Abstract
We have carried out free energy perturbation calculations on DNA double-stranded hexanucleotides. The sequence d(CGCGCG)2 has been "mutated" into d(CGTGCG).d(CGCACG) with the oligonucleotide in the A, B, and Z structural forms, both in vacuo and in aqueous solution. In addition, model free energy calculations have been carried out in which the electrostatic charges of the H-bonding groups of the bases in the major and minor grooves of the DNA are reduced to zero as a way of assessing the relative solvation effects of these groups in the different structural forms of DNA. Finally, energy component analyses have been carried out to assess the relative roles of different intranucleotide interactions on the B----Z equilibrium as a function of base sequence. In vacuo, the free energy for changing a G.C to an A.T base pair is largest in the Z conformation; in the A and B conformations, the free energy cost is approximately 2 kcal/mol lower (1 cal = 4.184 J). The results are similar when the simulations are run in explicit solvent: the change costs 3 kcal/mol more in the Z conformation than in the B form. These results are consistent with experimental data, where it is clear that A.T sequences are significantly more "Z-phobic" than G.C sequences. The calculations indicate that both intranucleotide and solvation interactions contribute to this Z-phobicity.
Full text
PDF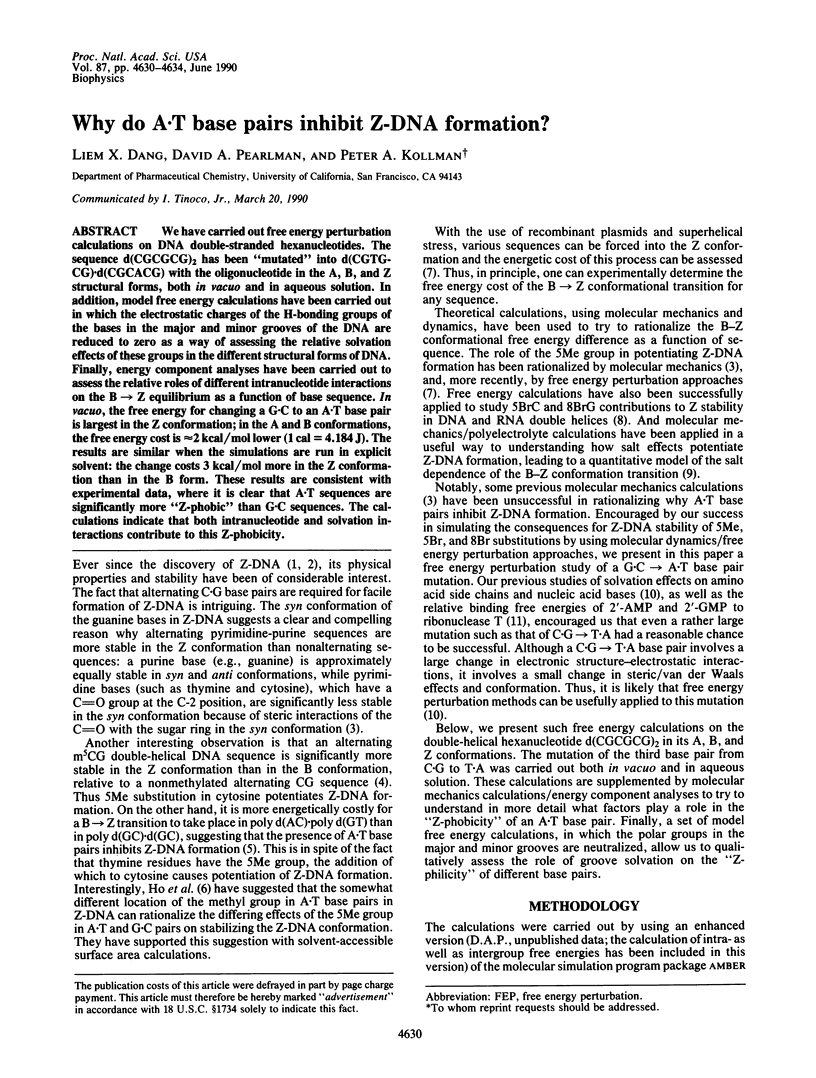
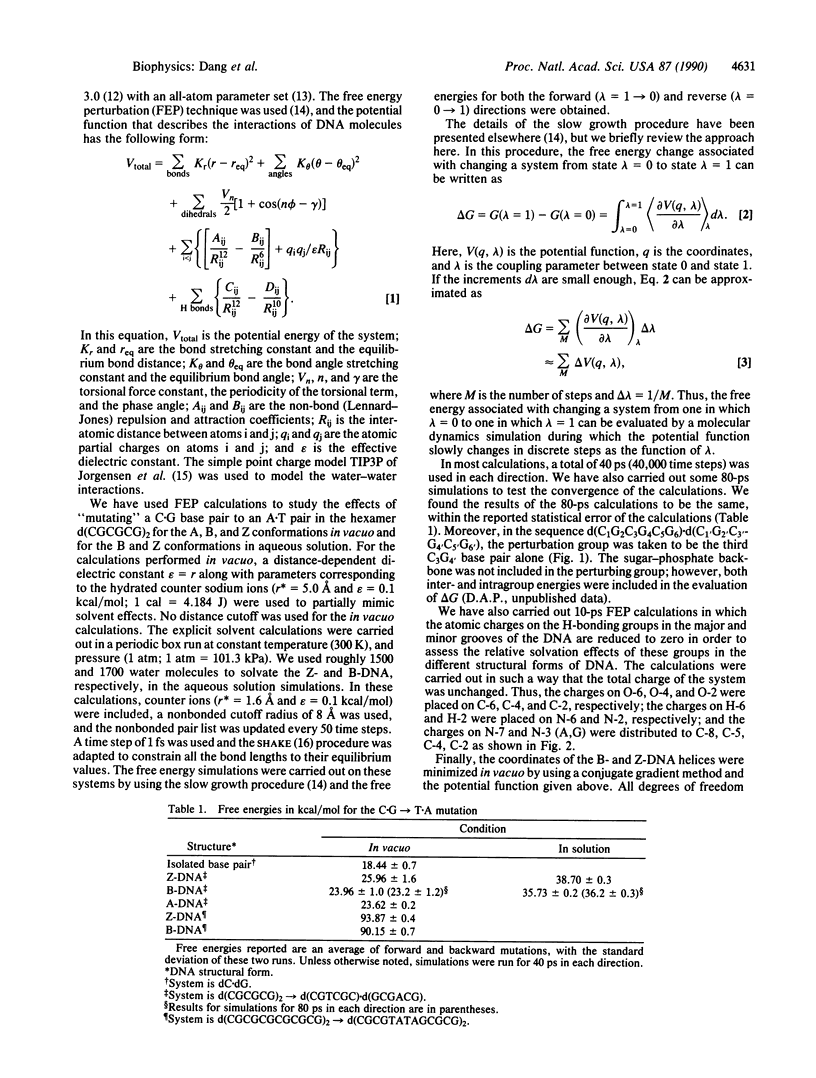
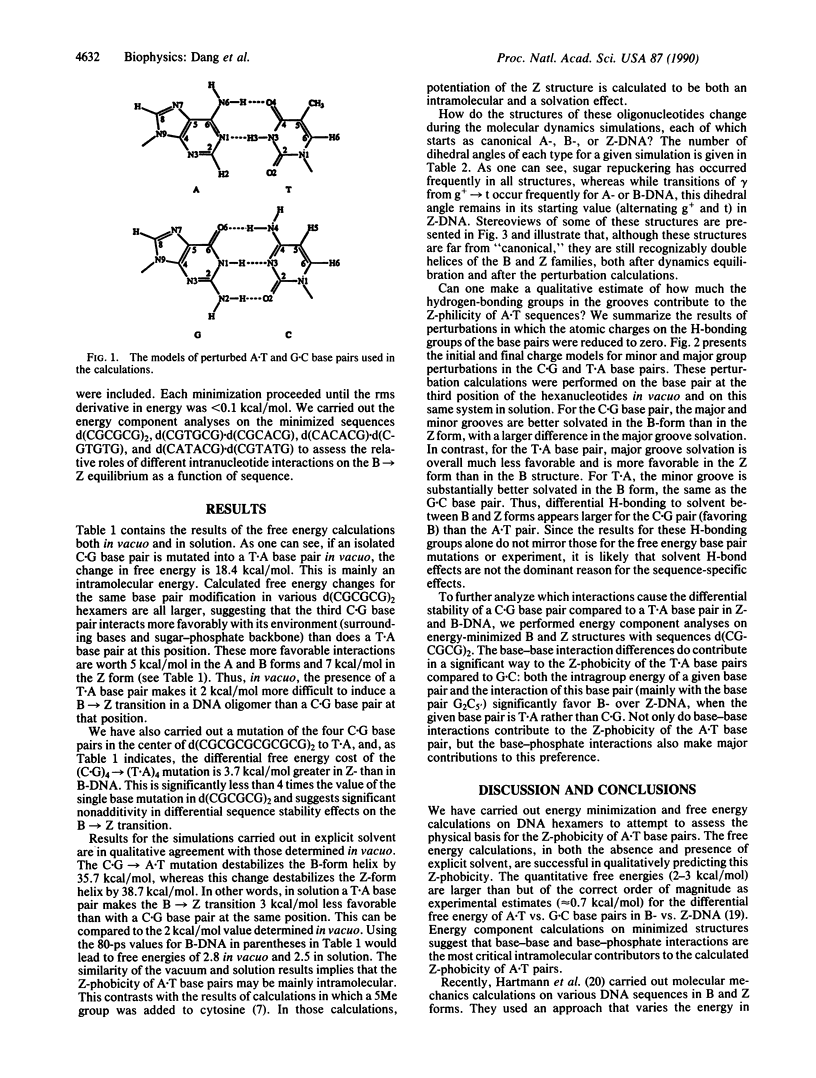
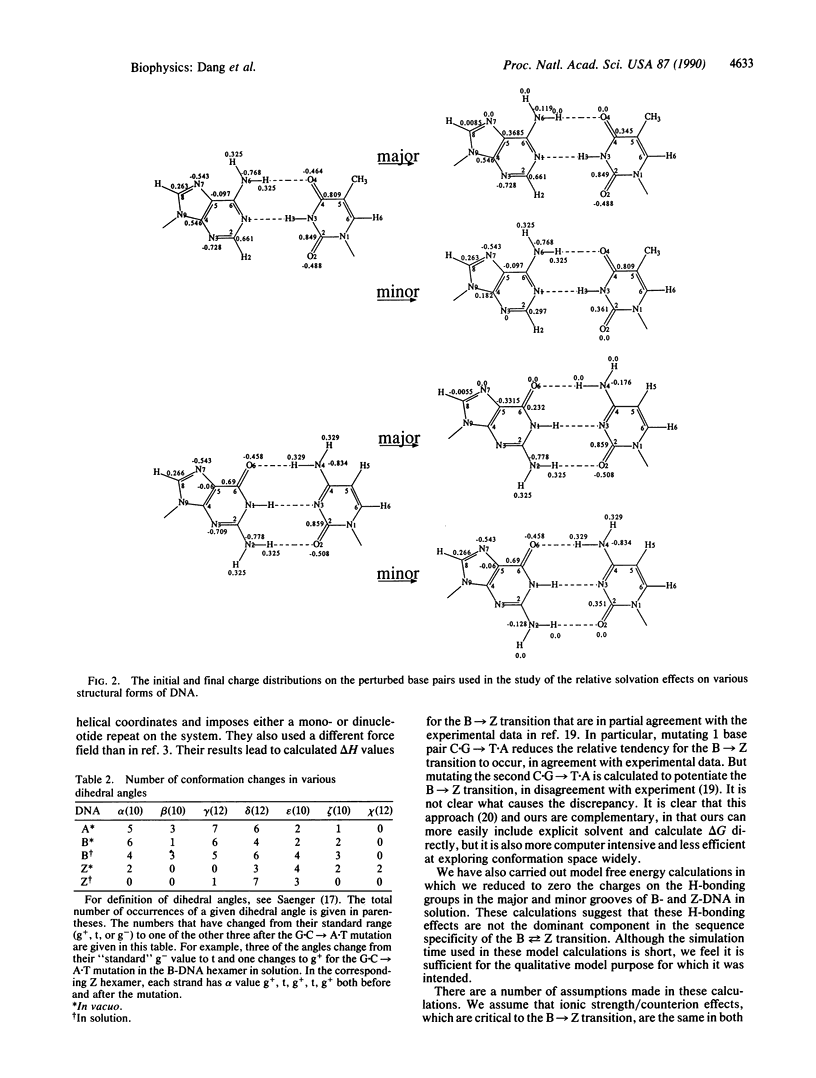
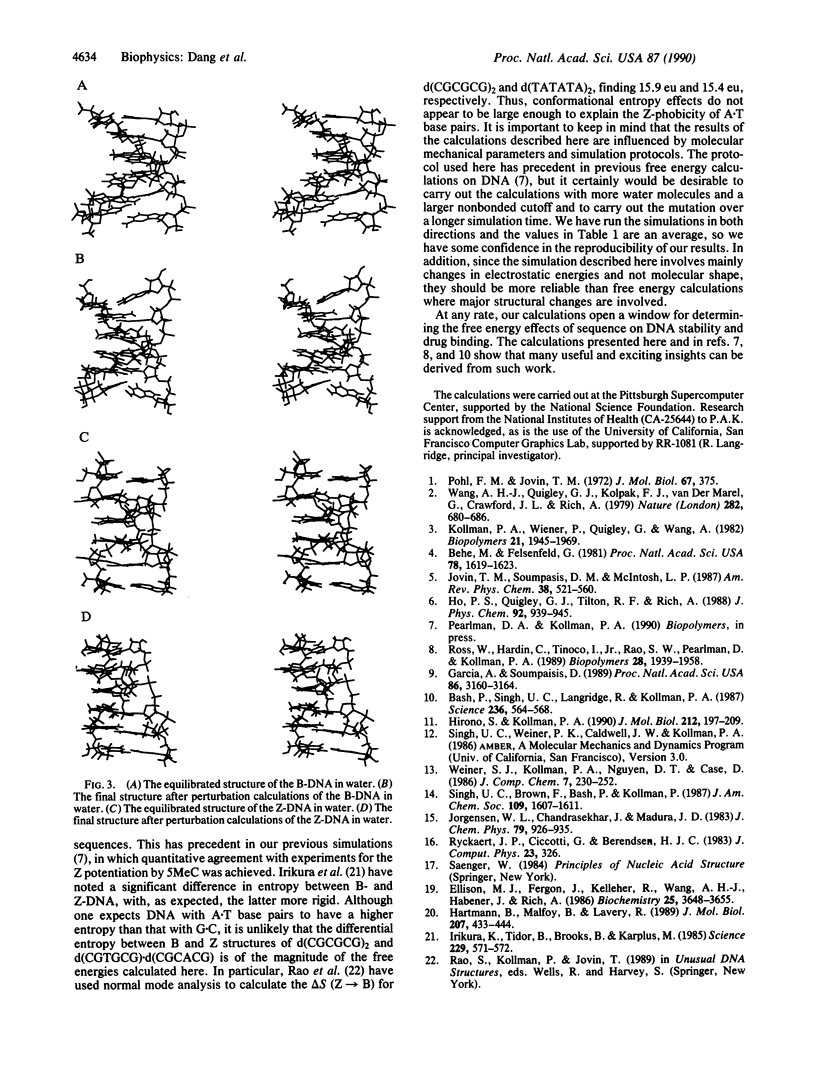
Selected References
These references are in PubMed. This may not be the complete list of references from this article.
- Bash P. A., Singh U. C., Langridge R., Kollman P. A. Free energy calculations by computer simulation. Science. 1987 May 1;236(4801):564–568. doi: 10.1126/science.3576184. [DOI] [PubMed] [Google Scholar]
- Behe M., Felsenfeld G. Effects of methylation on a synthetic polynucleotide: the B--Z transition in poly(dG-m5dC).poly(dG-m5dC). Proc Natl Acad Sci U S A. 1981 Mar;78(3):1619–1623. doi: 10.1073/pnas.78.3.1619. [DOI] [PMC free article] [PubMed] [Google Scholar]
- Ellison M. J., Feigon J., Kelleher R. J., 3rd, Wang A. H., Habener J. F., Rich A. An assessment of the Z-DNA forming potential of alternating dA-dT stretches in supercoiled plasmids. Biochemistry. 1986 Jun 17;25(12):3648–3655. doi: 10.1021/bi00360a026. [DOI] [PubMed] [Google Scholar]
- García A. E., Soumpasis D. M. Harmonic vibrations and thermodynamic stability of a DNA oligomer in monovalent salt solution. Proc Natl Acad Sci U S A. 1989 May;86(9):3160–3164. doi: 10.1073/pnas.86.9.3160. [DOI] [PMC free article] [PubMed] [Google Scholar]
- Hartmann B., Malfoy B., Lavery R. Theoretical prediction of base sequence effects in DNA. Experimental reactivity of Z-DNA and B-Z transition enthalpies. J Mol Biol. 1989 May 20;207(2):433–444. doi: 10.1016/0022-2836(89)90265-9. [DOI] [PubMed] [Google Scholar]
- Hirono S., Kollman P. A. Calculation of the relative binding free energy of 2'GMP and 2'AMP to ribonuclease T1 using molecular dynamics/free energy perturbation approaches. J Mol Biol. 1990 Mar 5;212(1):197–209. doi: 10.1016/0022-2836(90)90315-D. [DOI] [PubMed] [Google Scholar]
- Irikura K. K., Tidor B., Brooks B. R., Karplus M. Transition from B to Z DNA: contribution of internal fluctuations to the configurational entropy difference. Science. 1985 Aug 9;229(4713):571–572. doi: 10.1126/science.3839596. [DOI] [PubMed] [Google Scholar]
- Kollman P., Weiner P., Quigley G., Wang A. Molecular-mechanical studies of Z-DNA: a comparison of the structural and energetic properties of Z- and B-DNA. Biopolymers. 1982 Oct;21(10):1945–1969. doi: 10.1002/bip.360211003. [DOI] [PubMed] [Google Scholar]
- Pohl F. M., Jovin T. M. Salt-induced co-operative conformational change of a synthetic DNA: equilibrium and kinetic studies with poly (dG-dC). J Mol Biol. 1972 Jun 28;67(3):375–396. doi: 10.1016/0022-2836(72)90457-3. [DOI] [PubMed] [Google Scholar]
- Ross W. S., Hardin C. C., Tinoco I., Jr, Rao S. N., Pearlman D. A., Kollman P. A. Effects of nucleotide bromination on the stabilities of Z-RNA and Z-DNA: a molecular mechanics/thermodynamic perturbation study. Biopolymers. 1989 Nov;28(11):1939–1957. doi: 10.1002/bip.360281111. [DOI] [PubMed] [Google Scholar]
- Wang A. H., Quigley G. J., Kolpak F. J., Crawford J. L., van Boom J. H., van der Marel G., Rich A. Molecular structure of a left-handed double helical DNA fragment at atomic resolution. Nature. 1979 Dec 13;282(5740):680–686. doi: 10.1038/282680a0. [DOI] [PubMed] [Google Scholar]