Abstract
Polymerization of the deoxy form of sickle cell hemoglobin (Hb S; beta 6 Glu----Val) involves both hydrophobic and electrostatic intermolecular contacts. These interactions drive the mutated molecules into long fibrous rods composed of seven pairs of strands. X-ray crystallography of Hb S and electron microscopy image reconstruction of the fibers have revealed the remarkable complementarity between one of the beta 6 valines of each molecule (the donor site) and an acceptor site at the EF corner of a neighboring tetramer. This interaction constitutes the major lateral contact between the two strands in a pair. To estimate the relative importance of this key hydrophobic contact in polymer formation we have generated a polymerizing Hb with isoleucine at the beta 6 position (beta E6I) by site-directed mutagenesis. The mutated beta chains were produced in Escherichia coli and reassembled into functional tetramers with native alpha chains. Compared to native Hb S, the beta E6I mutant polymerizes faster and with a shortened delay time in 1.8 M phosphate buffer, indicating an increased stability of the nuclei preceding fiber growth. The solubility of the beta E6I mutant Hb is half that of native Hb S. Computer modeling of the donor-acceptor interaction shows that the presence of an isoleucine side chain at the donor site induces increased contacts with the receptor site and an increased buried surface area, in agreement with the higher hydrophobicity of the isoleucine residue. The agreement between the predicted and experimental differences in solubility suggests that the transfer of the beta 6 valine or isoleucine side chain from water to a hydrophobic environment is sufficient to explain the observations.
Full text
PDF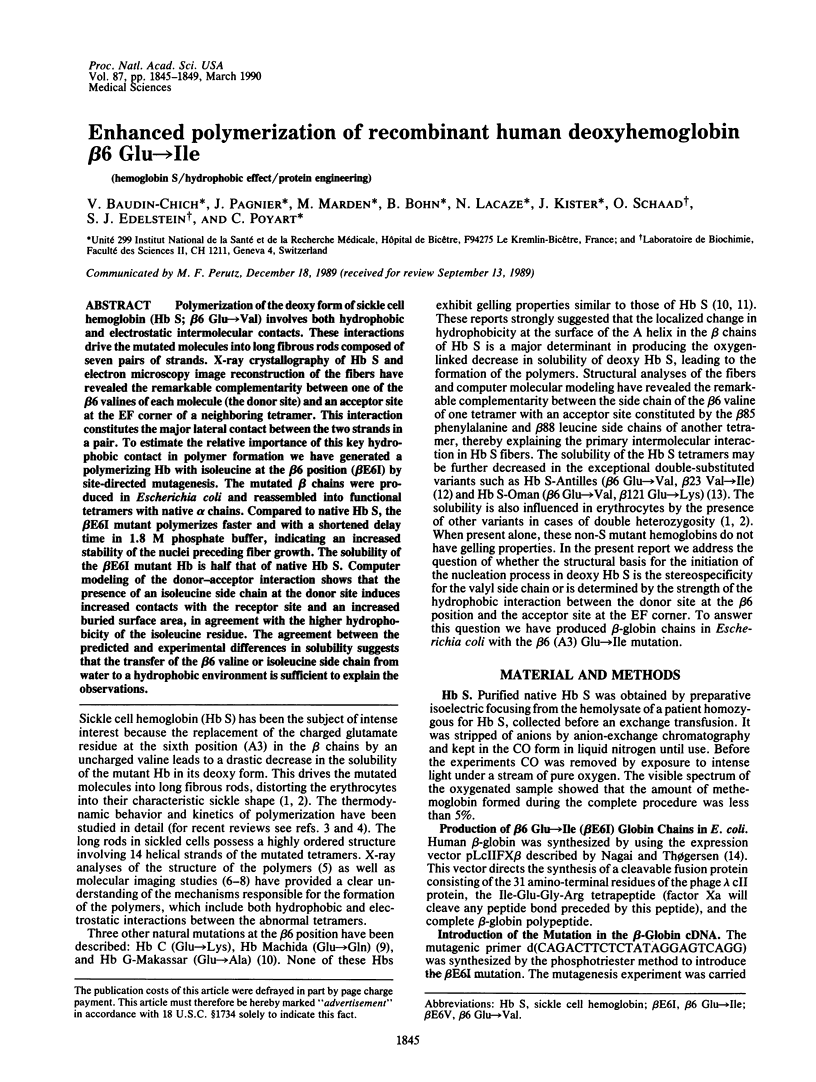
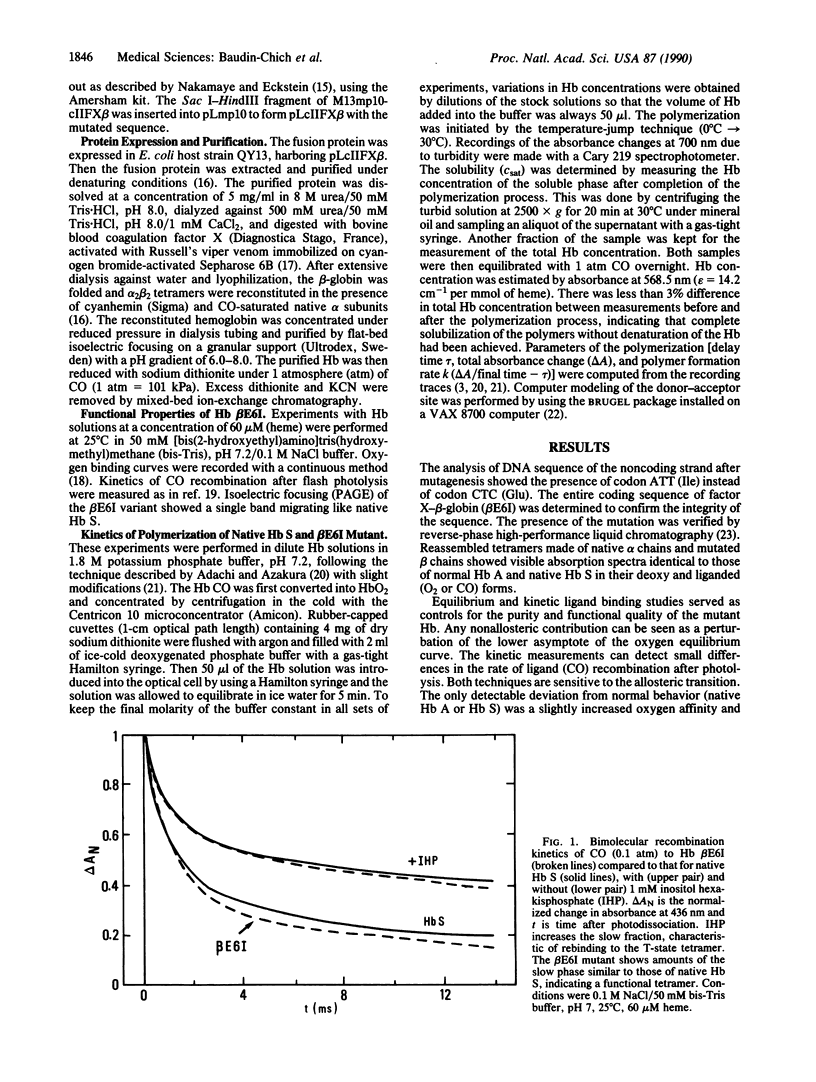
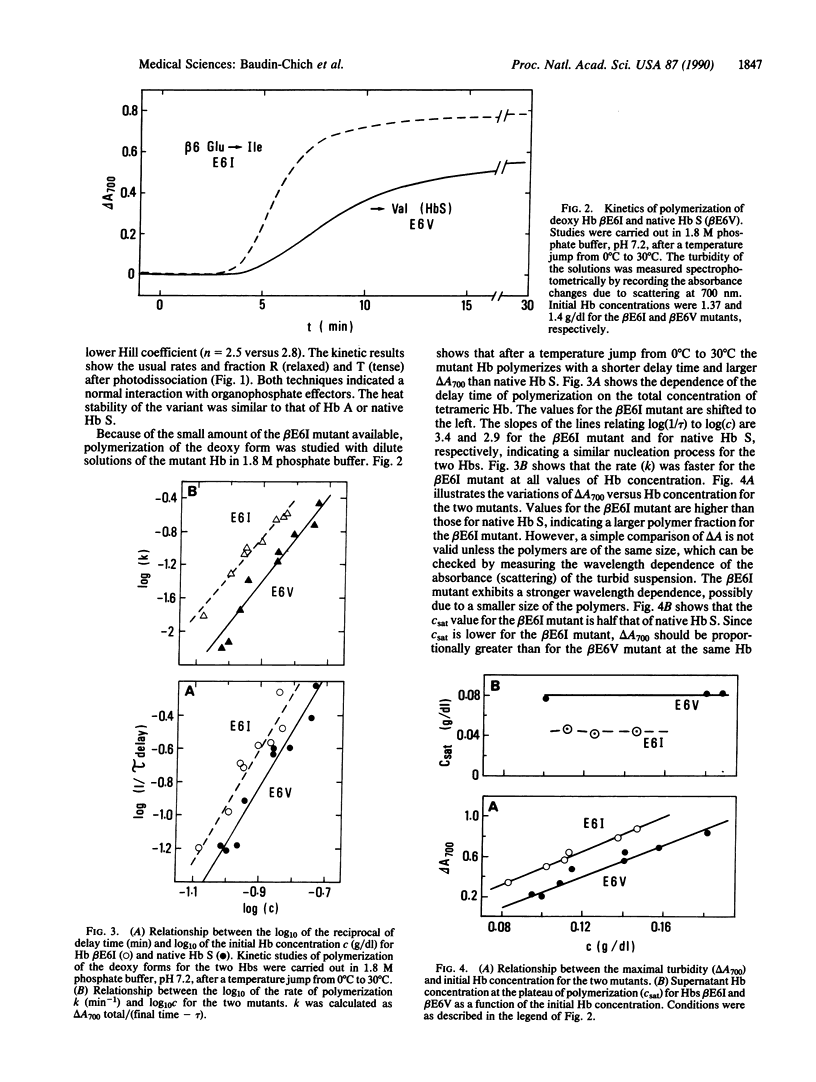
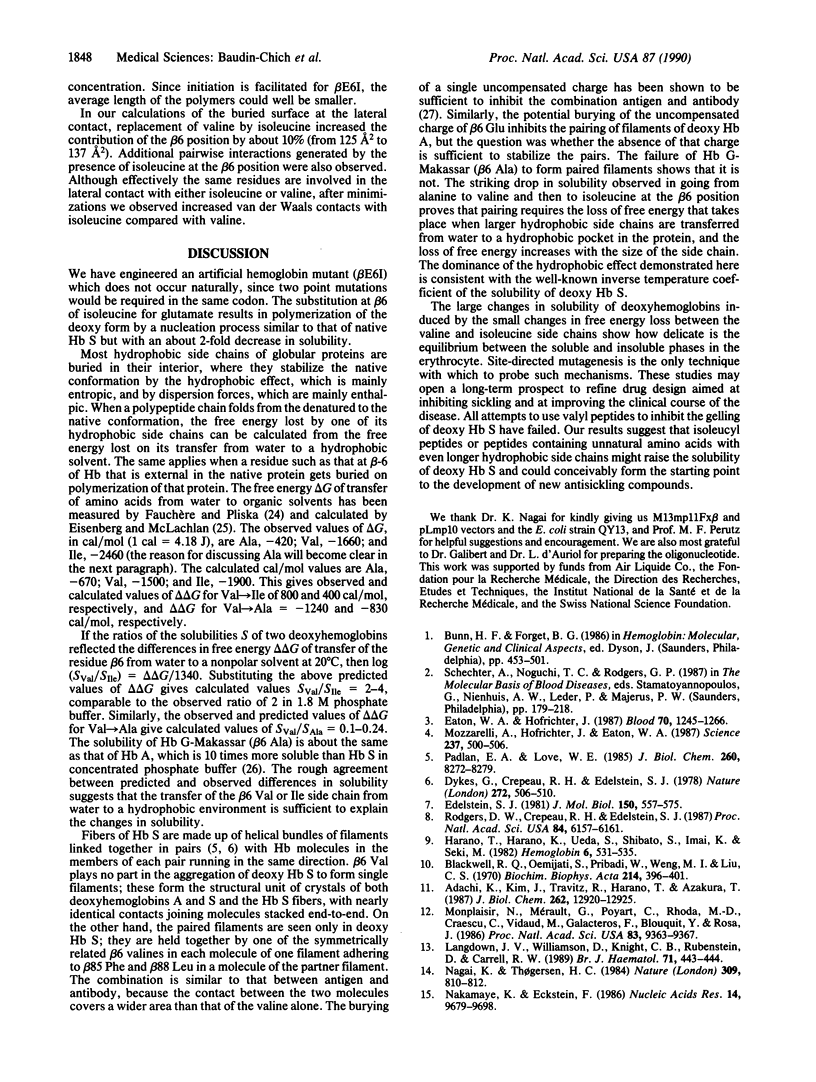
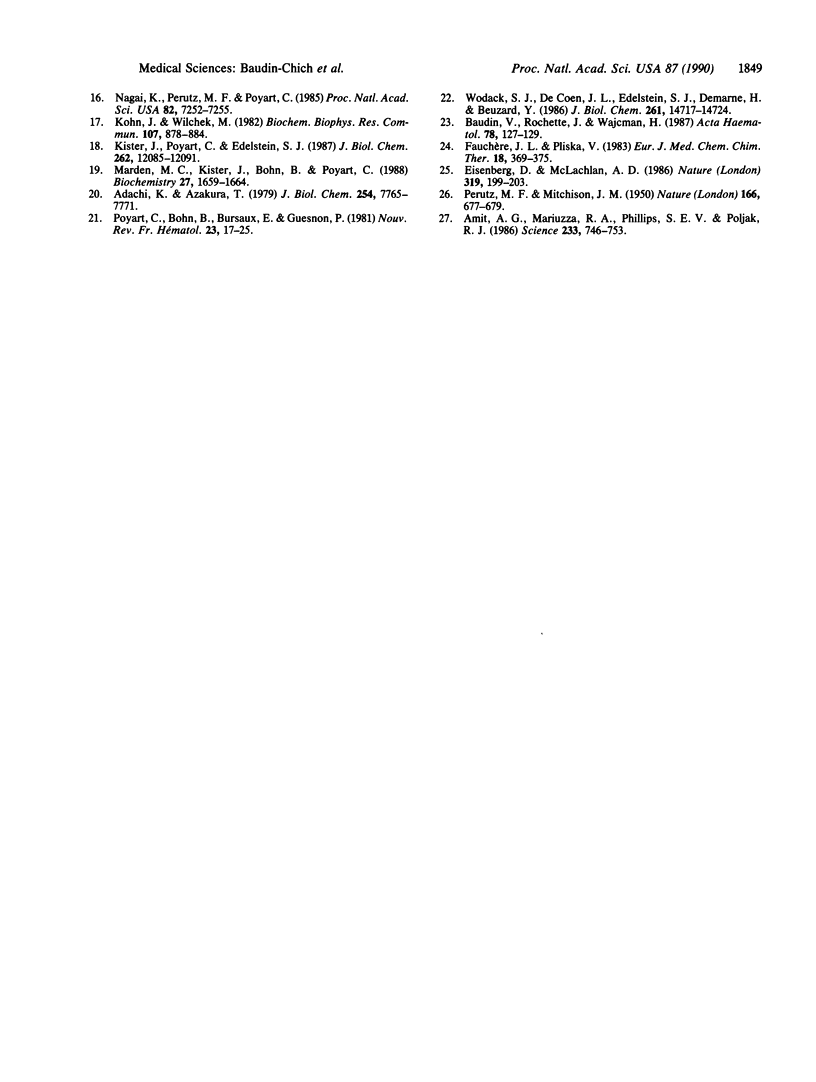
Selected References
These references are in PubMed. This may not be the complete list of references from this article.
- Adachi K., Asakura T. Nucleation-controlled aggregation of deoxyhemoglobin S. Possible difference in the size of nuclei in different phosphate concentrations. J Biol Chem. 1979 Aug 25;254(16):7765–7771. [PubMed] [Google Scholar]
- Adachi K., Kim J., Travitz R., Harano T., Asakura T. Effect of amino acid at the beta 6 position on surface hydrophobicity, stability, solubility, and the kinetics of polymerization of hemoglobin. Comparisons among Hb A (Glu beta 6), Hb C (Lys beta 6), Hb Machida (Gln beta 6), and Hb S (Val beta 6). J Biol Chem. 1987 Sep 25;262(27):12920–12925. [PubMed] [Google Scholar]
- Amit A. G., Mariuzza R. A., Phillips S. E., Poljak R. J. Three-dimensional structure of an antigen-antibody complex at 2.8 A resolution. Science. 1986 Aug 15;233(4765):747–753. doi: 10.1126/science.2426778. [DOI] [PubMed] [Google Scholar]
- Baudin-Chich V., Rochette J., Wajcman H. Strategy for structural characterization of haemoglobin variants. Acta Haematol. 1987;78(2-3):127–129. doi: 10.1159/000205860. [DOI] [PubMed] [Google Scholar]
- Blackwell R. Q., Oemijati S., Pribadi W., Weng M. I., Liu C. S. Hemoglobin G Makassar: beta-6 Glu leads to Ala. Biochim Biophys Acta. 1970 Sep 29;214(3):396–401. [PubMed] [Google Scholar]
- Dykes G., Crepeau R. H., Edelstein S. J. Three-dimensional reconstruction of the fibres of sickle cell haemoglobin. Nature. 1978 Apr 6;272(5653):506–510. doi: 10.1038/272506a0. [DOI] [PubMed] [Google Scholar]
- Eaton W. A., Hofrichter J. Hemoglobin S gelation and sickle cell disease. Blood. 1987 Nov;70(5):1245–1266. [PubMed] [Google Scholar]
- Edelstein S. J. Molecular topology in crystals and fibers of hemoglobin S. J Mol Biol. 1981 Aug 25;150(4):557–575. doi: 10.1016/0022-2836(81)90381-8. [DOI] [PubMed] [Google Scholar]
- Eisenberg D., McLachlan A. D. Solvation energy in protein folding and binding. Nature. 1986 Jan 16;319(6050):199–203. doi: 10.1038/319199a0. [DOI] [PubMed] [Google Scholar]
- Harano T., Harano K., Ueda S., Shibata S., Imai K., Seki M. Hemoglobin Machida [beta 6 (A3) Glu replaced by Gln], a new abnormal hemoglobin discovered in a Japanese family: structure, function and biosynthesis. Hemoglobin. 1982;6(5):531–535. doi: 10.3109/03630268209083766. [DOI] [PubMed] [Google Scholar]
- Kister J., Poyart C., Edelstein S. J. An expanded two-state allosteric model for interactions of human hemoglobin A with nonsaturating concentrations of 2,3-diphosphoglycerate. J Biol Chem. 1987 Sep 5;262(25):12085–12091. [PubMed] [Google Scholar]
- Kohn J., Wilchek M. A new approach (cyano-transfer) for cyanogen bromide activation of Sepharose at neutral pH, which yields activated resins, free of interfering nitrogen derivatives. Biochem Biophys Res Commun. 1982 Aug;107(3):878–884. doi: 10.1016/0006-291x(82)90604-0. [DOI] [PubMed] [Google Scholar]
- Langdown J. V., Williamson D., Knight C. B., Rubenstein D., Carrell R. W. A new doubly substituted sickling haemoglobin: HbS-Oman. Br J Haematol. 1989 Mar;71(3):443–444. doi: 10.1111/j.1365-2141.1989.tb04304.x. [DOI] [PubMed] [Google Scholar]
- Marden M. C., Kister J., Bohn B., Poyart C. T-state hemoglobin with four ligands bound. Biochemistry. 1988 Mar 8;27(5):1659–1664. doi: 10.1021/bi00405a041. [DOI] [PubMed] [Google Scholar]
- Monplaisir N., Merault G., Poyart C., Rhoda M. D., Craescu C., Vidaud M., Galacteros F., Blouquit Y., Rosa J. Hemoglobin S Antilles: a variant with lower solubility than hemoglobin S and producing sickle cell disease in heterozygotes. Proc Natl Acad Sci U S A. 1986 Dec;83(24):9363–9367. doi: 10.1073/pnas.83.24.9363. [DOI] [PMC free article] [PubMed] [Google Scholar]
- Mozzarelli A., Hofrichter J., Eaton W. A. Delay time of hemoglobin S polymerization prevents most cells from sickling in vivo. Science. 1987 Jul 31;237(4814):500–506. doi: 10.1126/science.3603036. [DOI] [PubMed] [Google Scholar]
- Nagai K., Perutz M. F., Poyart C. Oxygen binding properties of human mutant hemoglobins synthesized in Escherichia coli. Proc Natl Acad Sci U S A. 1985 Nov;82(21):7252–7255. doi: 10.1073/pnas.82.21.7252. [DOI] [PMC free article] [PubMed] [Google Scholar]
- Nagai K., Thøgersen H. C. Generation of beta-globin by sequence-specific proteolysis of a hybrid protein produced in Escherichia coli. 1984 Jun 28-Jul 4Nature. 309(5971):810–812. doi: 10.1038/309810a0. [DOI] [PubMed] [Google Scholar]
- Nakamaye K. L., Eckstein F. Inhibition of restriction endonuclease Nci I cleavage by phosphorothioate groups and its application to oligonucleotide-directed mutagenesis. Nucleic Acids Res. 1986 Dec 22;14(24):9679–9698. doi: 10.1093/nar/14.24.9679. [DOI] [PMC free article] [PubMed] [Google Scholar]
- PERUTZ M. F., MITCHISON J. M. State of haemoglobin in sickle-cell anaemia. Nature. 1950 Oct 21;166(4225):677–679. doi: 10.1038/166677a0. [DOI] [PubMed] [Google Scholar]
- Padlan E. A., Love W. E. Refined crystal structure of deoxyhemoglobin S. I. Restrained least-squares refinement at 3.0-A resolution. J Biol Chem. 1985 Jul 15;260(14):8272–8279. doi: 10.2210/pdb1hbs/pdb. [DOI] [PubMed] [Google Scholar]
- Rodgers D. W., Crepeau R. H., Edelstein S. J. Pairings and polarities of the 14 strands in sickle cell hemoglobin fibers. Proc Natl Acad Sci U S A. 1987 Sep;84(17):6157–6161. doi: 10.1073/pnas.84.17.6157. [DOI] [PMC free article] [PubMed] [Google Scholar]
- Wodak S. J., De Coen J. L., Edelstein S. J., Demarne H., Beuzard Y. Modification of human hemoglobin by glutathione. III. Perturbations of hemoglobin conformation analyzed by computer modeling. J Biol Chem. 1986 Nov 5;261(31):14717–14724. [PubMed] [Google Scholar]