Abstract
Isolated spinach chloroplasts have been used as a model system for studying the interaction of ozone, a component of photochemical smog, with plant membranes. Ozone bubbled into a suspension of isolated chloroplasts inhibits electron transport in both photosystems without uncoupling ATP production. Photosystem I (reduced 2,6-dichlorophenolindolphenol → NADP+) is a little more sensitive than photosystem II (H2O → 2,6-dichlophenolindolphenol). Ozone does not act as an energy transfer inhibitor, since the drop in ATP production and high energy intermediate (measured by amine-induced swelling) is nearly parallel to the decline in electron transport. A reasonable hypothesis is that ozone disrupts the normal pathway of energy flow from light-excited chlorophyll into the photoacts by a disruption of the components of the membrane but not a general disintegration of the membrane. In addition, ozone does not seem to penetrate into the grana region through the outer membrane of intact plastids, since ozone lowers the bicarbonate-supported O2 evolution but does not affect the rate of ferricyanide reduction in the same plastids after osmotic disruption. This would indicate that the effect of ozone on green plants, at low concentrations, may be due to the interaction of ozone with the first membrane it contacts and not directly with internal metabolic processes.
Full text
PDF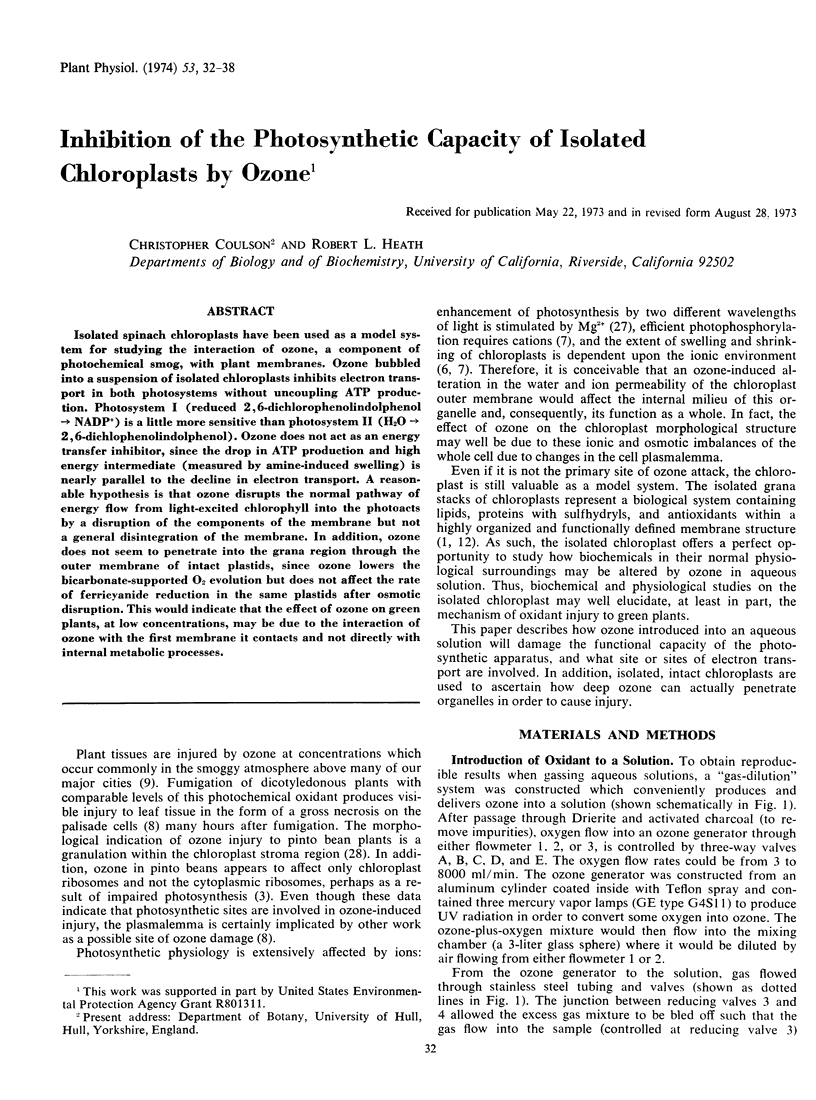
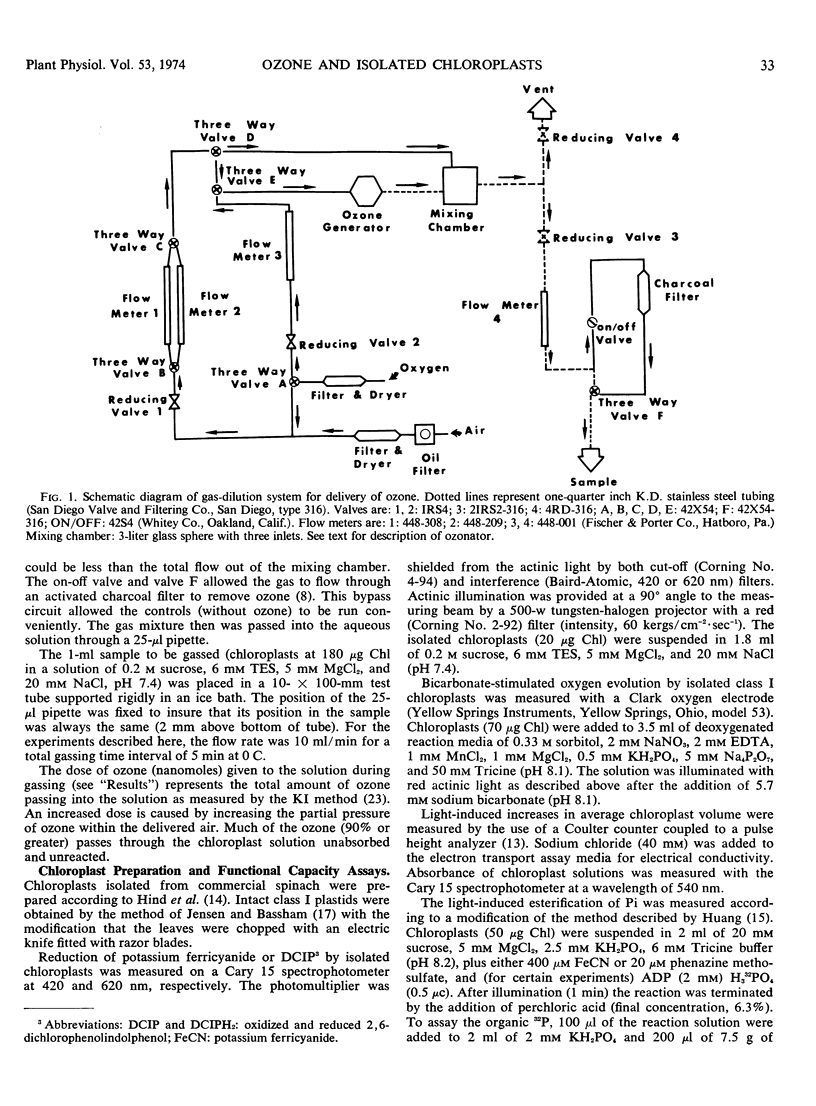
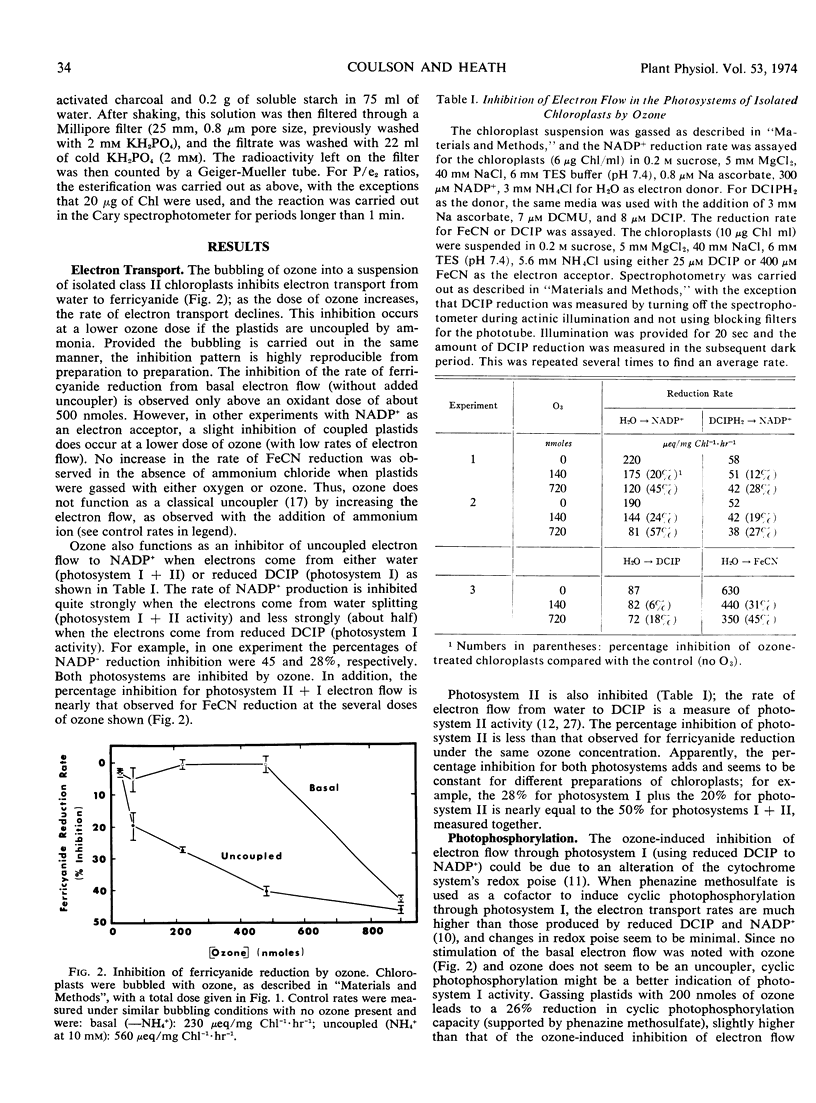
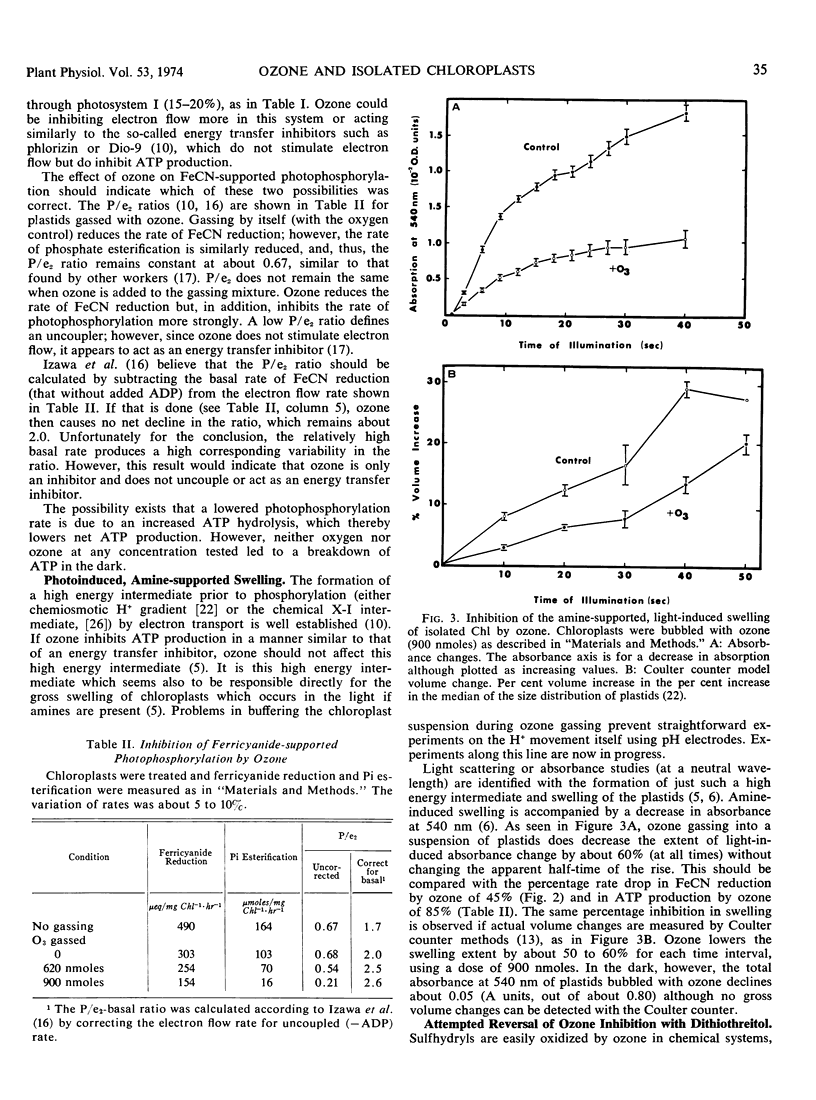
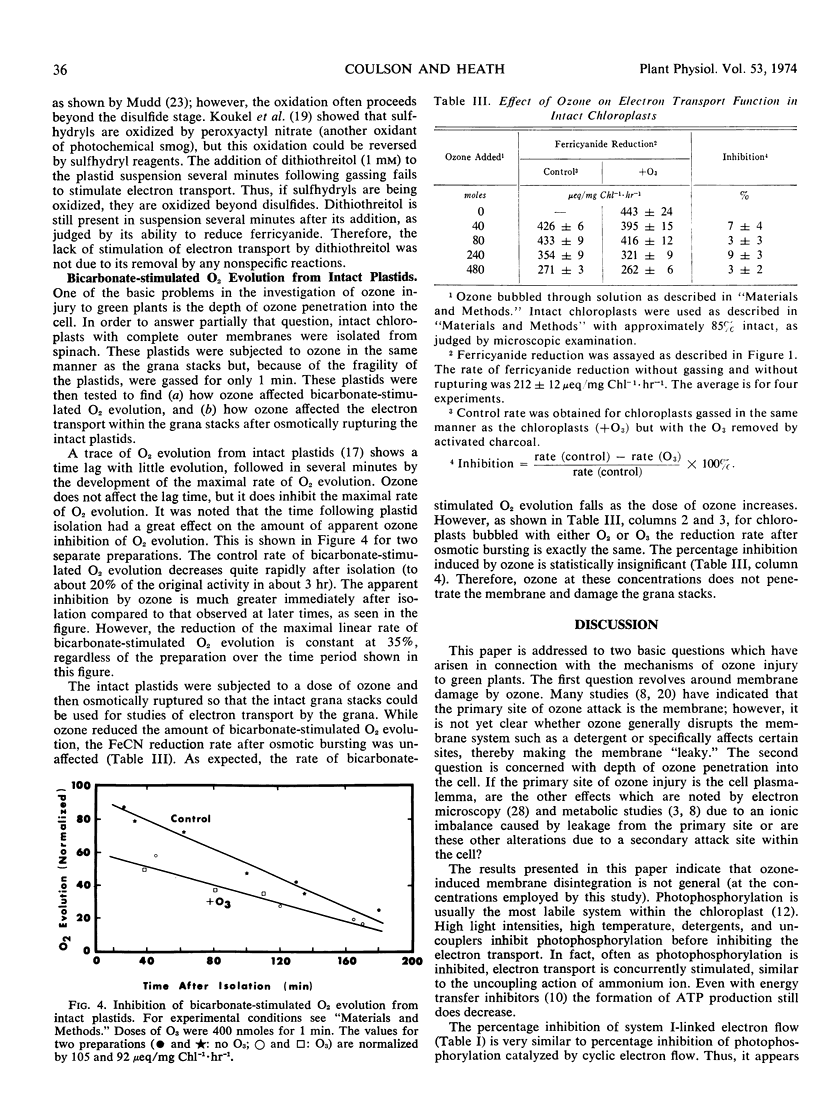
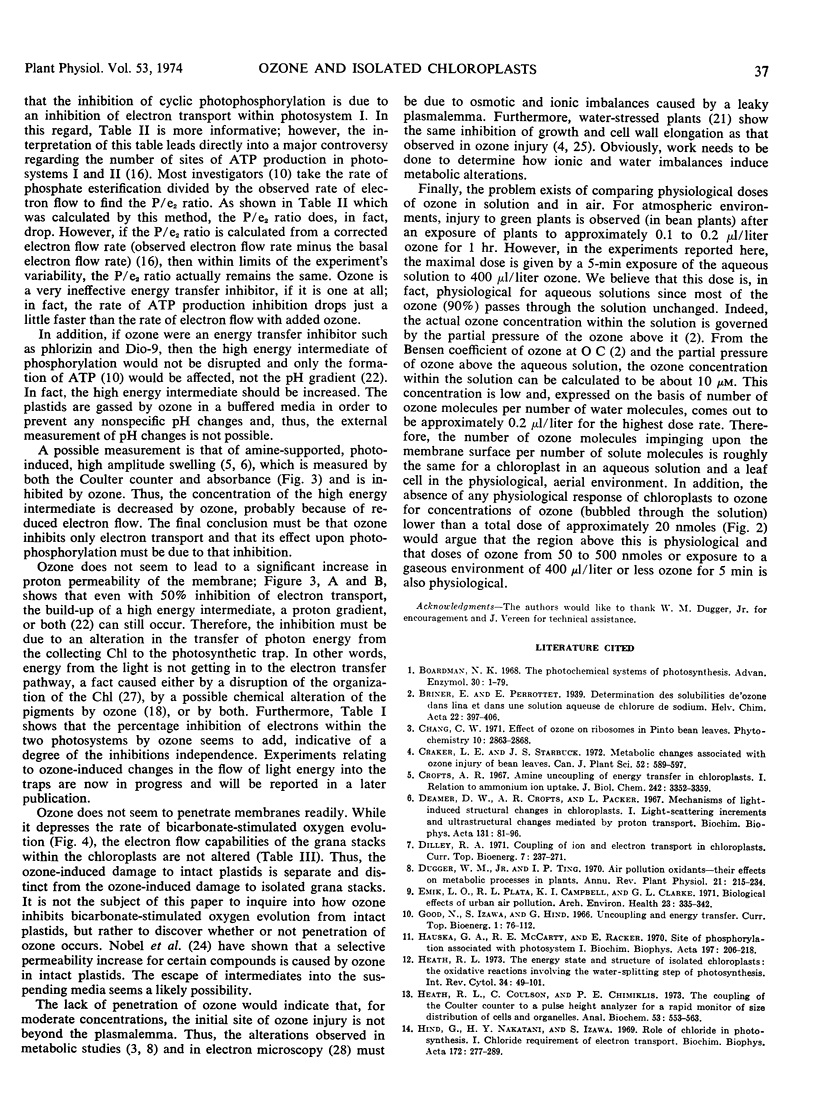
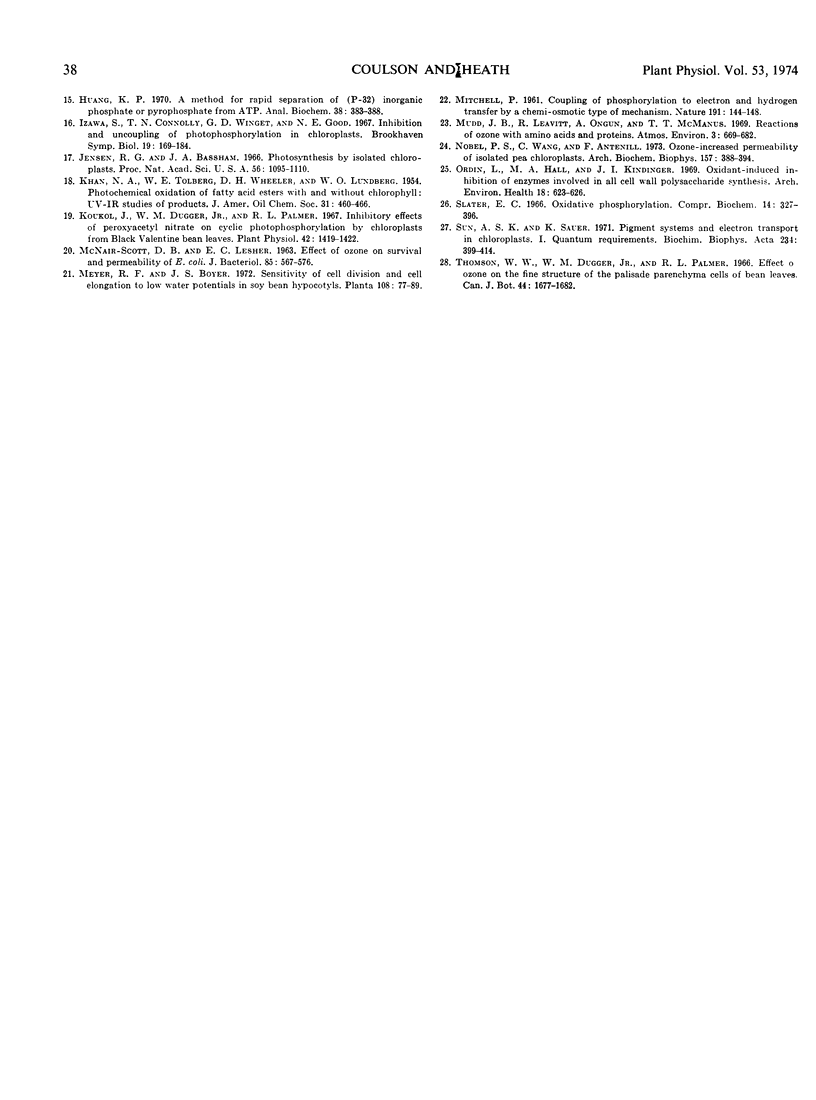
Selected References
These references are in PubMed. This may not be the complete list of references from this article.
- Crofts A. R. Amine uncoupling of energy transfer in chloroplasts. I. Relation to ammonium ion uptake. J Biol Chem. 1967 Jul 25;242(14):3352–3359. [PubMed] [Google Scholar]
- Emik L. O., Plata R. L., Campbell K. I., Clarke G. L. Biological effects of urban air pollution: Riverside summary. Arch Environ Health. 1971 Nov;23(5):335–342. doi: 10.1080/00039896.1971.10666018. [DOI] [PubMed] [Google Scholar]
- Hauska G. A., McCarty R. E., Racker E. The site of phosphorylation associated with photosystem. Biochim Biophys Acta. 1970 Mar 3;197(2):206–218. doi: 10.1016/0005-2728(70)90032-0. [DOI] [PubMed] [Google Scholar]
- Heath R. L., Coulson C. L., Chimiklis P. The coupling of the Coulter Counter to a pulse height analyzer for a rapid monitor of size distributions of cells and organelles. Anal Biochem. 1973 Jun;53(2):555–563. doi: 10.1016/0003-2697(73)90106-1. [DOI] [PubMed] [Google Scholar]
- Hind G., Nakatani H. Y., Izawa S. The role of Cl- in photosynthesis. I. The Cl- requirement of electron transport. Biochim Biophys Acta. 1969 Feb 25;172(2):277–289. doi: 10.1016/0005-2728(69)90070-x. [DOI] [PubMed] [Google Scholar]
- Huang K. P. A method for rapid separation of [32P]-inorganic phosphate or pyrophosphate from ATP. Anal Biochem. 1970 Dec;38(2):383–388. doi: 10.1016/0003-2697(70)90461-6. [DOI] [PubMed] [Google Scholar]
- Izawa S., Connolly T. N., Winget G. D., Good N. E. Inhibition and uncoupling of photophosphorylation in chloroplasts. Brookhaven Symp Biol. 1966;19:169–187. [PubMed] [Google Scholar]
- Jensen R. G., Bassham J. A. Photosynthesis by isolated chloroplasts. Proc Natl Acad Sci U S A. 1966 Oct;56(4):1095–1101. doi: 10.1073/pnas.56.4.1095. [DOI] [PMC free article] [PubMed] [Google Scholar]
- Koukol J., Dugger W. M., Jr, Palmer R. L. Inhibitory effect of peroxyacetyl nitrate on cyclic photophosphorylation by chloroplasts from black valentine bean leaves. Plant Physiol. 1967 Oct;42(10):1419–1422. doi: 10.1104/pp.42.10.1419. [DOI] [PMC free article] [PubMed] [Google Scholar]
- MITCHELL P. Coupling of phosphorylation to electron and hydrogen transfer by a chemi-osmotic type of mechanism. Nature. 1961 Jul 8;191:144–148. doi: 10.1038/191144a0. [DOI] [PubMed] [Google Scholar]
- Mudd J. B., Leavitt R., Ongun A., McManus T. T. Reaction of ozone with amino acids and proteins. Atmos Environ. 1969 Nov;3(6):669–682. doi: 10.1016/0004-6981(69)90024-9. [DOI] [PubMed] [Google Scholar]
- Nobel P. S., Wang C. T. Ozone increases the permeability of isolated pea chloroplasts. Arch Biochem Biophys. 1973 Aug;157(2):388–394. doi: 10.1016/0003-9861(73)90654-1. [DOI] [PubMed] [Google Scholar]
- Ordin L., Hall M. A., Kindinger J. I. Oxidant-induced inhibition of enzymes involved in cell wall polysaccharide synthesis. Arch Environ Health. 1969 Apr;18(4):623–626. doi: 10.1080/00039896.1969.10665462. [DOI] [PubMed] [Google Scholar]
- SCOTT D. B., LESHER E. C. EFFECT OF OZONE ON SURVIVAL AND PERMEABILITY OF ESCHERICHIA COLI. J Bacteriol. 1963 Mar;85:567–576. doi: 10.1002/path.1700850242. [DOI] [PMC free article] [PubMed] [Google Scholar]
- Sun A. S., Sauer K. Pigment systems and electron transport in chloroplasts. I. Quantum requirements for the two light reactions in spinach chloroplasts. Biochim Biophys Acta. 1971 Jun 15;234(3):399–414. doi: 10.1016/0005-2728(71)90207-6. [DOI] [PubMed] [Google Scholar]