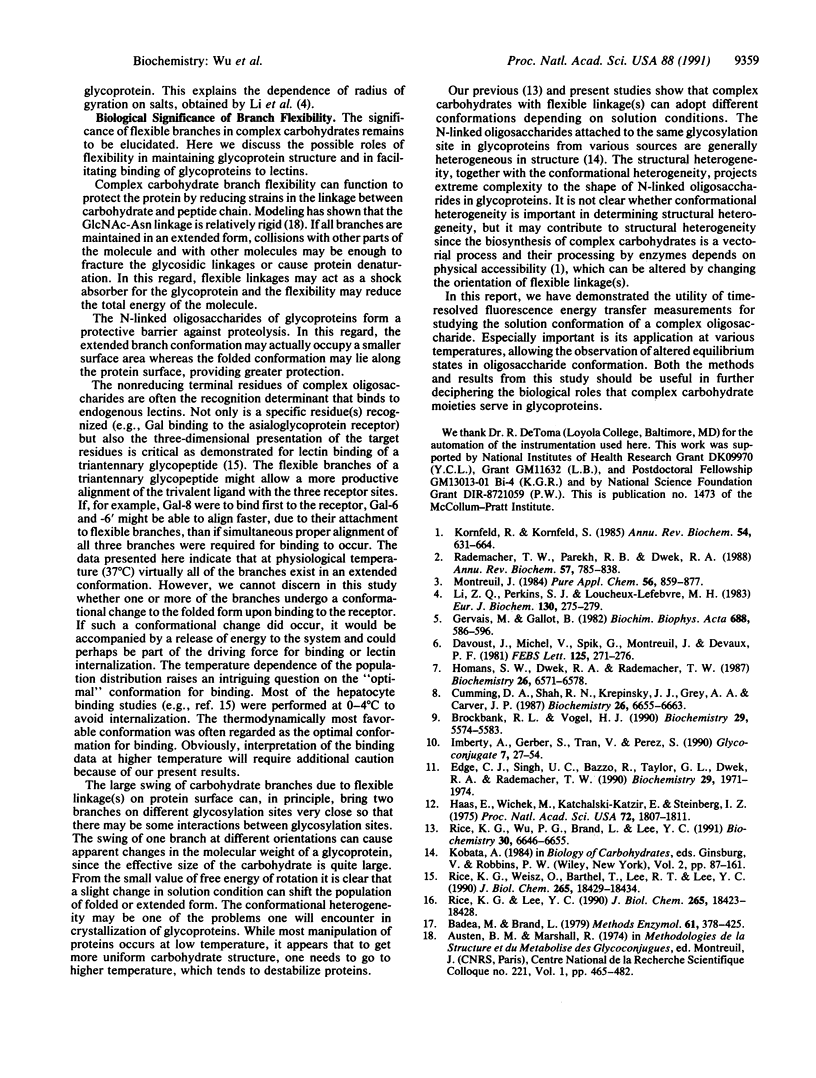
Selected References
These references are in PubMed. This may not be the complete list of references from this article.
- Badea M. G., Brand L. Time-resolved fluorescence measurements. Methods Enzymol. 1979;61:378–425. doi: 10.1016/0076-6879(79)61019-4. [DOI] [PubMed] [Google Scholar]
- Brockbank R. L., Vogel H. J. Structure of the oligosaccharide of hen phosvitin as determined by two-dimensional 1H NMR of the intact glycoprotein. Biochemistry. 1990 Jun 12;29(23):5574–5583. doi: 10.1021/bi00475a023. [DOI] [PubMed] [Google Scholar]
- Cumming D. A., Shah R. N., Krepinsky J. J., Grey A. A., Carver J. P. Solution conformation of the branch points of N-linked glycans: synthetic model compounds for tri'-antennary and tetraantennary glycans. Biochemistry. 1987 Oct 20;26(21):6655–6663. doi: 10.1021/bi00395a015. [DOI] [PubMed] [Google Scholar]
- Davoust J., Michel V., Spik G., Montreuil J., Devaux P. F. Flexibility of bi- and triantennary glycans of the N-acetyllactosaminic type. A spin label study. FEBS Lett. 1981 Mar 23;125(2):271–276. doi: 10.1016/0014-5793(81)80736-3. [DOI] [PubMed] [Google Scholar]
- Edge C. J., Singh U. C., Bazzo R., Taylor G. L., Dwek R. A., Rademacher T. W. 500-picosecond molecular dynamics in water of the Man alpha 1----2Man alpha glycosidic linkage present in Asn-linked oligomannose-type structures on glycoproteins. Biochemistry. 1990 Feb 27;29(8):1971–1974. doi: 10.1021/bi00460a001. [DOI] [PubMed] [Google Scholar]
- Haas E., Wilchek M., Katchalski-Katzir E., Steinberg I. Z. Distribution of end-to-end distances of oligopeptides in solution as estimated by energy transfer. Proc Natl Acad Sci U S A. 1975 May;72(5):1807–1811. doi: 10.1073/pnas.72.5.1807. [DOI] [PMC free article] [PubMed] [Google Scholar]
- Homans S. W., Dwek R. A., Rademacher T. W. Solution conformations of N-linked oligosaccharides. Biochemistry. 1987 Oct 20;26(21):6571–6578. doi: 10.1021/bi00395a001. [DOI] [PubMed] [Google Scholar]
- Kornfeld R., Kornfeld S. Assembly of asparagine-linked oligosaccharides. Annu Rev Biochem. 1985;54:631–664. doi: 10.1146/annurev.bi.54.070185.003215. [DOI] [PubMed] [Google Scholar]
- Li Z. Q., Perkins S. J., Loucheux-Lefebvre M. H. alpha 1 acid glycoprotein: a small-angle neutron scattering study of a human plasma glycoprotein. Eur J Biochem. 1983 Feb 1;130(2):275–279. doi: 10.1111/j.1432-1033.1983.tb07147.x. [DOI] [PubMed] [Google Scholar]
- Rademacher T. W., Parekh R. B., Dwek R. A. Glycobiology. Annu Rev Biochem. 1988;57:785–838. doi: 10.1146/annurev.bi.57.070188.004033. [DOI] [PubMed] [Google Scholar]
- Rice K. G., Lee Y. C. Modification of triantennary glycopeptide into probes for the asialoglycoprotein receptor of hepatocytes. J Biol Chem. 1990 Oct 25;265(30):18423–18428. [PubMed] [Google Scholar]
- Rice K. G., Weisz O. A., Barthel T., Lee R. T., Lee Y. C. Defined geometry of binding between triantennary glycopeptide and the asialoglycoprotein receptor of rat heptocytes. J Biol Chem. 1990 Oct 25;265(30):18429–18434. [PubMed] [Google Scholar]
- Rice K. G., Wu R. G., Brand L., Lee Y. C. Interterminal distance and flexibility of a triantennary glycopeptide as measured by resonance energy transfer. Biochemistry. 1991 Jul 9;30(27):6646–6655. doi: 10.1021/bi00241a003. [DOI] [PubMed] [Google Scholar]