Abstract
In bicarbonate/CO2 buffer, Mn(II) and Fe(II) catalyze the oxidation of amino acids by H2O2 and the dismutation of H2O2. As the Mn(II)/Fe(II) ratio is increased, the yield of carbonyl compounds per mole of leucine oxidized is essentially constant, but the ratio of alpha-ketoisocaproate to isovaleraldehyde formed increases, and the fraction of H2O2 converted to O2 increases. In the absence of Fe(II), the rate of Mn(II)-catalyzed leucine oxidation is directly proportional to the H2O2, Mn(II), and amino acid concentrations and is proportional to the square of the HCO3- concentration. The rate of Mn(II)-catalyzed O2 production in the presence of 50 mM alanine or leucine is about 4-fold the rate observed in the absence of amino acids and accounts for about half of the H2O2 consumed; the other half of the H2O2 is consumed in the oxidation of the amino acids. In contrast, O2 production is increased nearly 18-fold by the presence of alpha-methylalanine and accounts for about 90% of the H2O2 consumed. The data are consistent with the view that H2O2 decomposition is an inner sphere (cage-like) process catalyzed by a Mn coordination complex of the composition Mn(II), amino acid, (HCO3-)2. Oxidation of the amino acid in this complex most likely proceeds by a free radical mechanism involving hydrogen abstraction from the alpha-carbon as a critical step. The results demonstrate that at physiological concentrations of HCO3- and CO2, Mn(II) is able to facilitate Fenton-type reactions.
Full text
PDF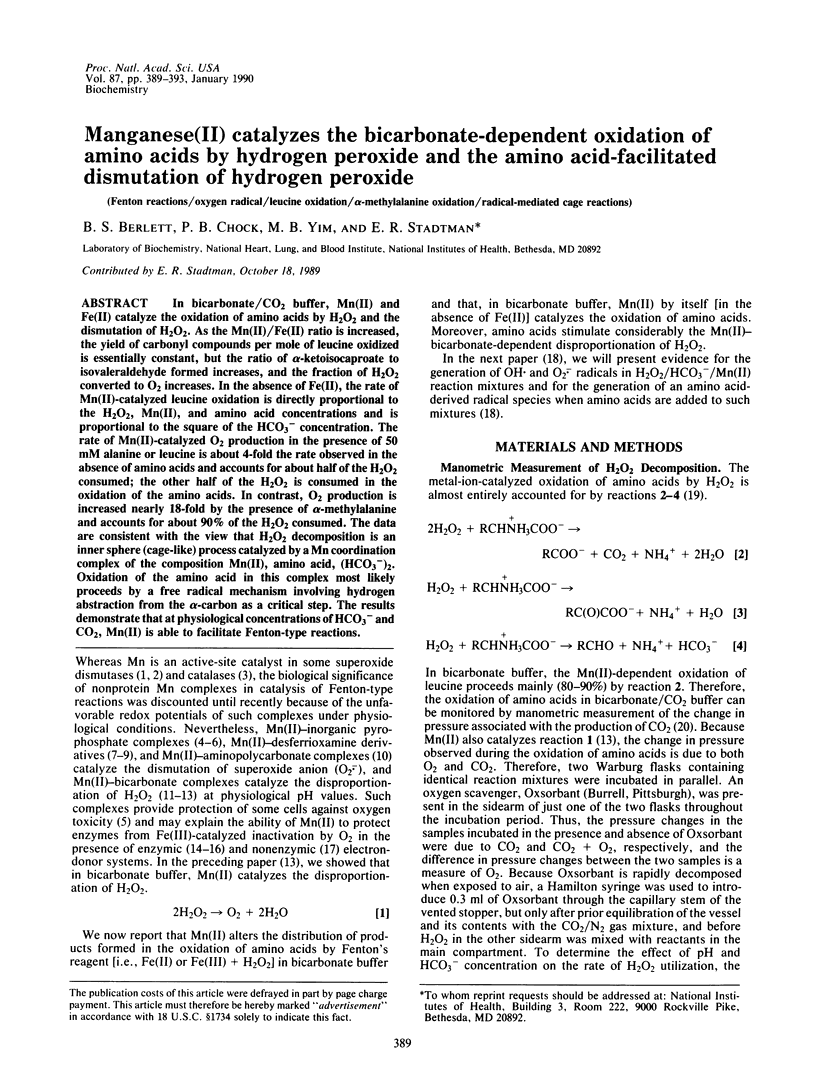
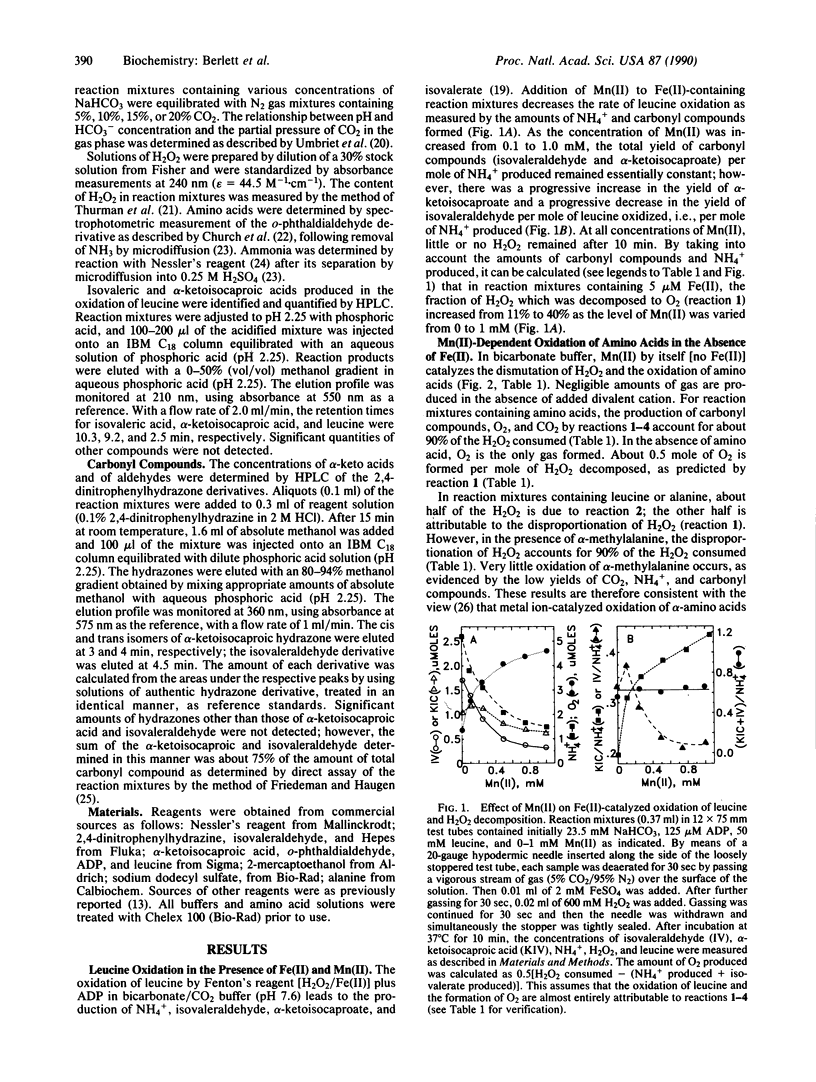
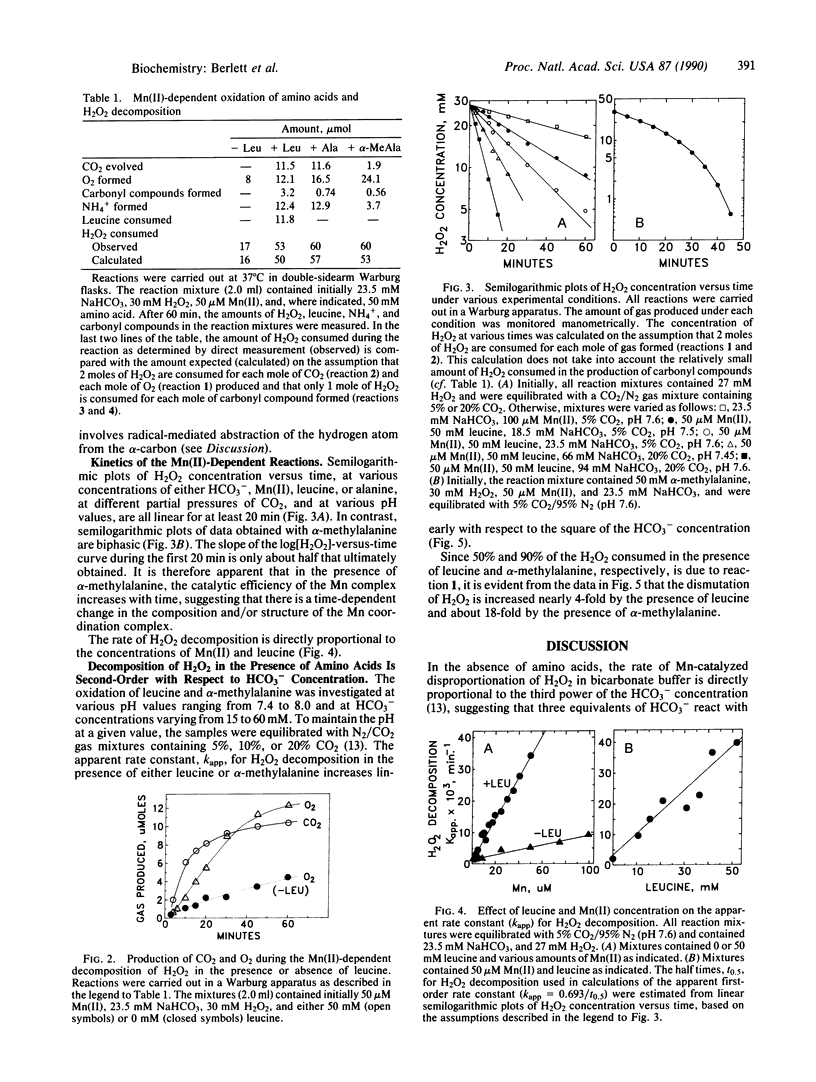
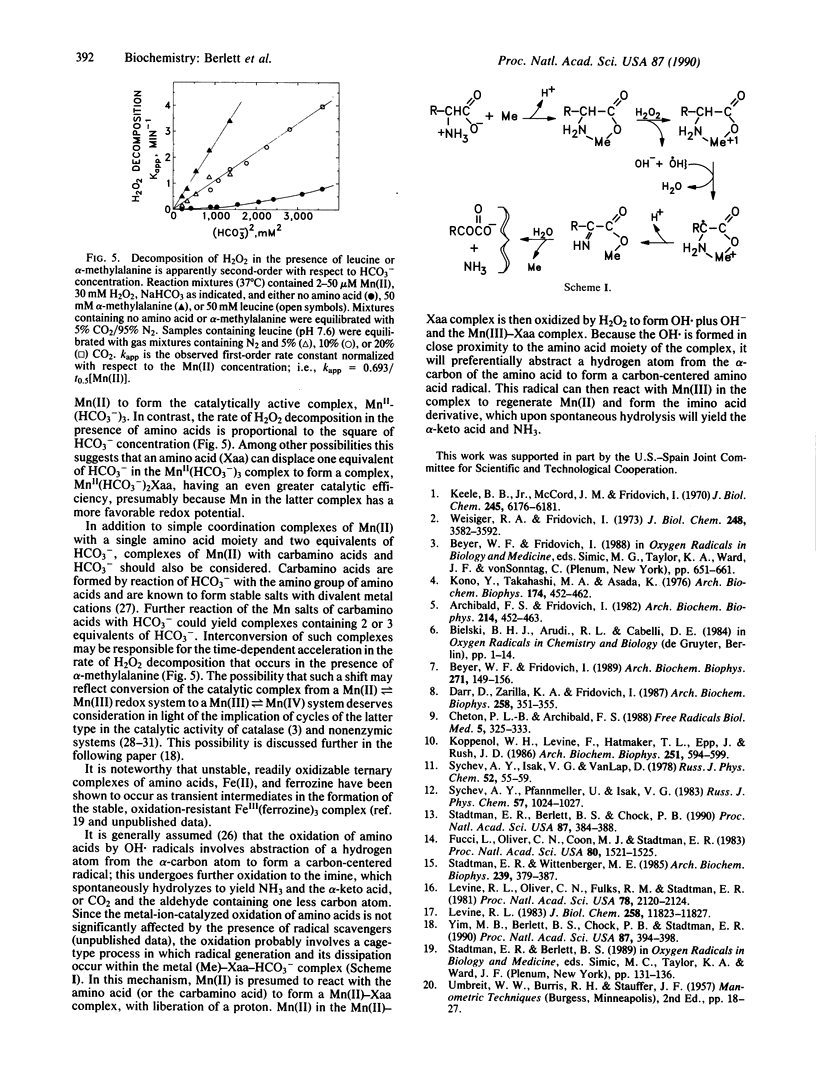
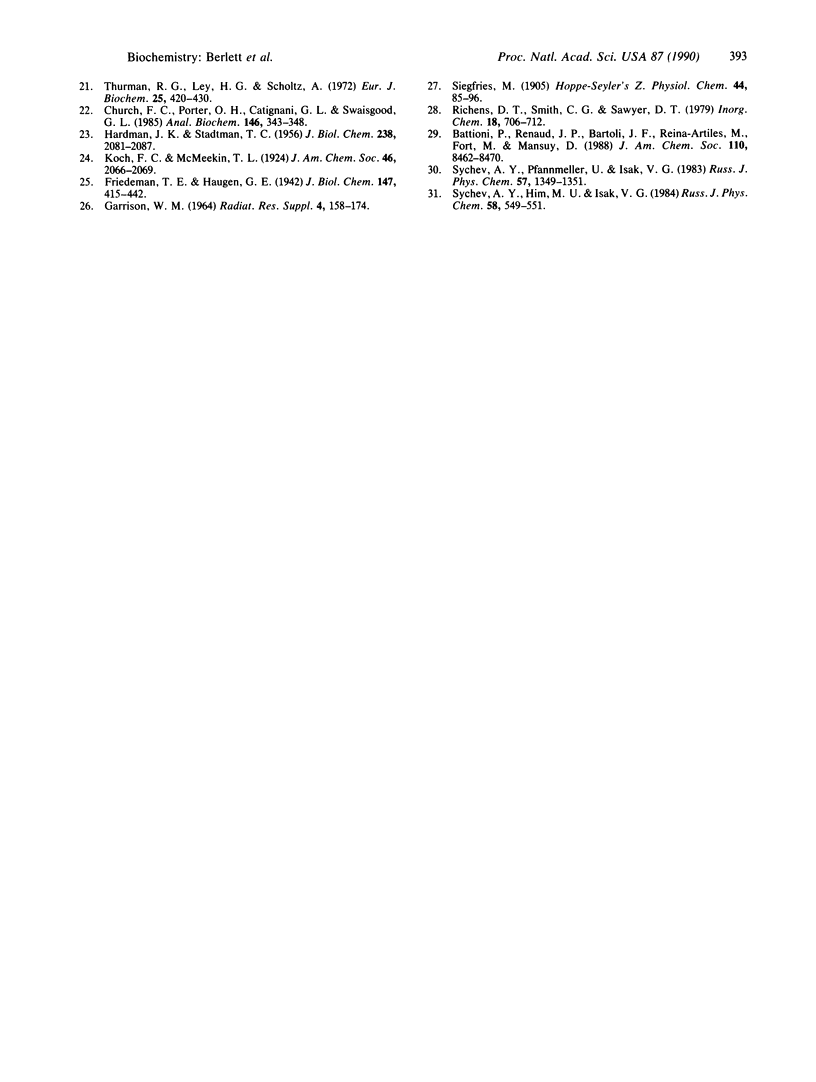
Selected References
These references are in PubMed. This may not be the complete list of references from this article.
- Archibald F. S., Fridovich I. The scavenging of superoxide radical by manganous complexes: in vitro. Arch Biochem Biophys. 1982 Apr 1;214(2):452–463. doi: 10.1016/0003-9861(82)90049-2. [DOI] [PubMed] [Google Scholar]
- Beyer W. F., Jr, Fridovich I. Characterization of a superoxide dismutase mimic prepared from desferrioxamine and MnO2. Arch Biochem Biophys. 1989 May 15;271(1):149–156. doi: 10.1016/0003-9861(89)90265-8. [DOI] [PubMed] [Google Scholar]
- Cheton P. L., Archibald F. S. Manganese complexes and the generation and scavenging of hydroxyl free radicals. Free Radic Biol Med. 1988;5(5-6):325–333. doi: 10.1016/0891-5849(88)90104-9. [DOI] [PubMed] [Google Scholar]
- Church F. C., Porter D. H., Catignani G. L., Swaisgood H. E. An o-phthalaldehyde spectrophotometric assay for proteinases. Anal Biochem. 1985 May 1;146(2):343–348. doi: 10.1016/0003-2697(85)90549-4. [DOI] [PubMed] [Google Scholar]
- Darr D., Zarilla K. A., Fridovich I. A mimic of superoxide dismutase activity based upon desferrioxamine B and manganese(IV). Arch Biochem Biophys. 1987 Nov 1;258(2):351–355. doi: 10.1016/0003-9861(87)90354-7. [DOI] [PubMed] [Google Scholar]
- Fucci L., Oliver C. N., Coon M. J., Stadtman E. R. Inactivation of key metabolic enzymes by mixed-function oxidation reactions: possible implication in protein turnover and ageing. Proc Natl Acad Sci U S A. 1983 Mar;80(6):1521–1525. doi: 10.1073/pnas.80.6.1521. [DOI] [PMC free article] [PubMed] [Google Scholar]
- HARDMAN J. K., STADTMAN T. C. Metabolism of amega-amino acids. III. Mechanism of conversion of gamma-aminobutyrate to gamma-hydroxybutryate by Clostridium aminobutyricum. J Biol Chem. 1963 Jun;238:2081–2087. [PubMed] [Google Scholar]
- Keele B. B., Jr, McCord J. M., Fridovich I. Superoxide dismutase from escherichia coli B. A new manganese-containing enzyme. J Biol Chem. 1970 Nov 25;245(22):6176–6181. [PubMed] [Google Scholar]
- Kono Y., Takahashi M. A., Asada K. Oxidation of manganous pyrophosphate by superoxide radicals and illuminated spinach chloroplasts. Arch Biochem Biophys. 1976 Jun;174(2):454–462. doi: 10.1016/0003-9861(76)90373-8. [DOI] [PubMed] [Google Scholar]
- Koppenol W. H., Levine F., Hatmaker T. L., Epp J., Rush J. D. Catalysis of superoxide dismutation by manganese aminopolycarboxylate complexes. Arch Biochem Biophys. 1986 Dec;251(2):594–599. doi: 10.1016/0003-9861(86)90368-1. [DOI] [PubMed] [Google Scholar]
- Levine R. L., Oliver C. N., Fulks R. M., Stadtman E. R. Turnover of bacterial glutamine synthetase: oxidative inactivation precedes proteolysis. Proc Natl Acad Sci U S A. 1981 Apr;78(4):2120–2124. doi: 10.1073/pnas.78.4.2120. [DOI] [PMC free article] [PubMed] [Google Scholar]
- Levine R. L. Oxidative modification of glutamine synthetase. I. Inactivation is due to loss of one histidine residue. J Biol Chem. 1983 Oct 10;258(19):11823–11827. [PubMed] [Google Scholar]
- Stadtman E. R., Berlett B. S., Chock P. B. Manganese-dependent disproportionation of hydrogen peroxide in bicarbonate buffer. Proc Natl Acad Sci U S A. 1990 Jan;87(1):384–388. doi: 10.1073/pnas.87.1.384. [DOI] [PMC free article] [PubMed] [Google Scholar]
- Stadtman E. R., Wittenberger M. E. Inactivation of Escherichia coli glutamine synthetase by xanthine oxidase, nicotinate hydroxylase, horseradish peroxidase, or glucose oxidase: effects of ferredoxin, putidaredoxin, and menadione. Arch Biochem Biophys. 1985 Jun;239(2):379–387. doi: 10.1016/0003-9861(85)90703-9. [DOI] [PubMed] [Google Scholar]
- Thurman R. G., Ley H. G., Scholz R. Hepatic microsomal ethanol oxidation. Hydrogen peroxide formation and the role of catalase. Eur J Biochem. 1972 Feb;25(3):420–430. doi: 10.1111/j.1432-1033.1972.tb01711.x. [DOI] [PubMed] [Google Scholar]
- Weisiger R. A., Fridovich I. Superoxide dismutase. Organelle specificity. J Biol Chem. 1973 May 25;248(10):3582–3592. [PubMed] [Google Scholar]
- Yim M. B., Berlett B. S., Chock P. B., Stadtman E. R. Manganese(II)-bicarbonate-mediated catalytic activity for hydrogen peroxide dismutation and amino acid oxidation: detection of free radical intermediates. Proc Natl Acad Sci U S A. 1990 Jan;87(1):394–398. doi: 10.1073/pnas.87.1.394. [DOI] [PMC free article] [PubMed] [Google Scholar]