Abstract
The Escherichia coli mannitol permease catalyzes the concomitant transport and phosphorylation of D-mannitol. This 68-kDa protein consists of a membrane-bound, N-terminal domain involved in mannitol binding and translocation and a C-terminal, cytoplasmic domain responsible for mannitol phosphorylation. Secondary-structure prediction methods suggest that the N-terminal half of the permease spans the membrane approximately seven times in alpha-helical segments, but these data cannot conclusively predict the structure. We have used gene fusions between mtlA (encoding the permease) and 'phoA (encoding alkaline phosphatase lacking its signal sequence) to further investigate the topology of the mannitol permease. Initially, fusions were constructed by using a lambda TnphoA vector and in vitro cloning of 'phoA into naturally occurring restriction sites in mtlA. However, the former method gave severe problems with insertion "hot-spots" in our vector systems, and the latter method was limited by the number of useful restriction sites. Therefore, we developed a nested-deletion method for creating mtlA-phoA fusions. 'phoA was first cloned downstream from the part of mtlA encoding the membrane-bound half of the permease. This construct was then treated with the appropriate restriction enzymes and with exonuclease III to create random fusions. An analysis of greater than 40 different fusion clones constructed by these methods provides strong evidence for six membrane-spanning regions in the mannitol permease with three relatively short periplasmic loops and two large cytoplasmic loops in the membrane-bound half of the protein.
Full text
PDF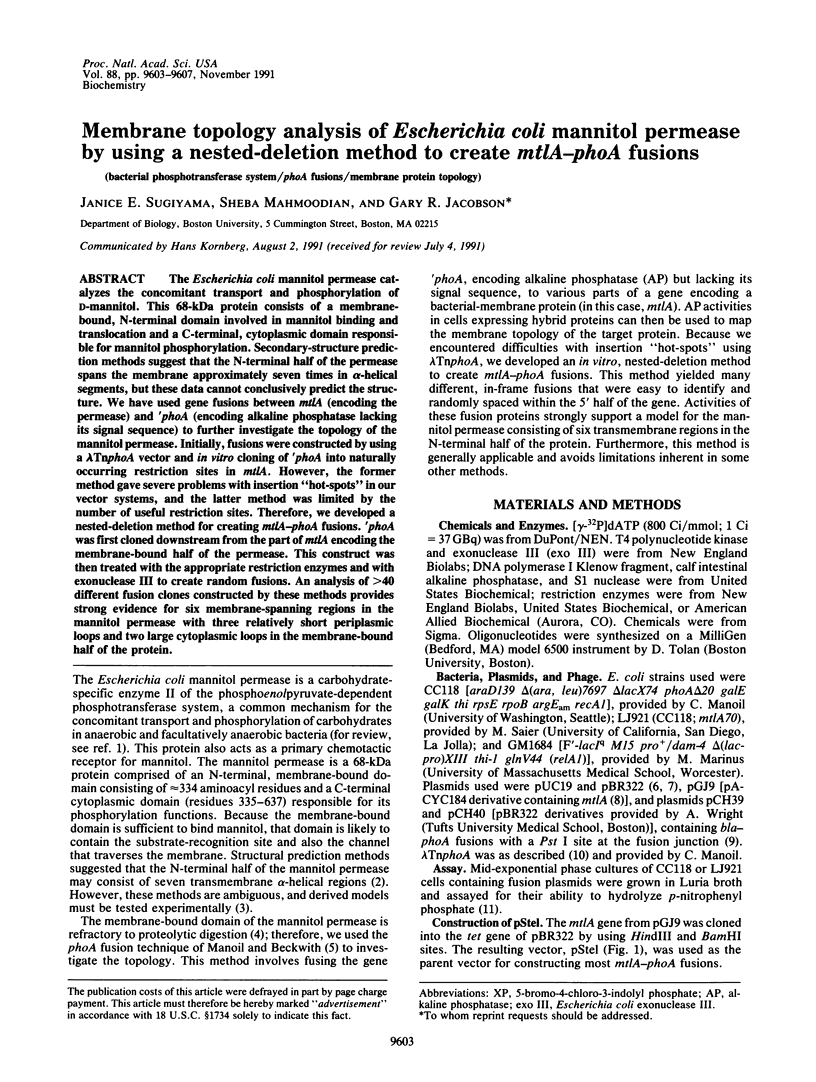
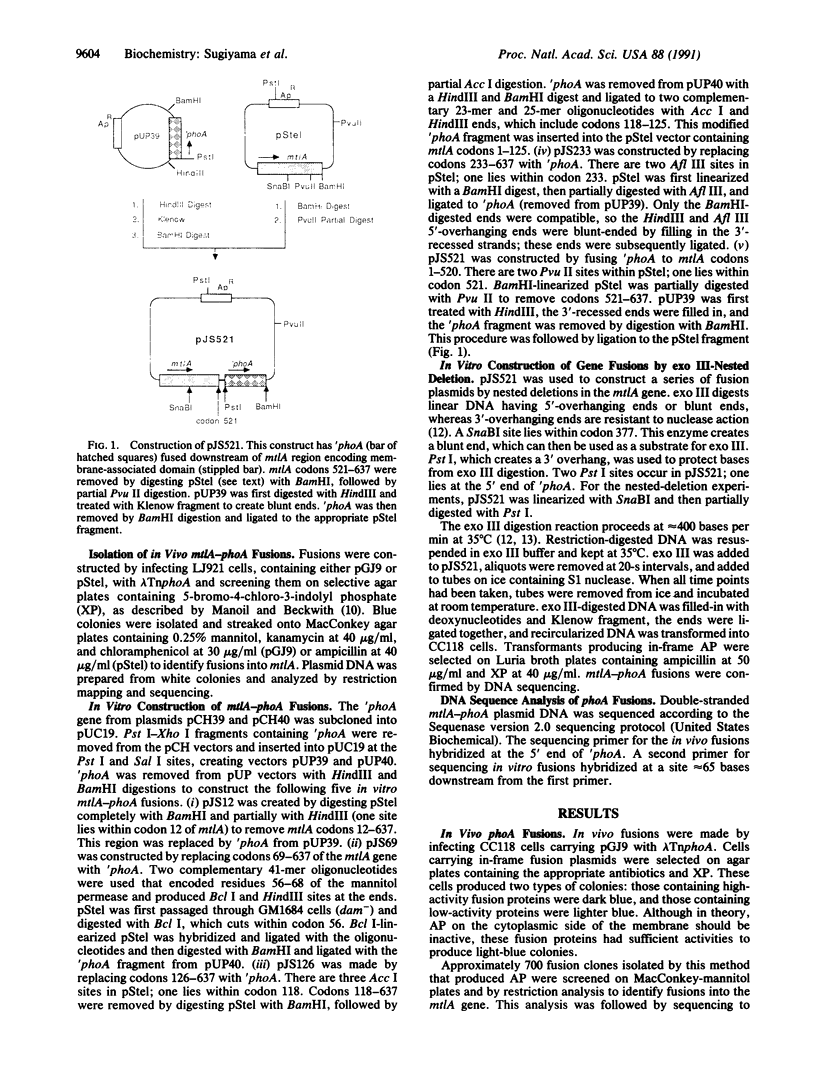
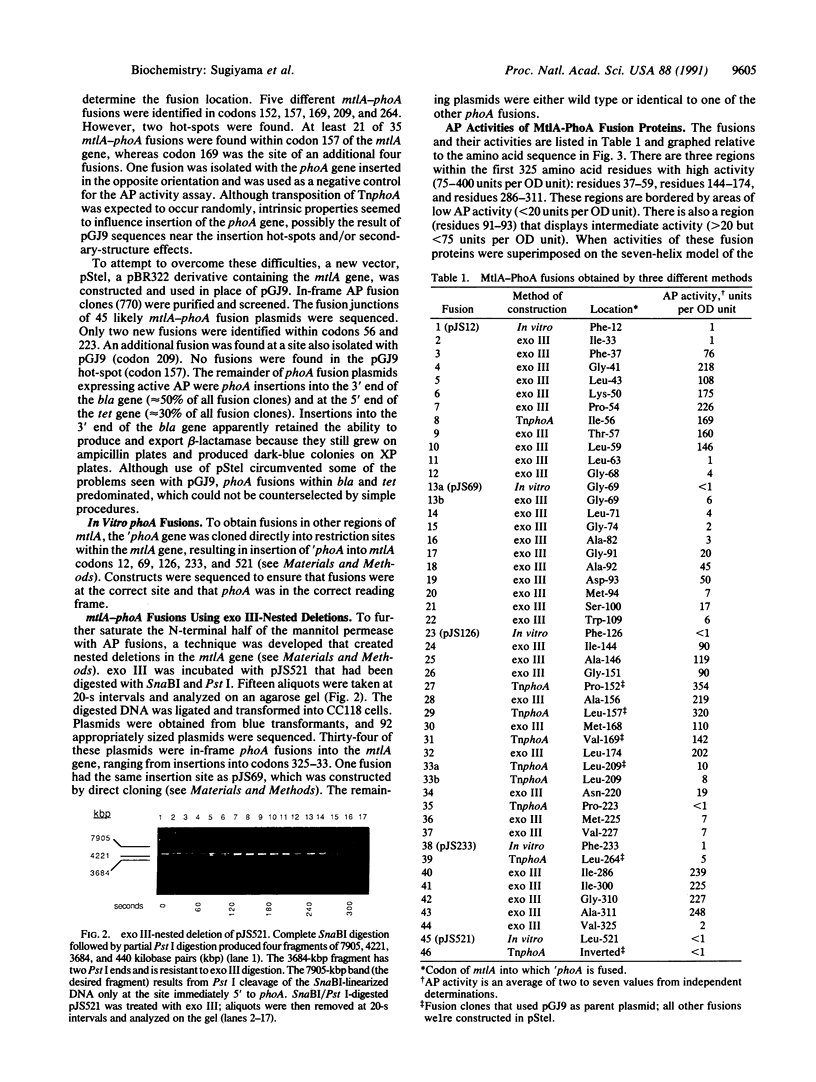
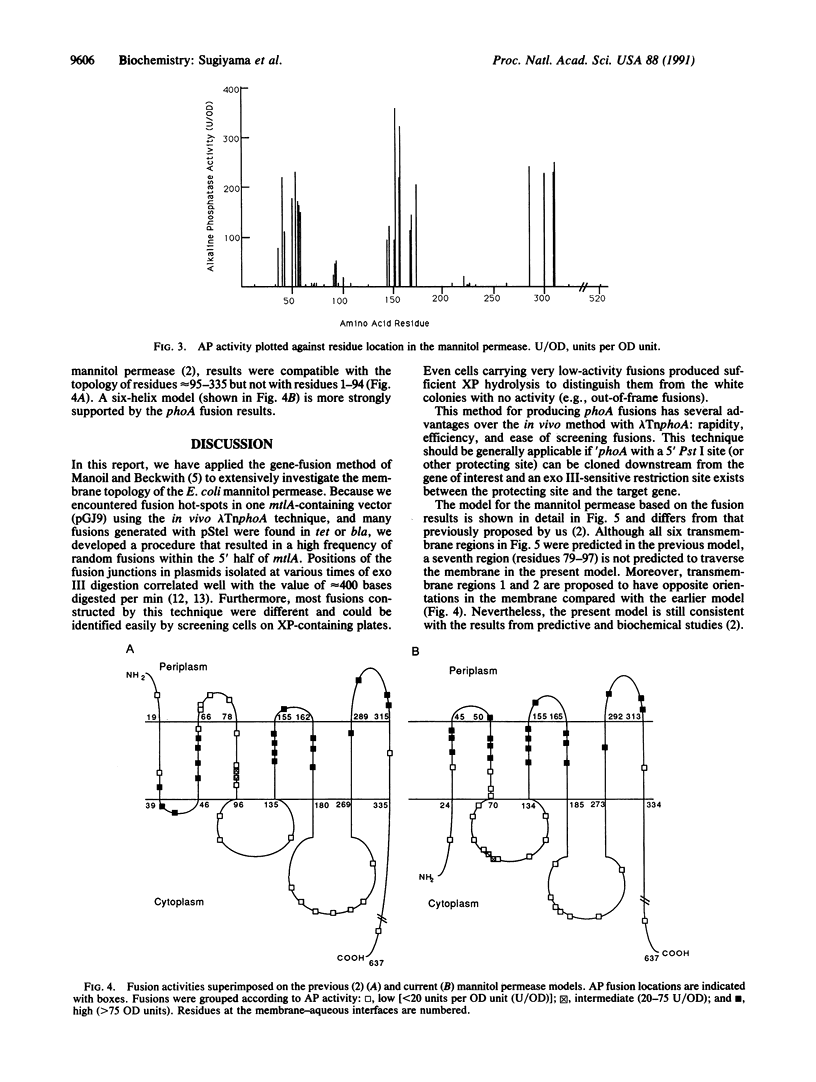
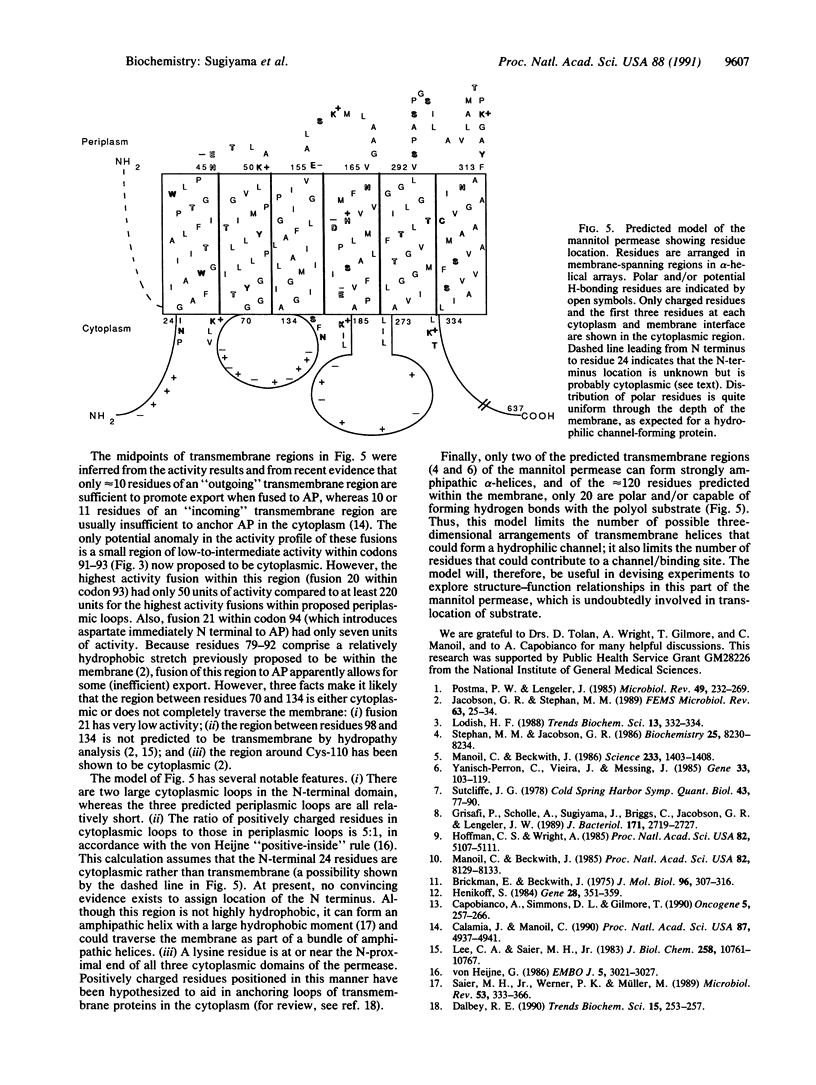
Images in this article
Selected References
These references are in PubMed. This may not be the complete list of references from this article.
- Brickman E., Beckwith J. Analysis of the regulation of Escherichia coli alkaline phosphatase synthesis using deletions and phi80 transducing phages. J Mol Biol. 1975 Aug 5;96(2):307–316. doi: 10.1016/0022-2836(75)90350-2. [DOI] [PubMed] [Google Scholar]
- Calamia J., Manoil C. lac permease of Escherichia coli: topology and sequence elements promoting membrane insertion. Proc Natl Acad Sci U S A. 1990 Jul;87(13):4937–4941. doi: 10.1073/pnas.87.13.4937. [DOI] [PMC free article] [PubMed] [Google Scholar]
- Capobianco A. J., Simmons D. L., Gilmore T. D. Cloning and expression of a chicken c-rel cDNA: unlike p59v-rel, p68c-rel is a cytoplasmic protein in chicken embryo fibroblasts. Oncogene. 1990 Mar;5(3):257–265. [PubMed] [Google Scholar]
- Dalbey R. E. Positively charged residues are important determinants of membrane protein topology. Trends Biochem Sci. 1990 Jul;15(7):253–257. doi: 10.1016/0968-0004(90)90047-f. [DOI] [PubMed] [Google Scholar]
- Grisafi P. L., Scholle A., Sugiyama J., Briggs C., Jacobson G. R., Lengeler J. W. Deletion mutants of the Escherichia coli K-12 mannitol permease: dissection of transport-phosphorylation, phospho-exchange, and mannitol-binding activities. J Bacteriol. 1989 May;171(5):2719–2727. doi: 10.1128/jb.171.5.2719-2727.1989. [DOI] [PMC free article] [PubMed] [Google Scholar]
- Heijne G. The distribution of positively charged residues in bacterial inner membrane proteins correlates with the trans-membrane topology. EMBO J. 1986 Nov;5(11):3021–3027. doi: 10.1002/j.1460-2075.1986.tb04601.x. [DOI] [PMC free article] [PubMed] [Google Scholar]
- Henikoff S. Unidirectional digestion with exonuclease III creates targeted breakpoints for DNA sequencing. Gene. 1984 Jun;28(3):351–359. doi: 10.1016/0378-1119(84)90153-7. [DOI] [PubMed] [Google Scholar]
- Hoffman C. S., Wright A. Fusions of secreted proteins to alkaline phosphatase: an approach for studying protein secretion. Proc Natl Acad Sci U S A. 1985 Aug;82(15):5107–5111. doi: 10.1073/pnas.82.15.5107. [DOI] [PMC free article] [PubMed] [Google Scholar]
- Jacobson G. R., Stephan M. M. Structural and functional domains of the mannitol-specific enzyme II of the E. coli phosphoenolpyruvate-dependent phosphotransferase system. FEMS Microbiol Rev. 1989 Jun;5(1-2):25–34. doi: 10.1016/0168-6445(89)90005-3. [DOI] [PubMed] [Google Scholar]
- Lee C. A., Saier M. H., Jr Mannitol-specific enzyme II of the bacterial phosphotransferase system. III. The nucleotide sequence of the permease gene. J Biol Chem. 1983 Sep 10;258(17):10761–10767. [PubMed] [Google Scholar]
- Lodish H. F. Multi-spanning membrane proteins: how accurate are the models? Trends Biochem Sci. 1988 Sep;13(9):332–334. doi: 10.1016/0968-0004(88)90101-6. [DOI] [PubMed] [Google Scholar]
- Manoil C., Beckwith J. A genetic approach to analyzing membrane protein topology. Science. 1986 Sep 26;233(4771):1403–1408. doi: 10.1126/science.3529391. [DOI] [PubMed] [Google Scholar]
- Manoil C., Beckwith J. TnphoA: a transposon probe for protein export signals. Proc Natl Acad Sci U S A. 1985 Dec;82(23):8129–8133. doi: 10.1073/pnas.82.23.8129. [DOI] [PMC free article] [PubMed] [Google Scholar]
- Postma P. W., Lengeler J. W. Phosphoenolpyruvate:carbohydrate phosphotransferase system of bacteria. Microbiol Rev. 1985 Sep;49(3):232–269. doi: 10.1128/mr.49.3.232-269.1985. [DOI] [PMC free article] [PubMed] [Google Scholar]
- Saier M. H., Jr, Werner P. K., Müller M. Insertion of proteins into bacterial membranes: mechanism, characteristics, and comparisons with the eucaryotic process. Microbiol Rev. 1989 Sep;53(3):333–366. doi: 10.1128/mr.53.3.333-366.1989. [DOI] [PMC free article] [PubMed] [Google Scholar]
- Stephan M. M., Jacobson G. R. Membrane disposition of the Escherichia coli mannitol permease: identification of membrane-bound and cytoplasmic domains. Biochemistry. 1986 Dec 16;25(25):8230–8234. doi: 10.1021/bi00373a016. [DOI] [PubMed] [Google Scholar]
- Sutcliffe J. G. Complete nucleotide sequence of the Escherichia coli plasmid pBR322. Cold Spring Harb Symp Quant Biol. 1979;43(Pt 1):77–90. doi: 10.1101/sqb.1979.043.01.013. [DOI] [PubMed] [Google Scholar]
- Yanisch-Perron C., Vieira J., Messing J. Improved M13 phage cloning vectors and host strains: nucleotide sequences of the M13mp18 and pUC19 vectors. Gene. 1985;33(1):103–119. doi: 10.1016/0378-1119(85)90120-9. [DOI] [PubMed] [Google Scholar]