Abstract
An in vitro system has been developed to study the mechanism by which fibronectin (FN) regulates capillary endothelial cell growth in the presence of soluble angiogenic mitogens. Endothelial cells were cultured in chemically defined medium containing a constant, saturating amount of basic fibroblast growth factor. Formation of cell-FN contacts was then varied in a controlled fashion by three different techniques: (i) nonadhesive, bacteriological dishes were precoated with increasing densities of FN; (ii) soluble RGD peptides were used to progressively inhibit binding of cell-surface integrin receptors to adsorbed FN; and (iii) FN-coated surfaces were covered with increasingly thick layers of polyhydroxyethylmethacrylate (a nonadhesive polymer) to physically restrict cell access to FN binding sites. Endothelial cells became more extended and proliferated more rapidly as FN coating concentrations were raised from approximately 250 to approximately 10,000 FN molecules per micron 2. Computerized morphometric analysis confirmed that cell shape (projected cell areas) was determined by the density of FN contacts and that DNA synthetic levels were tightly coupled to the extent of cell spreading, regardless of the method used to perturb cell adhesion. In contrast, neither soluble FN nor cell-surface binding of FN-coated microbeads (diameter, 4.5 microns) had any effect on growth when cells were grown in suspension and cell spreading was prohibited. These results suggest that FN controls capillary endothelial cell proliferation based on its ability to support tension-dependent alterations of cell shape--i.e., both by binding to cell-surface integrins and by resisting mechanical loads that are applied to these receptors.
Full text
PDF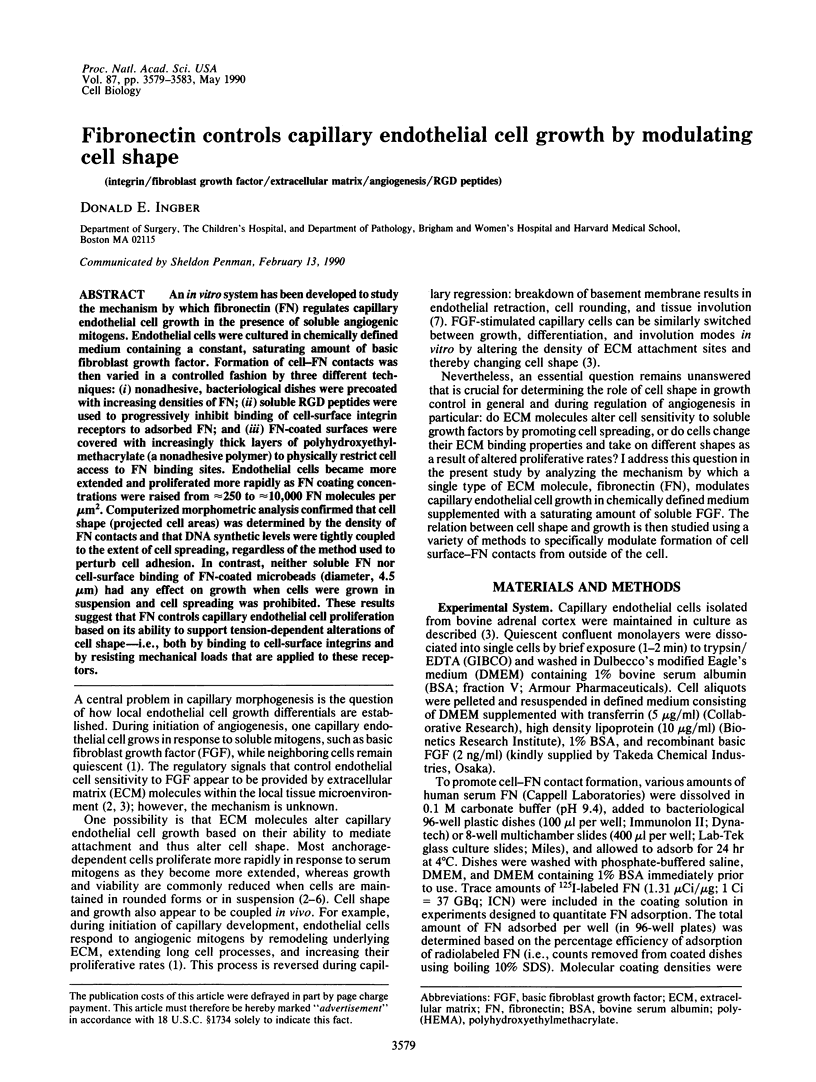
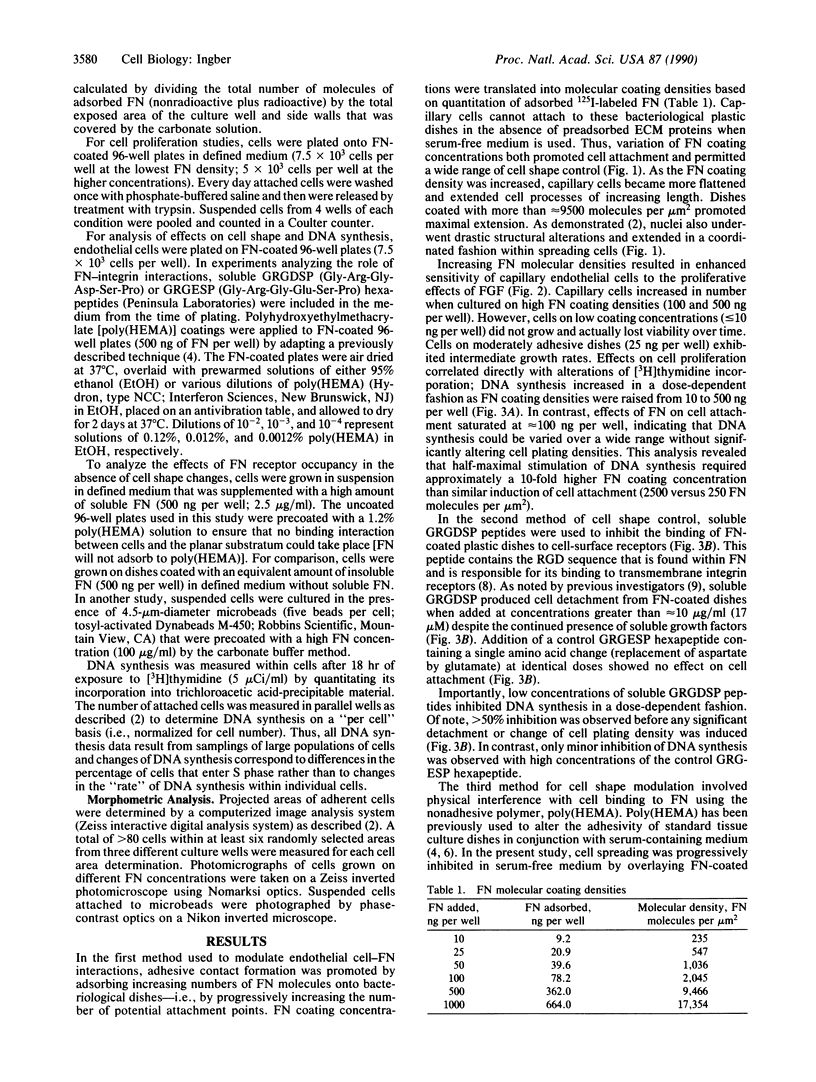
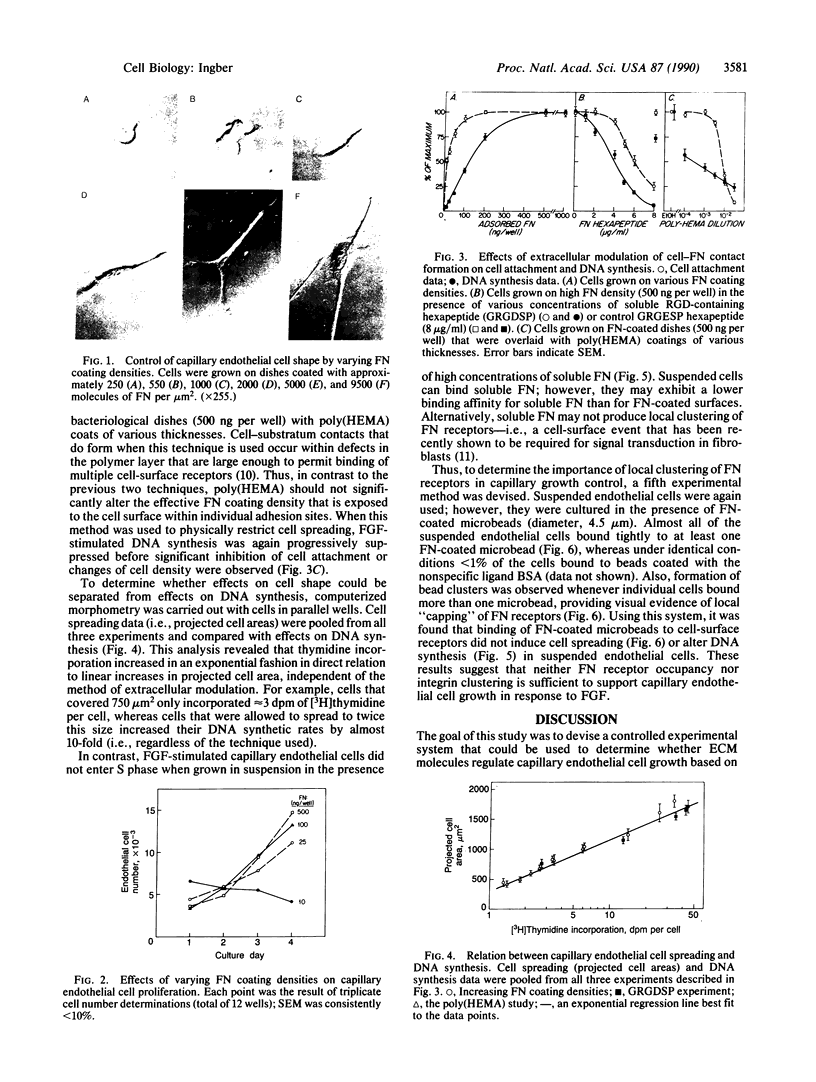
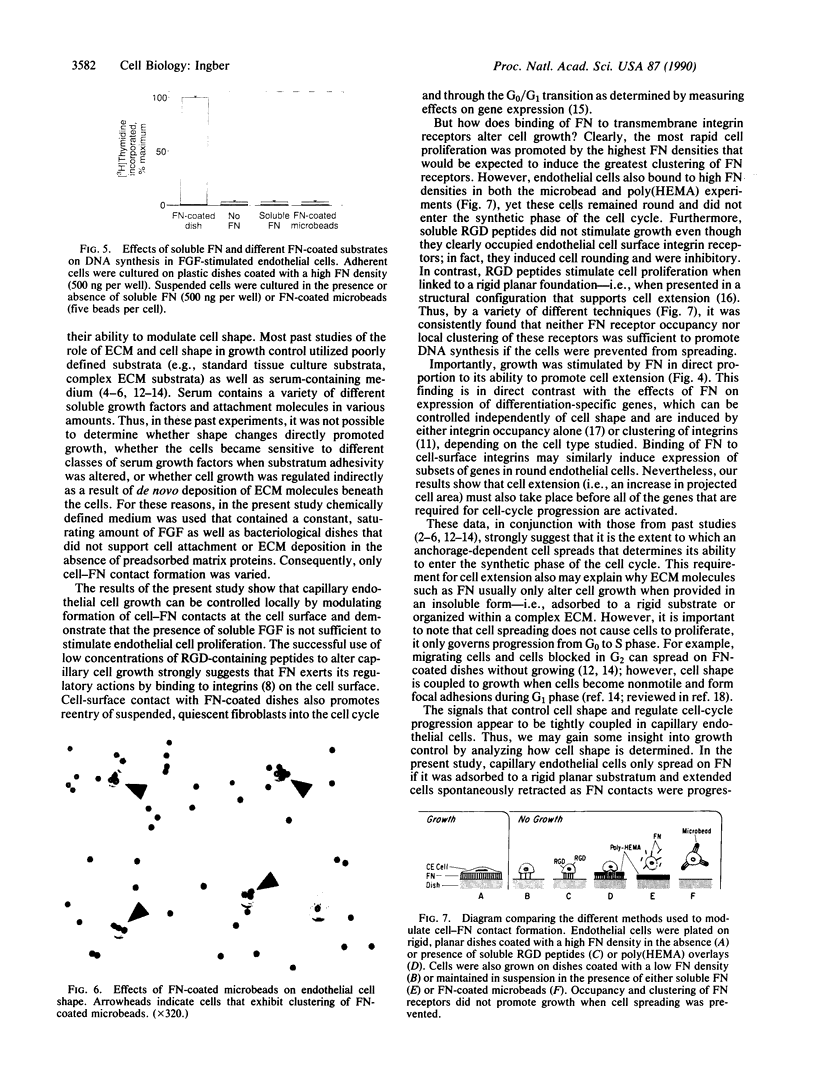
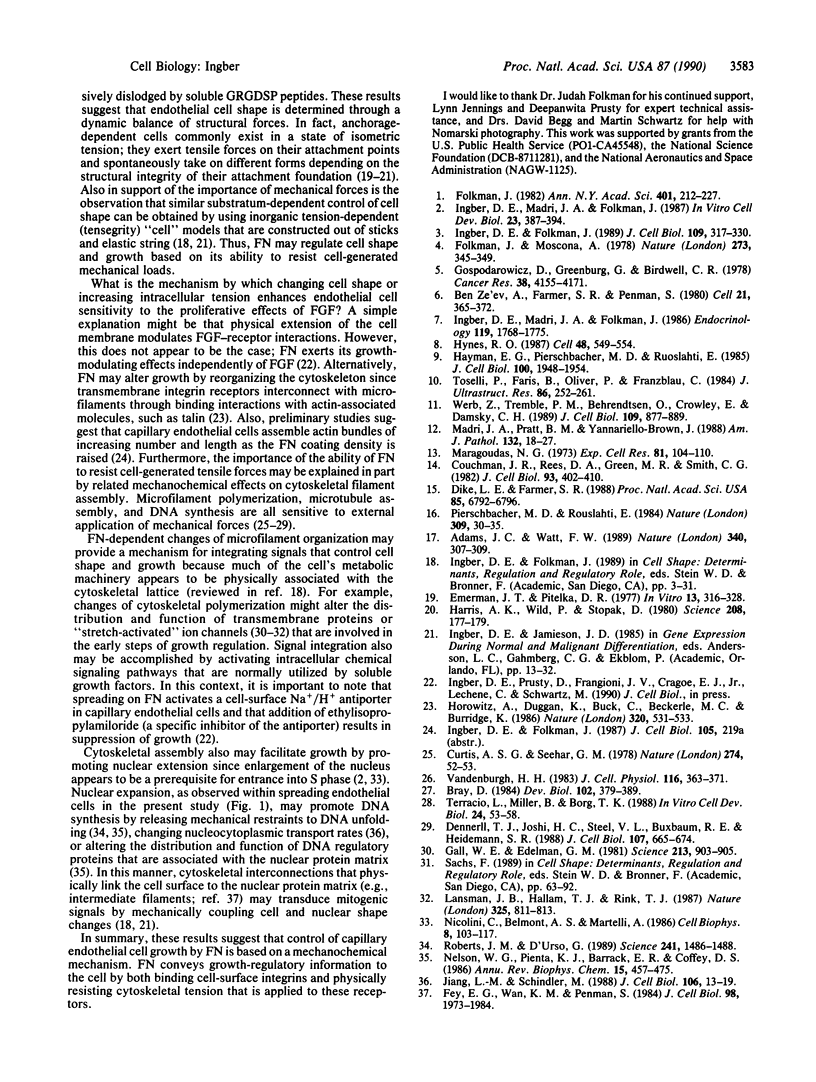
Images in this article
Selected References
These references are in PubMed. This may not be the complete list of references from this article.
- Adams J. C., Watt F. M. Fibronectin inhibits the terminal differentiation of human keratinocytes. Nature. 1989 Jul 27;340(6231):307–309. doi: 10.1038/340307a0. [DOI] [PubMed] [Google Scholar]
- Ben-Ze'ev A., Farmer S. R., Penman S. Protein synthesis requires cell-surface contact while nuclear events respond to cell shape in anchorage-dependent fibroblasts. Cell. 1980 Sep;21(2):365–372. doi: 10.1016/0092-8674(80)90473-0. [DOI] [PubMed] [Google Scholar]
- Bray D. Axonal growth in response to experimentally applied mechanical tension. Dev Biol. 1984 Apr;102(2):379–389. doi: 10.1016/0012-1606(84)90202-1. [DOI] [PubMed] [Google Scholar]
- Couchman J. R., Rees D. A., Green M. R., Smith C. G. Fibronectin has a dual role in locomotion and anchorage of primary chick fibroblasts and can promote entry into the division cycle. J Cell Biol. 1982 May;93(2):402–410. doi: 10.1083/jcb.93.2.402. [DOI] [PMC free article] [PubMed] [Google Scholar]
- Curtis A. S., Seehar G. M. The control of cell division by tension or diffusion. Nature. 1978 Jul 6;274(5666):52–53. doi: 10.1038/274052a0. [DOI] [PubMed] [Google Scholar]
- Dennerll T. J., Joshi H. C., Steel V. L., Buxbaum R. E., Heidemann S. R. Tension and compression in the cytoskeleton of PC-12 neurites. II: Quantitative measurements. J Cell Biol. 1988 Aug;107(2):665–674. doi: 10.1083/jcb.107.2.665. [DOI] [PMC free article] [PubMed] [Google Scholar]
- Dike L. E., Farmer S. R. Cell adhesion induces expression of growth-associated genes in suspension-arrested fibroblasts. Proc Natl Acad Sci U S A. 1988 Sep;85(18):6792–6796. doi: 10.1073/pnas.85.18.6792. [DOI] [PMC free article] [PubMed] [Google Scholar]
- Emerman J. T., Pitelka D. R. Maintenance and induction of morphological differentiation in dissociated mammary epithelium on floating collagen membranes. In Vitro. 1977 May;13(5):316–328. doi: 10.1007/BF02616178. [DOI] [PubMed] [Google Scholar]
- Fey E. G., Wan K. M., Penman S. Epithelial cytoskeletal framework and nuclear matrix-intermediate filament scaffold: three-dimensional organization and protein composition. J Cell Biol. 1984 Jun;98(6):1973–1984. doi: 10.1083/jcb.98.6.1973. [DOI] [PMC free article] [PubMed] [Google Scholar]
- Folkman J. Angiogenesis: initiation and control. Ann N Y Acad Sci. 1982;401:212–227. doi: 10.1111/j.1749-6632.1982.tb25720.x. [DOI] [PubMed] [Google Scholar]
- Folkman J., Moscona A. Role of cell shape in growth control. Nature. 1978 Jun 1;273(5661):345–349. doi: 10.1038/273345a0. [DOI] [PubMed] [Google Scholar]
- Gall W. E., Edelman G. M. Lateral diffusion of surface molecules in animal cells and tissues. Science. 1981 Aug 21;213(4510):903–905. doi: 10.1126/science.7196087. [DOI] [PubMed] [Google Scholar]
- Gospodarowicz D., Greenburg G., Birdwell C. R. Determination of cellular shape by the extracellular matrix and its correlation with the control of cellular growth. Cancer Res. 1978 Nov;38(11 Pt 2):4155–4171. [PubMed] [Google Scholar]
- Harris A. K., Wild P., Stopak D. Silicone rubber substrata: a new wrinkle in the study of cell locomotion. Science. 1980 Apr 11;208(4440):177–179. doi: 10.1126/science.6987736. [DOI] [PubMed] [Google Scholar]
- Hayman E. G., Pierschbacher M. D., Ruoslahti E. Detachment of cells from culture substrate by soluble fibronectin peptides. J Cell Biol. 1985 Jun;100(6):1948–1954. doi: 10.1083/jcb.100.6.1948. [DOI] [PMC free article] [PubMed] [Google Scholar]
- Horwitz A., Duggan K., Buck C., Beckerle M. C., Burridge K. Interaction of plasma membrane fibronectin receptor with talin--a transmembrane linkage. Nature. 1986 Apr 10;320(6062):531–533. doi: 10.1038/320531a0. [DOI] [PubMed] [Google Scholar]
- Hynes R. O. Integrins: a family of cell surface receptors. Cell. 1987 Feb 27;48(4):549–554. doi: 10.1016/0092-8674(87)90233-9. [DOI] [PubMed] [Google Scholar]
- Ingber D. E., Folkman J. Mechanochemical switching between growth and differentiation during fibroblast growth factor-stimulated angiogenesis in vitro: role of extracellular matrix. J Cell Biol. 1989 Jul;109(1):317–330. doi: 10.1083/jcb.109.1.317. [DOI] [PMC free article] [PubMed] [Google Scholar]
- Ingber D. E., Madri J. A., Folkman J. A possible mechanism for inhibition of angiogenesis by angiostatic steroids: induction of capillary basement membrane dissolution. Endocrinology. 1986 Oct;119(4):1768–1775. doi: 10.1210/endo-119-4-1768. [DOI] [PubMed] [Google Scholar]
- Ingber D. E., Madri J. A., Folkman J. Endothelial growth factors and extracellular matrix regulate DNA synthesis through modulation of cell and nuclear expansion. In Vitro Cell Dev Biol. 1987 May;23(5):387–394. doi: 10.1007/BF02620997. [DOI] [PubMed] [Google Scholar]
- Jiang L. W., Schindler M. Nuclear transport in 3T3 fibroblasts: effects of growth factors, transformation, and cell shape. J Cell Biol. 1988 Jan;106(1):13–19. doi: 10.1083/jcb.106.1.13. [DOI] [PMC free article] [PubMed] [Google Scholar]
- Lansman J. B., Hallam T. J., Rink T. J. Single stretch-activated ion channels in vascular endothelial cells as mechanotransducers? 1987 Feb 26-Mar 4Nature. 325(6107):811–813. doi: 10.1038/325811a0. [DOI] [PubMed] [Google Scholar]
- Madri J. A., Pratt B. M., Yannariello-Brown J. Matrix-driven cell size change modulates aortic endothelial cell proliferation and sheet migration. Am J Pathol. 1988 Jul;132(1):18–27. [PMC free article] [PubMed] [Google Scholar]
- Maroudas N. G. Growth of fibroblasts on linear and planar anchorages of limiting dimensions. Exp Cell Res. 1973 Sep;81(1):104–110. doi: 10.1016/0014-4827(73)90116-x. [DOI] [PubMed] [Google Scholar]
- Nelson W. G., Pienta K. J., Barrack E. R., Coffey D. S. The role of the nuclear matrix in the organization and function of DNA. Annu Rev Biophys Biophys Chem. 1986;15:457–475. doi: 10.1146/annurev.bb.15.060186.002325. [DOI] [PubMed] [Google Scholar]
- Nicolini C., Belmont A. S., Martelli A. Critical nuclear DNA size and distribution associated with S phase initiation. Peripheral location of initiation and termination sites. Cell Biophys. 1986 Apr;8(2):103–117. doi: 10.1007/BF02788475. [DOI] [PubMed] [Google Scholar]
- Pierschbacher M. D., Ruoslahti E. Cell attachment activity of fibronectin can be duplicated by small synthetic fragments of the molecule. Nature. 1984 May 3;309(5963):30–33. doi: 10.1038/309030a0. [DOI] [PubMed] [Google Scholar]
- Roberts J. M., D'Urso G. An origin unwinding activity regulates initiation of DNA replication during mammalian cell cycle. Science. 1988 Sep 16;241(4872):1486–1489. doi: 10.1126/science.2843984. [DOI] [PubMed] [Google Scholar]
- Terracio L., Miller B., Borg T. K. Effects of cyclic mechanical stimulation of the cellular components of the heart: in vitro. In Vitro Cell Dev Biol. 1988 Jan;24(1):53–58. doi: 10.1007/BF02623815. [DOI] [PubMed] [Google Scholar]
- Toselli P., Faris B., Oliver P., Franzblau C. Ultrastructural studies of attachment site formation in aortic smooth muscle cells cultured on collagen-hydroxyethylmethacrylate hydrogels. J Ultrastruct Res. 1984 Mar;86(3):252–261. doi: 10.1016/s0022-5320(84)90105-9. [DOI] [PubMed] [Google Scholar]
- Vandenburgh H. H. Cell shape and growth regulation in skeletal muscle: exogenous versus endogenous factors. J Cell Physiol. 1983 Sep;116(3):363–371. doi: 10.1002/jcp.1041160314. [DOI] [PubMed] [Google Scholar]
- Werb Z., Tremble P. M., Behrendtsen O., Crowley E., Damsky C. H. Signal transduction through the fibronectin receptor induces collagenase and stromelysin gene expression. J Cell Biol. 1989 Aug;109(2):877–889. doi: 10.1083/jcb.109.2.877. [DOI] [PMC free article] [PubMed] [Google Scholar]