Abstract
Arginyl-tRNA synthetase (arginine-tRNA ligase, EC 6.1.1.19) is found in extracts of mammalian cells both as a free protein (Mr = 60,000) and as a component (Mr approximately 72,000) of the high molecular weight aminoacyl-tRNA synthetase complex (Mr greater than 10(6). Several pieces of evidence indicate that the low molecular weight free form is not a proteolytic degradation product of the complex-bound enzyme but that it preexists in vivo: (i) the endogenous free form differs in size from the active proteolytic fragment generated in vitro, (ii) conditions expected to increase or decrease the amount of proteolysis do not alter the ratio of the two forms of the enzyme, and (iii) the free form contains an NH2-terminal methionine residue. A model is presented that provides a rationale for the existence of two forms of arginyl-tRNA synthetase in cells. In this model the complexed enzyme supplies arginyl-tRNA for protein synthesis, whereas the free enzyme provides arginyl-tRNA for the NH2-terminal arginine modification of proteins by arginyl-tRNA:protein arginyltransferase. This latter process targets certain proteins for removal by the ubiquitin-dependent protein degradation pathway. The necessity for an additional pool of arginyl-tRNA for the modification reaction leads to the conclusion that the arginyl-tRNA destined for protein synthesis (and/or protein modification) is channeled and unavailable for other processes. Other evidence supporting channeling in protein synthesis is discussed.
Full text
PDF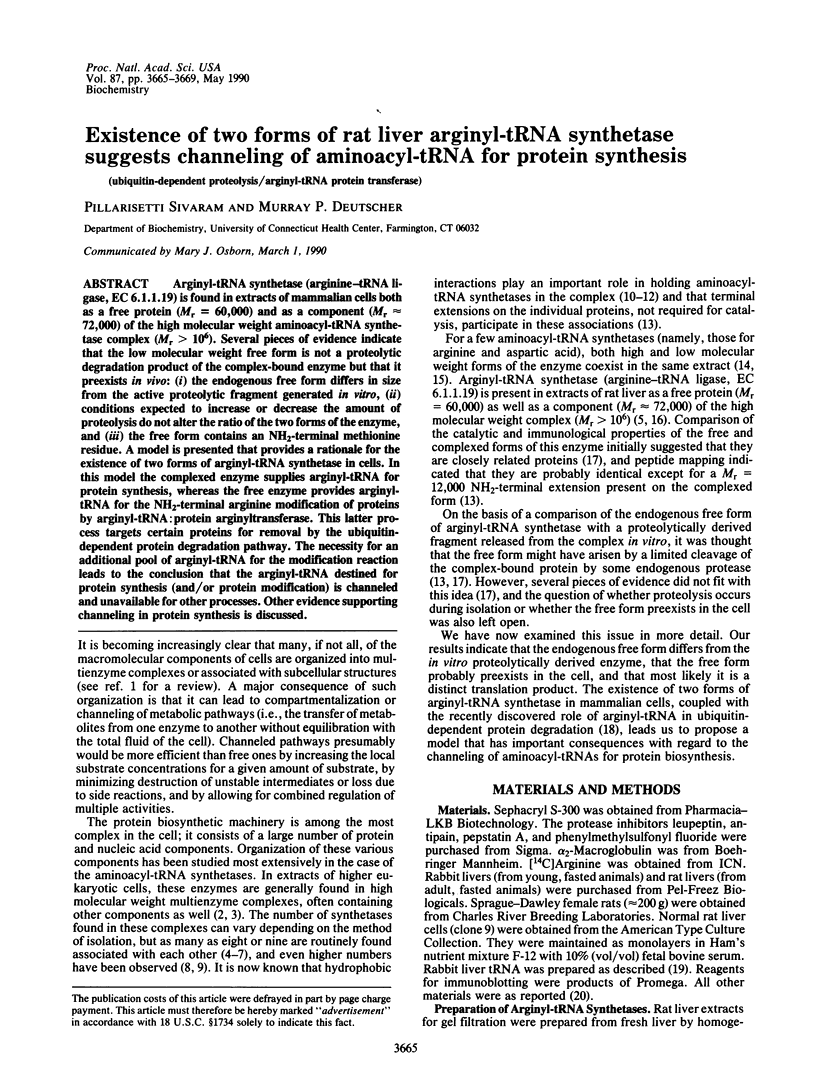
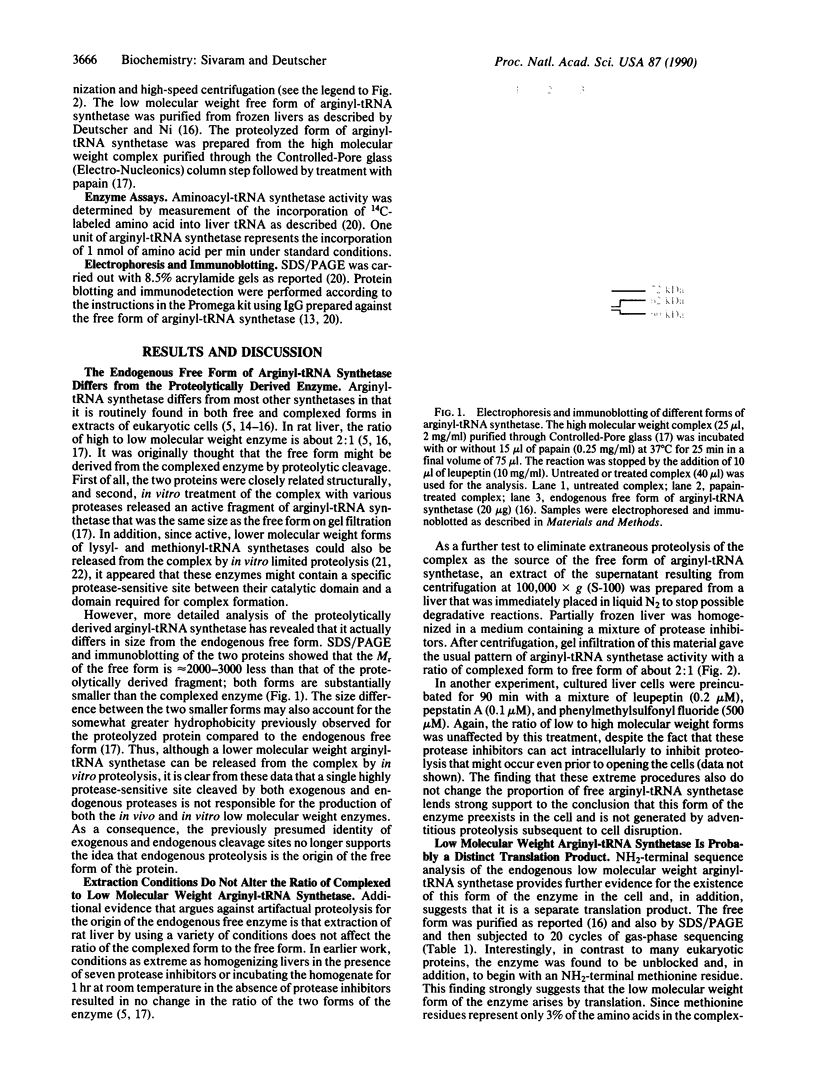
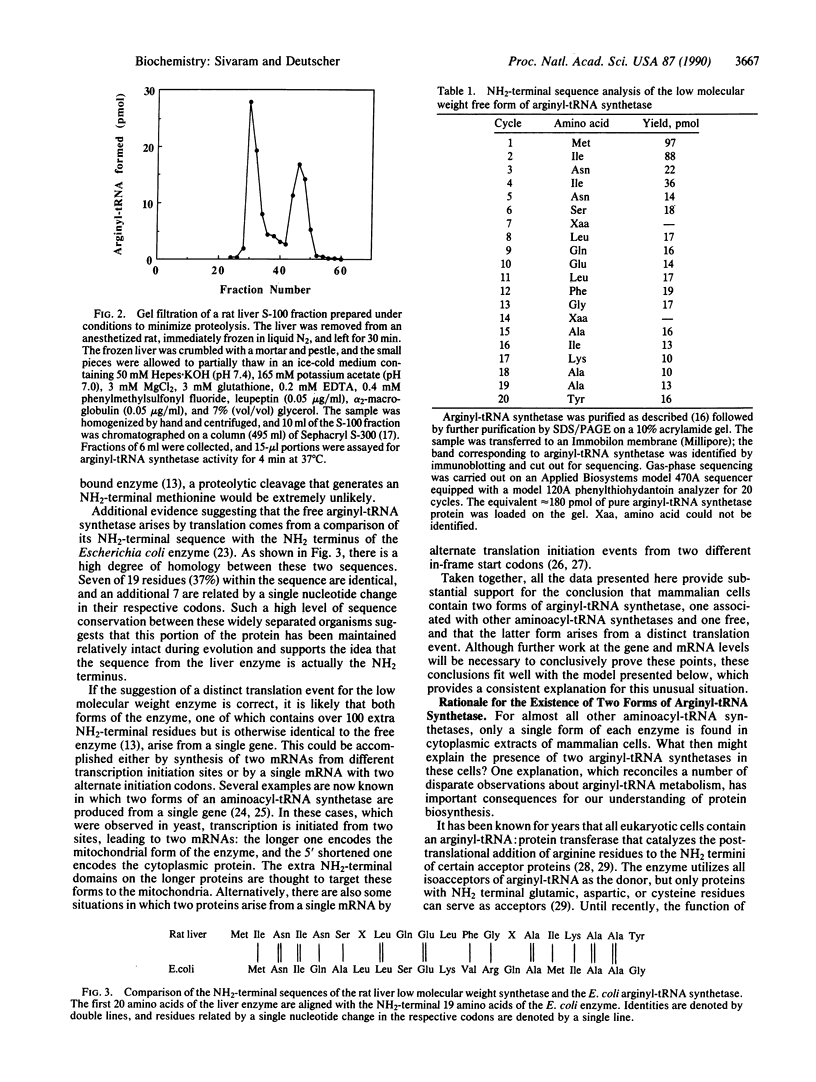
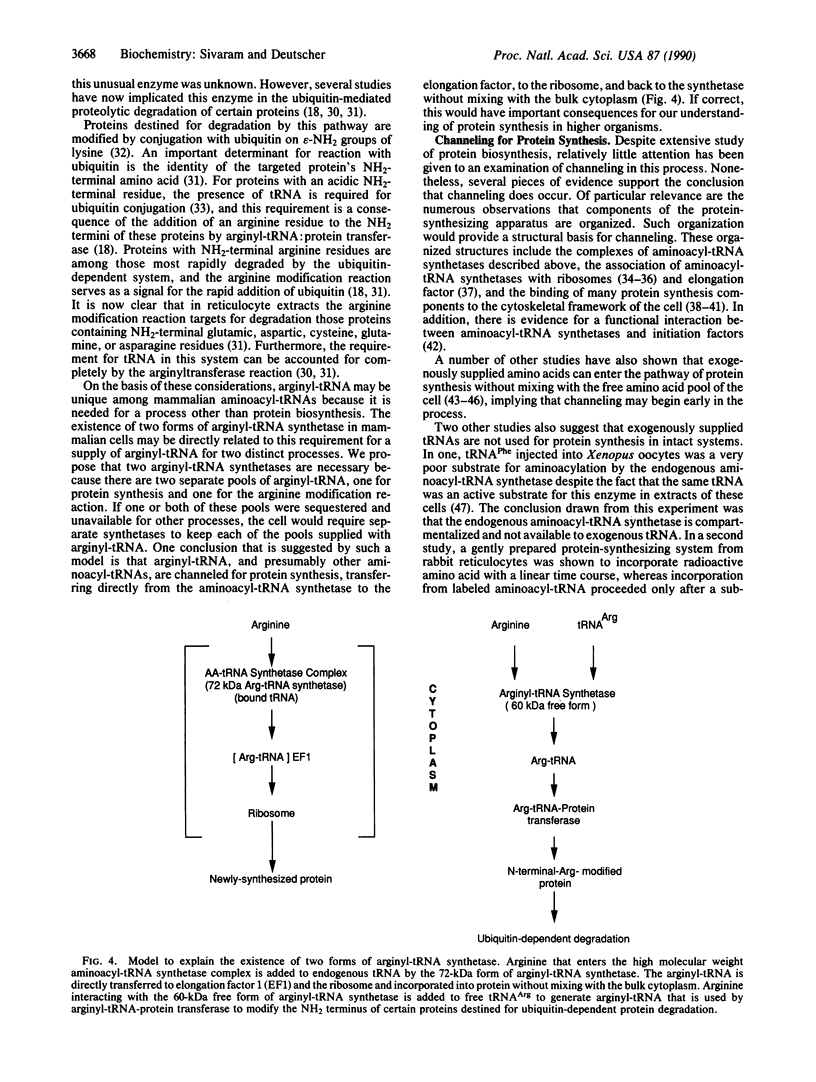
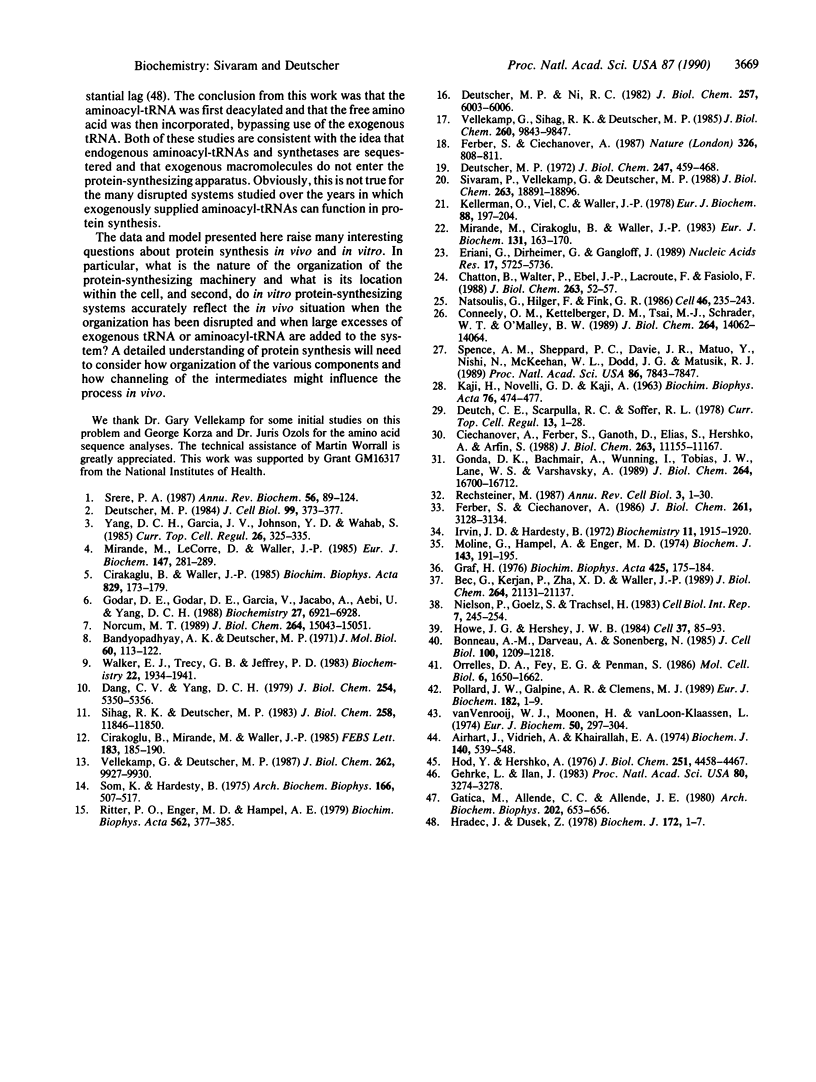
Images in this article
Selected References
These references are in PubMed. This may not be the complete list of references from this article.
- Bandyopadhyay A. K., Deutscher M. P. Complex of aminoacyl-transfer RNA synthetases. J Mol Biol. 1971 Aug 28;60(1):113–122. doi: 10.1016/0022-2836(71)90451-7. [DOI] [PubMed] [Google Scholar]
- Bec G., Kerjan P., Zha X. D., Waller J. P. Valyl-tRNA synthetase from rabbit liver. I. Purification as a heterotypic complex in association with elongation factor 1. J Biol Chem. 1989 Dec 15;264(35):21131–21137. [PubMed] [Google Scholar]
- Bonneau A. M., Darveau A., Sonenberg N. Effect of viral infection on host protein synthesis and mRNA association with the cytoplasmic cytoskeletal structure. J Cell Biol. 1985 Apr;100(4):1209–1218. doi: 10.1083/jcb.100.4.1209. [DOI] [PMC free article] [PubMed] [Google Scholar]
- Chatton B., Walter P., Ebel J. P., Lacroute F., Fasiolo F. The yeast VAS1 gene encodes both mitochondrial and cytoplasmic valyl-tRNA synthetases. J Biol Chem. 1988 Jan 5;263(1):52–57. [PubMed] [Google Scholar]
- Ciechanover A., Ferber S., Ganoth D., Elias S., Hershko A., Arfin S. Purification and characterization of arginyl-tRNA-protein transferase from rabbit reticulocytes. Its involvement in post-translational modification and degradation of acidic NH2 termini substrates of the ubiquitin pathway. J Biol Chem. 1988 Aug 15;263(23):11155–11167. [PubMed] [Google Scholar]
- Cirakoglu B., Mirande M., Waller J. P. A model for the structural organization of aminoacyl-tRNA synthetases in mammalian cells. FEBS Lett. 1985 Apr 22;183(2):185–190. [PubMed] [Google Scholar]
- Cirakoğlu B., Waller J. P. Multiple forms of arginyl- and lysyl-tRNA synthetases in rat liver: a re-evaluation. Biochim Biophys Acta. 1985 Jun 10;829(2):173–179. doi: 10.1016/0167-4838(85)90186-4. [DOI] [PubMed] [Google Scholar]
- Conneely O. M., Kettelberger D. M., Tsai M. J., Schrader W. T., O'Malley B. W. The chicken progesterone receptor A and B isoforms are products of an alternate translation initiation event. J Biol Chem. 1989 Aug 25;264(24):14062–14064. [PubMed] [Google Scholar]
- Deutch C. E., Scarpulla R. C., Soffer R. L. Posttranslational NH2-terminal aminoacylation. Curr Top Cell Regul. 1978;13:1–28. doi: 10.1016/b978-0-12-152813-3.50005-7. [DOI] [PubMed] [Google Scholar]
- Deutscher M. P., Ni R. C. Purification of a low molecular weight form of rat liver arginyl-tRNA synthetase. J Biol Chem. 1982 Jun 10;257(11):6003–6006. [PubMed] [Google Scholar]
- Deutscher M. P. Reactions at the 3' terminus of transfer ribonucleic acid. 3. Catalytic properties of two purified rabbit liver transfer ribonucleic acid nucleotidyl transferases. J Biol Chem. 1972 Jan 25;247(2):459–468. [PubMed] [Google Scholar]
- Deutscher M. P. The eucaryotic aminoacyl-tRNA synthetase complex: suggestions for its structure and function. J Cell Biol. 1984 Aug;99(2):373–377. doi: 10.1083/jcb.99.2.373. [DOI] [PMC free article] [PubMed] [Google Scholar]
- Eriani G., Dirheimer G., Gangloff J. Isolation and characterization of the gene coding for Escherichia coli arginyl-tRNA synthetase. Nucleic Acids Res. 1989 Jul 25;17(14):5725–5736. doi: 10.1093/nar/17.14.5725. [DOI] [PMC free article] [PubMed] [Google Scholar]
- Ferber S., Ciechanover A. Role of arginine-tRNA in protein degradation by the ubiquitin pathway. Nature. 1987 Apr 23;326(6115):808–811. doi: 10.1038/326808a0. [DOI] [PubMed] [Google Scholar]
- Ferber S., Ciechanover A. Transfer RNA is required for conjugation of ubiquitin to selective substrates of the ubiquitin- and ATP-dependent proteolytic system. J Biol Chem. 1986 Mar 5;261(7):3128–3134. [PubMed] [Google Scholar]
- Gatica M., Allende C. C., Allende J. E. Evidence for in vivo compartmentation of phenylalanyl-tRNA ligase in amphibian oocytes. Arch Biochem Biophys. 1980 Jul;202(2):653–656. doi: 10.1016/0003-9861(80)90474-9. [DOI] [PubMed] [Google Scholar]
- Gehrke L., Ilan J. Preferential utilization of exogenously supplied leucine for protein synthesis in estradiol-induced and uninduced cockerel liver explants. Proc Natl Acad Sci U S A. 1983 Jun;80(11):3274–3278. doi: 10.1073/pnas.80.11.3274. [DOI] [PMC free article] [PubMed] [Google Scholar]
- Godar D. E., Godar D. E., Garcia V., Jacobo A., Aebi U., Yang D. C. Structural organization of the multienzyme complex of mammalian aminoacyl-tRNA synthetases. Biochemistry. 1988 Sep 6;27(18):6921–6928. doi: 10.1021/bi00418a038. [DOI] [PubMed] [Google Scholar]
- Gonda D. K., Bachmair A., Wünning I., Tobias J. W., Lane W. S., Varshavsky A. Universality and structure of the N-end rule. J Biol Chem. 1989 Oct 5;264(28):16700–16712. [PubMed] [Google Scholar]
- Graf H. Intraction of aminoacyl-tRNA synthetases with ribosomes and ribosomal subunits. Biochim Biophys Acta. 1976 Mar 4;425(2):175–184. doi: 10.1016/0005-2787(76)90023-x. [DOI] [PubMed] [Google Scholar]
- Hod Y., Hershko A. Relationship of the pool of intracellular valine to protein synthesis and degradation in cultured cells. J Biol Chem. 1976 Jul 25;251(14):4458–4457. [PubMed] [Google Scholar]
- Howe J. G., Hershey J. W. Translational initiation factor and ribosome association with the cytoskeletal framework fraction from HeLa cells. Cell. 1984 May;37(1):85–93. doi: 10.1016/0092-8674(84)90303-9. [DOI] [PubMed] [Google Scholar]
- Hradec J., Dusek Z. All factors required for protein synthesis are retained on heparin bound to Sepharose. Biochem J. 1978 Apr 15;172(1):1–7. doi: 10.1042/bj1720001. [DOI] [PMC free article] [PubMed] [Google Scholar]
- Irvin J. D., Hardesty B. Binding of aminoacyl transfer ribonucleic acid synthetases to ribosomes from rabbit reticulocytes. Biochemistry. 1972 May 9;11(10):1915–1920. doi: 10.1021/bi00760a028. [DOI] [PubMed] [Google Scholar]
- KAJI H., NOVELLI G. D., KAJI A. A SOLUBLE AMINO ACID-INCORPORATING SYSTEM FROM RAT LIVER. Biochim Biophys Acta. 1963 Nov 22;76:474–477. [PubMed] [Google Scholar]
- Kellermann O., Viel C., Waller J. P. Methionyl-tRNA synthetase from sheep mammary gland. Purification of a fully active monomeric enzyme derived from high-molecular-weight complexes by controlled proteolysis. Eur J Biochem. 1978 Jul 17;88(1):197–204. doi: 10.1111/j.1432-1033.1978.tb12438.x. [DOI] [PubMed] [Google Scholar]
- Mirande M., Cirakoğlu B., Waller J. P. Seven mammalian aminoacyl-tRNA synthetases associated within the same complex are functionally independent. Eur J Biochem. 1983 Mar 1;131(1):163–170. doi: 10.1111/j.1432-1033.1983.tb07244.x. [DOI] [PubMed] [Google Scholar]
- Mirande M., Le Corre D., Waller J. P. A complex from cultured Chinese hamster ovary cells containing nine aminoacyl-tRNA synthetases. Thermolabile leucyl-tRNA synthetase from the tsH1 mutant cell line is an integral component of this complex. Eur J Biochem. 1985 Mar 1;147(2):281–289. doi: 10.1111/j.1432-1033.1985.tb08748.x. [DOI] [PubMed] [Google Scholar]
- Moline G., Hampel A., Enger M. D. Polyribosomal and particulate distribution of lysyl- and phenylalanyl-transfer ribonucleic acid synthetases. Biochem J. 1974 Oct;143(1):191–195. doi: 10.1042/bj1430191. [DOI] [PMC free article] [PubMed] [Google Scholar]
- Natsoulis G., Hilger F., Fink G. R. The HTS1 gene encodes both the cytoplasmic and mitochondrial histidine tRNA synthetases of S. cerevisiae. Cell. 1986 Jul 18;46(2):235–243. doi: 10.1016/0092-8674(86)90740-3. [DOI] [PubMed] [Google Scholar]
- Nielsen P., Goelz S., Trachsel H. The role of the cytoskeleton in eukaryotic protein synthesis. (A minireview). Cell Biol Int Rep. 1983 Apr;7(4):245–254. doi: 10.1016/0309-1651(83)90057-7. [DOI] [PubMed] [Google Scholar]
- Norcum M. T. Isolation and electron microscopic characterization of the high molecular mass aminoacyl-tRNA synthetase complex from murine erythroleukemia cells. J Biol Chem. 1989 Sep 5;264(25):15043–15051. [PubMed] [Google Scholar]
- Ornelles D. A., Fey E. G., Penman S. Cytochalasin releases mRNA from the cytoskeletal framework and inhibits protein synthesis. Mol Cell Biol. 1986 May;6(5):1650–1662. doi: 10.1128/mcb.6.5.1650. [DOI] [PMC free article] [PubMed] [Google Scholar]
- Pollard J. W., Galpine A. R., Clemens M. J. A novel role for aminoacyl-tRNA synthetases in the regulation of polypeptide chain initiation. Eur J Biochem. 1989 Jun 1;182(1):1–9. doi: 10.1111/j.1432-1033.1989.tb14793.x. [DOI] [PubMed] [Google Scholar]
- Rechsteiner M. Ubiquitin-mediated pathways for intracellular proteolysis. Annu Rev Cell Biol. 1987;3:1–30. doi: 10.1146/annurev.cb.03.110187.000245. [DOI] [PubMed] [Google Scholar]
- Ritter P. O., Enger M. D., Hampel A. E. Characterization of postribosomal aminoacyl-tRNA synthetases in cultured Chinese hamster ovary cells. Biochim Biophys Acta. 1979 May 24;562(3):377–385. doi: 10.1016/0005-2787(79)90102-3. [DOI] [PubMed] [Google Scholar]
- Sihag R. K., Deutscher M. P. Perturbation of the aminoacyl-tRNA synthetase complex by salts and detergents. Importance of hydrophobic interactions and possible involvement of lipids. J Biol Chem. 1983 Oct 10;258(19):11846–11850. [PubMed] [Google Scholar]
- Sivaram P., Vellekamp G., Deutscher M. P. A role for lipids in the functional and structural properties of the rat liver aminoacyl-tRNA synthetase complex. J Biol Chem. 1988 Dec 15;263(35):18891–18896. [PubMed] [Google Scholar]
- Som K., Hardesty B. Isolation and partial characterization of an aminoacyl-tRNA synthetase complex from rabbit reticulocytes. Arch Biochem Biophys. 1975 Feb;166(2):507–517. doi: 10.1016/0003-9861(75)90414-2. [DOI] [PubMed] [Google Scholar]
- Spence A. M., Sheppard P. C., Davie J. R., Matuo Y., Nishi N., McKeehan W. L., Dodd J. G., Matusik R. J. Regulation of a bifunctional mRNA results in synthesis of secreted and nuclear probasin. Proc Natl Acad Sci U S A. 1989 Oct;86(20):7843–7847. doi: 10.1073/pnas.86.20.7843. [DOI] [PMC free article] [PubMed] [Google Scholar]
- Srere P. A. Complexes of sequential metabolic enzymes. Annu Rev Biochem. 1987;56:89–124. doi: 10.1146/annurev.bi.56.070187.000513. [DOI] [PubMed] [Google Scholar]
- Van Dang C., Yang D. C. Disassembly and gross structure of particulate aminoacyl-tRNA synthetases from rat liver. Isolation and the structural relationship of synthetase complexes. J Biol Chem. 1979 Jun 25;254(12):5350–5356. [PubMed] [Google Scholar]
- Van Venrooij W. J., Moonen H., Van Loon-Klaassen L. Source of amino acids used for protein synthesis in HeLa cells. Eur J Biochem. 1974 Dec 16;50(1):297–304. doi: 10.1111/j.1432-1033.1974.tb03898.x. [DOI] [PubMed] [Google Scholar]
- Vellekamp G., Deutscher M. P. A basic NH2-terminal extension of rat liver arginyl-tRNA synthetase required for its association with high molecular weight complexes. J Biol Chem. 1987 Jul 25;262(21):9927–9930. [PubMed] [Google Scholar]
- Vellekamp G., Sihag R. K., Deutscher M. P. Comparison of the complexed and free forms of rat liver arginyl-tRNA synthetase and origin of the free form. J Biol Chem. 1985 Aug 15;260(17):9843–9847. [PubMed] [Google Scholar]
- Walker E. J., Treacy G. B., Jeffrey P. D. Molecular weights of mitochondrial and cytoplasmic aminoacyl-tRNA synthetases of beef liver and their complexes. Biochemistry. 1983 Apr 12;22(8):1934–1941. doi: 10.1021/bi00277a030. [DOI] [PubMed] [Google Scholar]
- Wallyn C. S., Vidrich A., Airhart J., Khairallah E. A. Analysis of the specific radioactivity of valine isolated from aminoacyl-transfer ribonucleic acid of rat liver. Biochem J. 1974 Jun;140(3):545–548. doi: 10.1042/bj1400545. [DOI] [PMC free article] [PubMed] [Google Scholar]
- Yang D. C., Garcia J. V., Johnson Y. D., Wahab S. Multienzyme complexes of mammalian aminoacyl-tRNA synthetases. Curr Top Cell Regul. 1985;26:325–335. doi: 10.1016/b978-0-12-152826-3.50031-0. [DOI] [PubMed] [Google Scholar]