Abstract
At physiological concentrations of HCO3- and CO2, Mn(II) catalyzes disproportionation of H2O2. This catalase-like activity is directly proportional to the concentrations of Mn(II) and H2O2, and it increases exponentially with increases in pH. The effect of increasing pH is almost completely attributable to the concomitant increase in HCO3- concentration. The rate is proportional to the third power of the HCO3- concentration, suggesting that 3 equivalents of HCO3- combine with 1 equivalent of Mn(II) to form the catalytic complex. It is presumed that the redox potential of the Mn(II) in equilibrium with Mn(III) couple in such a complex permits H2O2 to carry out facile reactions with Mn(II) comparable to those that occur with Fe(III) and Cu(II) chelate complexes, in which OH. and O2-. are established intermediates. The Mn-catalyzed disproportionation of H2O2 does not occur at physiological pH in the absence of HCO3-. Hepes, inorganic phosphate, and inorganic pyrophosphate inhibit the reaction catalyzed by the Mn/HCO3- system. These results are similar to those of Sychev et al. [Sychev, A.Y., Pfannmeller, U. & Isak, V.G. (1983) Russ. J. Phys. Chem. 57, 1690-1693]. The catalase-like activity of Mn(II)-bicarbonate complexes reported here, together with the superoxide dismutase activity of Mn complexes demonstrated by Archibald and Fridovich [Archibald, F.S. & Fridovich, I. (1982) Arch. Biochem. Biophys. 214, 452-463], strengthen the proposition that Mn may play an important role in the protection of cells against oxygen radical-mediated damage.
Full text
PDF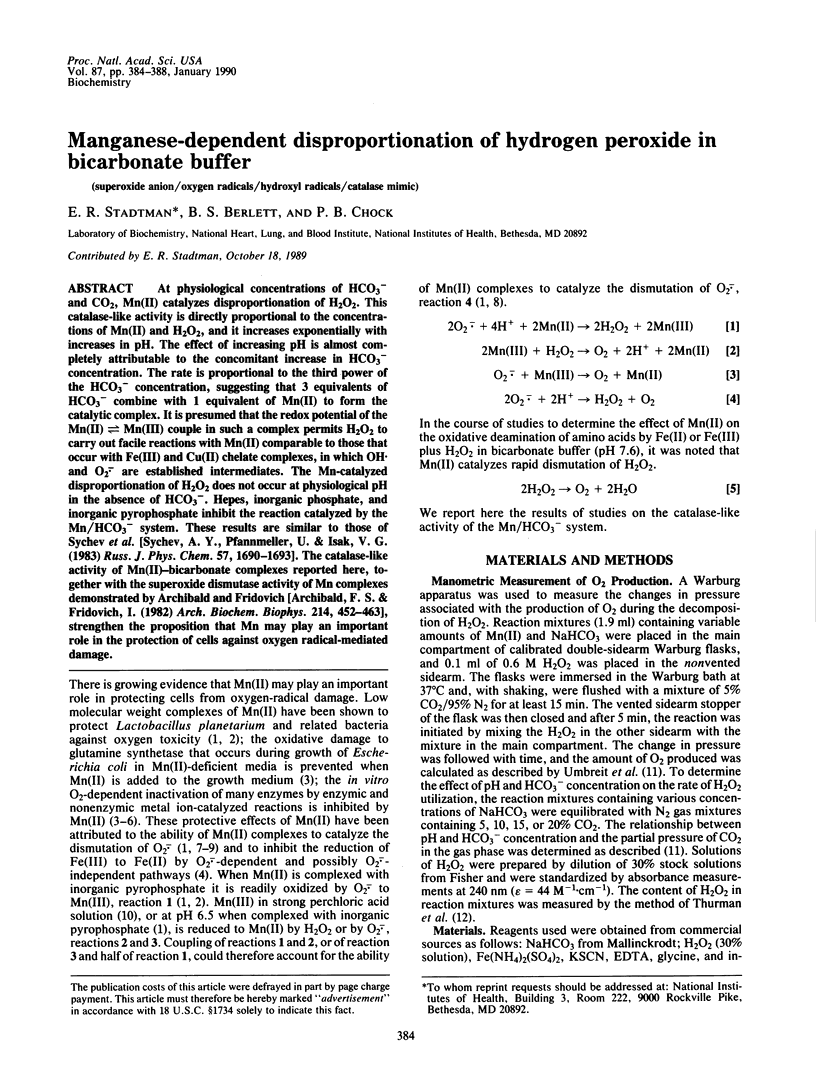
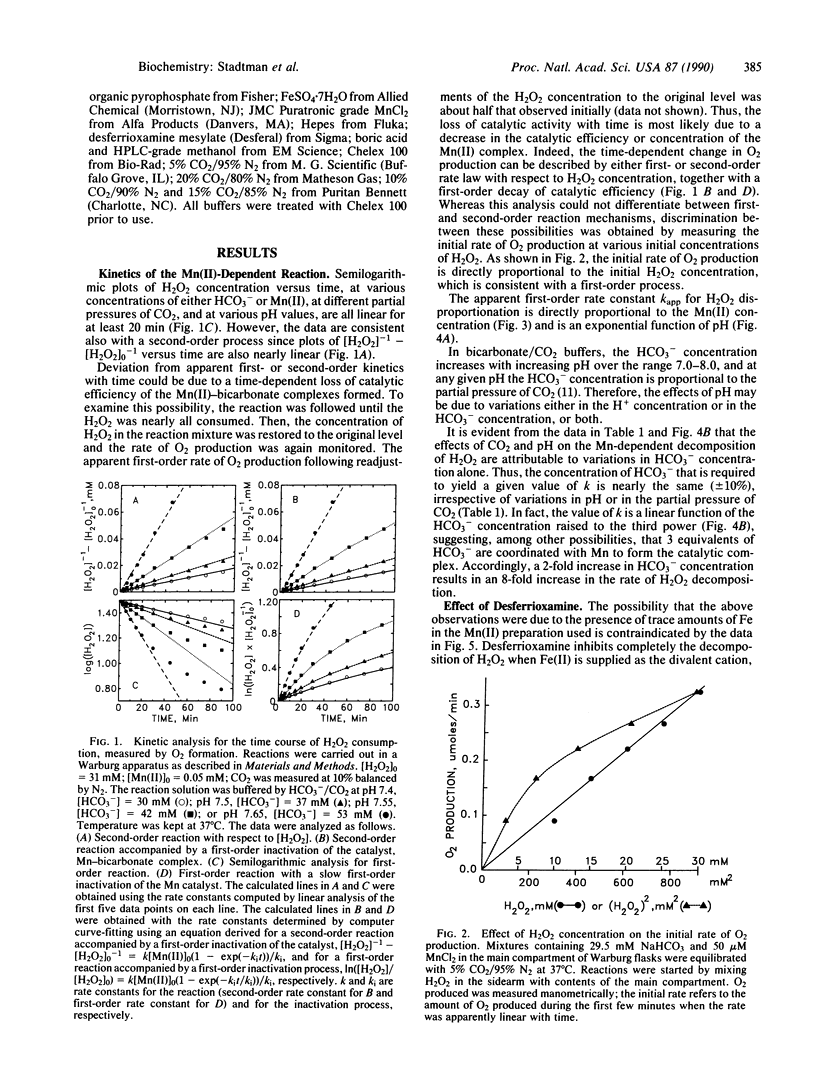
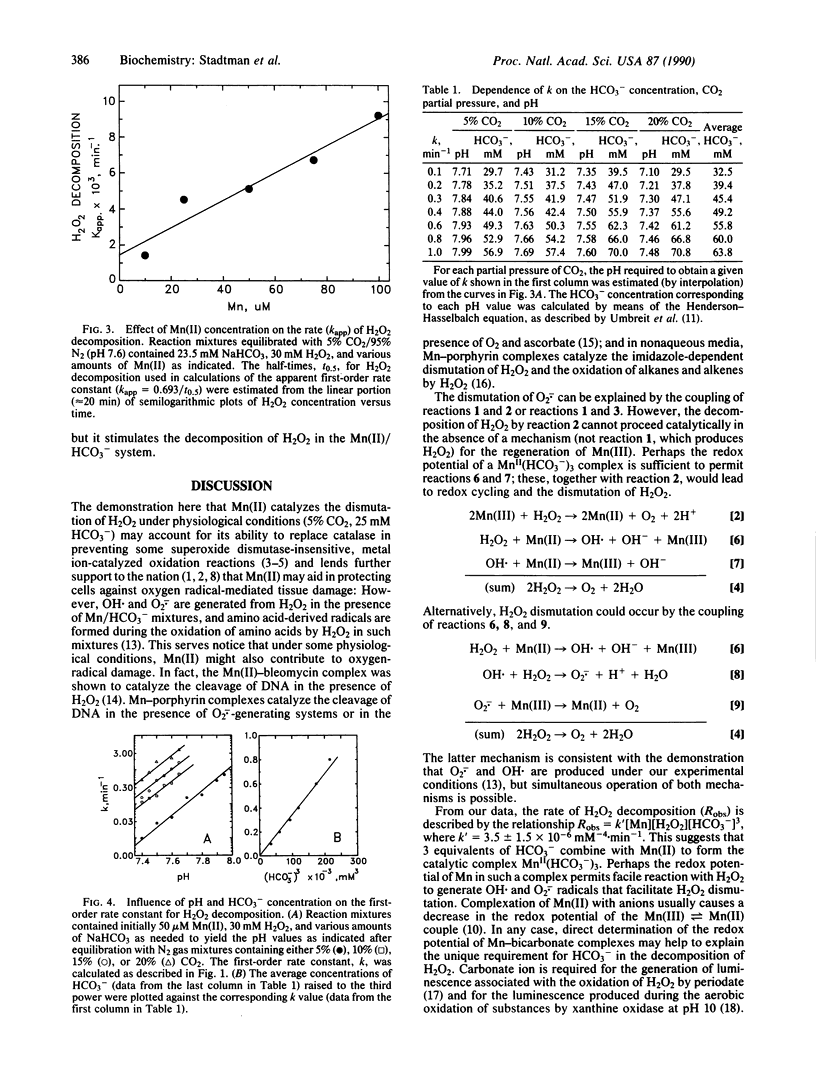
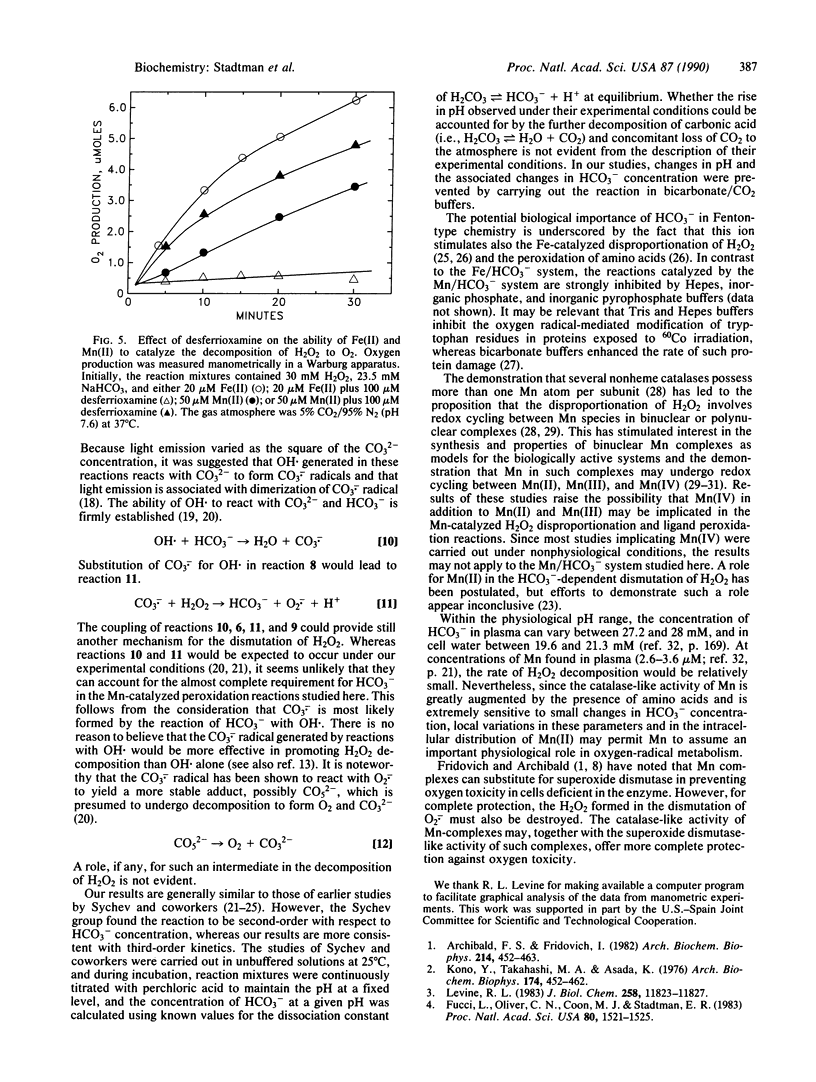
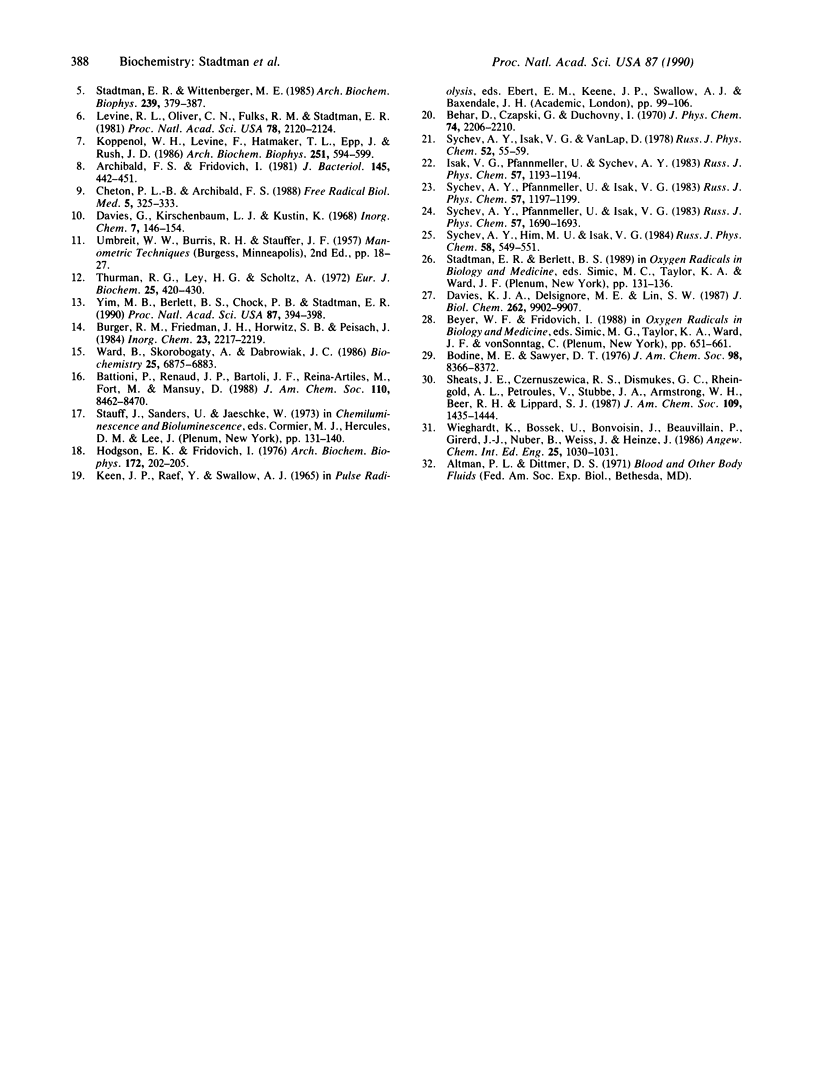
Selected References
These references are in PubMed. This may not be the complete list of references from this article.
- Archibald F. S., Fridovich I. Manganese and defenses against oxygen toxicity in Lactobacillus plantarum. J Bacteriol. 1981 Jan;145(1):442–451. doi: 10.1128/jb.145.1.442-451.1981. [DOI] [PMC free article] [PubMed] [Google Scholar]
- Archibald F. S., Fridovich I. The scavenging of superoxide radical by manganous complexes: in vitro. Arch Biochem Biophys. 1982 Apr 1;214(2):452–463. doi: 10.1016/0003-9861(82)90049-2. [DOI] [PubMed] [Google Scholar]
- Cheton P. L., Archibald F. S. Manganese complexes and the generation and scavenging of hydroxyl free radicals. Free Radic Biol Med. 1988;5(5-6):325–333. doi: 10.1016/0891-5849(88)90104-9. [DOI] [PubMed] [Google Scholar]
- Davies K. J., Delsignore M. E., Lin S. W. Protein damage and degradation by oxygen radicals. II. Modification of amino acids. J Biol Chem. 1987 Jul 15;262(20):9902–9907. [PubMed] [Google Scholar]
- Fucci L., Oliver C. N., Coon M. J., Stadtman E. R. Inactivation of key metabolic enzymes by mixed-function oxidation reactions: possible implication in protein turnover and ageing. Proc Natl Acad Sci U S A. 1983 Mar;80(6):1521–1525. doi: 10.1073/pnas.80.6.1521. [DOI] [PMC free article] [PubMed] [Google Scholar]
- Hodgson E. K., Fridovich I. The mechanism of the activity-dependent luminescence of xanthine oxidase. Arch Biochem Biophys. 1976 Jan;172(1):202–205. doi: 10.1016/0003-9861(76)90067-9. [DOI] [PubMed] [Google Scholar]
- Kono Y., Takahashi M. A., Asada K. Oxidation of manganous pyrophosphate by superoxide radicals and illuminated spinach chloroplasts. Arch Biochem Biophys. 1976 Jun;174(2):454–462. doi: 10.1016/0003-9861(76)90373-8. [DOI] [PubMed] [Google Scholar]
- Koppenol W. H., Levine F., Hatmaker T. L., Epp J., Rush J. D. Catalysis of superoxide dismutation by manganese aminopolycarboxylate complexes. Arch Biochem Biophys. 1986 Dec;251(2):594–599. doi: 10.1016/0003-9861(86)90368-1. [DOI] [PubMed] [Google Scholar]
- Levine R. L., Oliver C. N., Fulks R. M., Stadtman E. R. Turnover of bacterial glutamine synthetase: oxidative inactivation precedes proteolysis. Proc Natl Acad Sci U S A. 1981 Apr;78(4):2120–2124. doi: 10.1073/pnas.78.4.2120. [DOI] [PMC free article] [PubMed] [Google Scholar]
- Levine R. L. Oxidative modification of glutamine synthetase. I. Inactivation is due to loss of one histidine residue. J Biol Chem. 1983 Oct 10;258(19):11823–11827. [PubMed] [Google Scholar]
- Stadtman E. R., Wittenberger M. E. Inactivation of Escherichia coli glutamine synthetase by xanthine oxidase, nicotinate hydroxylase, horseradish peroxidase, or glucose oxidase: effects of ferredoxin, putidaredoxin, and menadione. Arch Biochem Biophys. 1985 Jun;239(2):379–387. doi: 10.1016/0003-9861(85)90703-9. [DOI] [PubMed] [Google Scholar]
- Thurman R. G., Ley H. G., Scholz R. Hepatic microsomal ethanol oxidation. Hydrogen peroxide formation and the role of catalase. Eur J Biochem. 1972 Feb;25(3):420–430. doi: 10.1111/j.1432-1033.1972.tb01711.x. [DOI] [PubMed] [Google Scholar]
- Ward B., Skorobogaty A., Dabrowiak J. C. DNA cleavage specificity of a group of cationic metalloporphyrins. Biochemistry. 1986 Nov 4;25(22):6875–6883. doi: 10.1021/bi00370a021. [DOI] [PubMed] [Google Scholar]
- Yim M. B., Berlett B. S., Chock P. B., Stadtman E. R. Manganese(II)-bicarbonate-mediated catalytic activity for hydrogen peroxide dismutation and amino acid oxidation: detection of free radical intermediates. Proc Natl Acad Sci U S A. 1990 Jan;87(1):394–398. doi: 10.1073/pnas.87.1.394. [DOI] [PMC free article] [PubMed] [Google Scholar]