Abstract
Cells from patients with Cockayne syndrome (CS) are hypersensitive to UV-irradiation but have an apparently normal ability to remove pyrimidine dimers from the genome overall. We have measured the repair of pyrimidine dimers in defined DNA sequences in three normal and two CS cell strains. When compared to a nontranscribed locus, transcriptionally active genes were preferentially repaired in all three normal cell strains. There was no significant variation in levels of repair between various normal individuals or between two constitutively expressed genes, indicating that preferential repair may be a consistent feature of constitutively expressed genes in human cells. Neither CS strain, from independent complementation groups, was able to repair transcriptionally active DNA with a similar rate and to the same extent as normal cells, indicating that the genetic defect in CS lies in the pathway for repair of transcriptionally active DNA. These results have implications for understanding the pleiotropic clinical effects associated with disorders having defects in the repair of DNA damage. In particular, neurodegeneration appears to be associated with the loss of preferential repair of active genes and is not simply correlated with reduced levels of overall repair.
Full text
PDF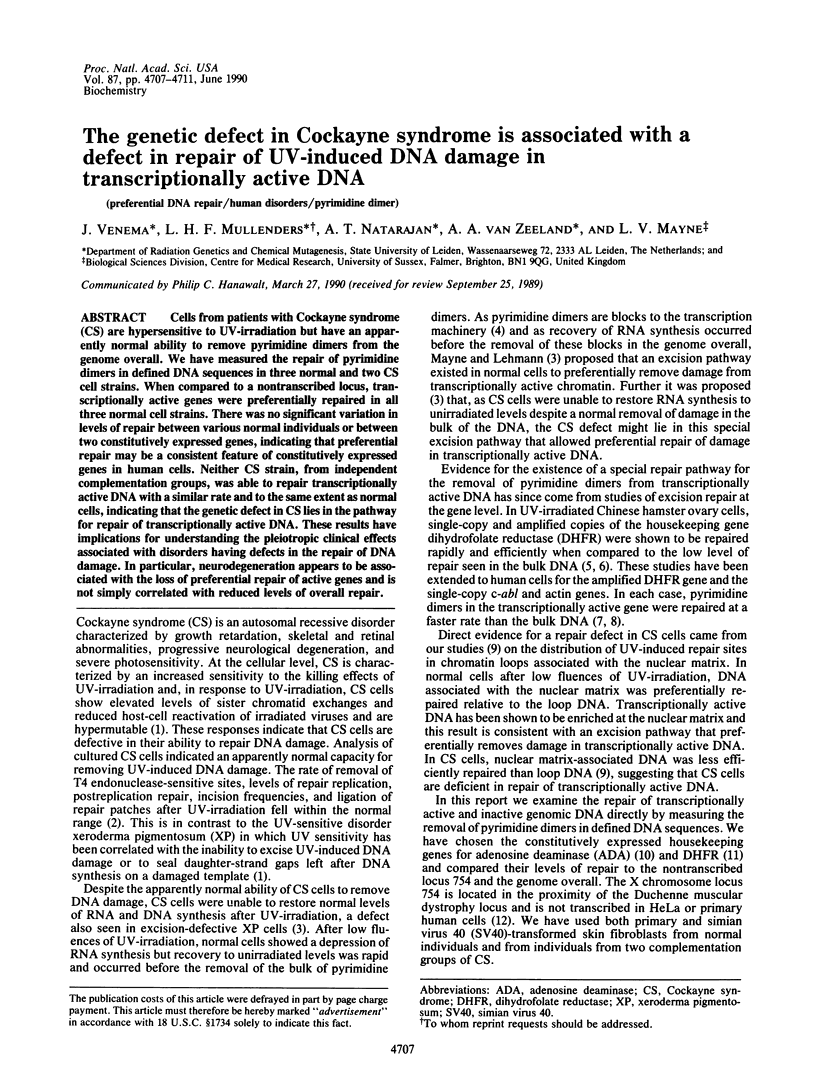
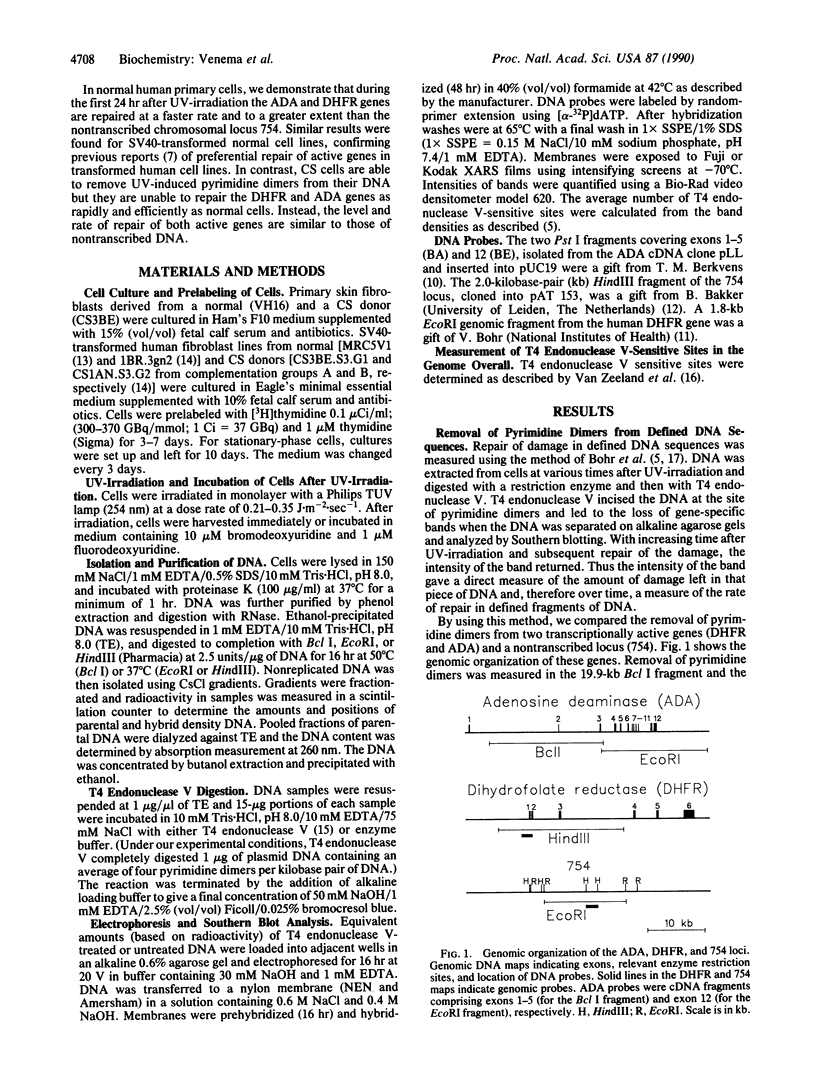
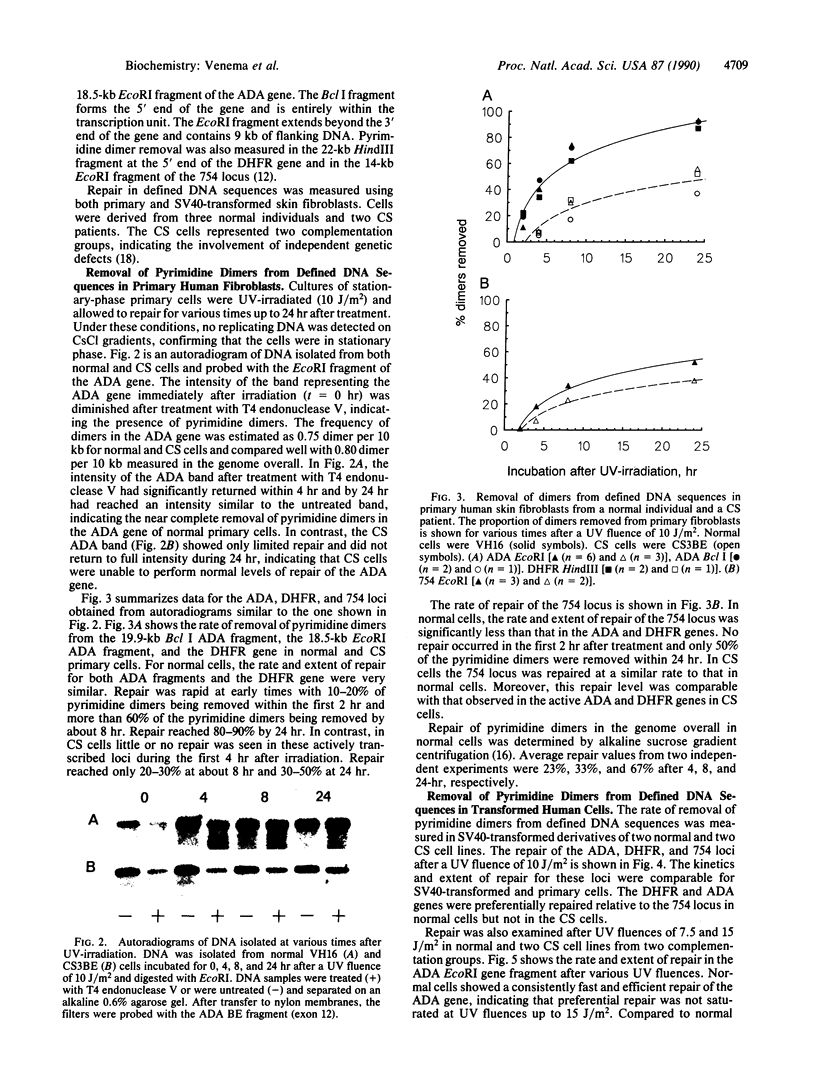
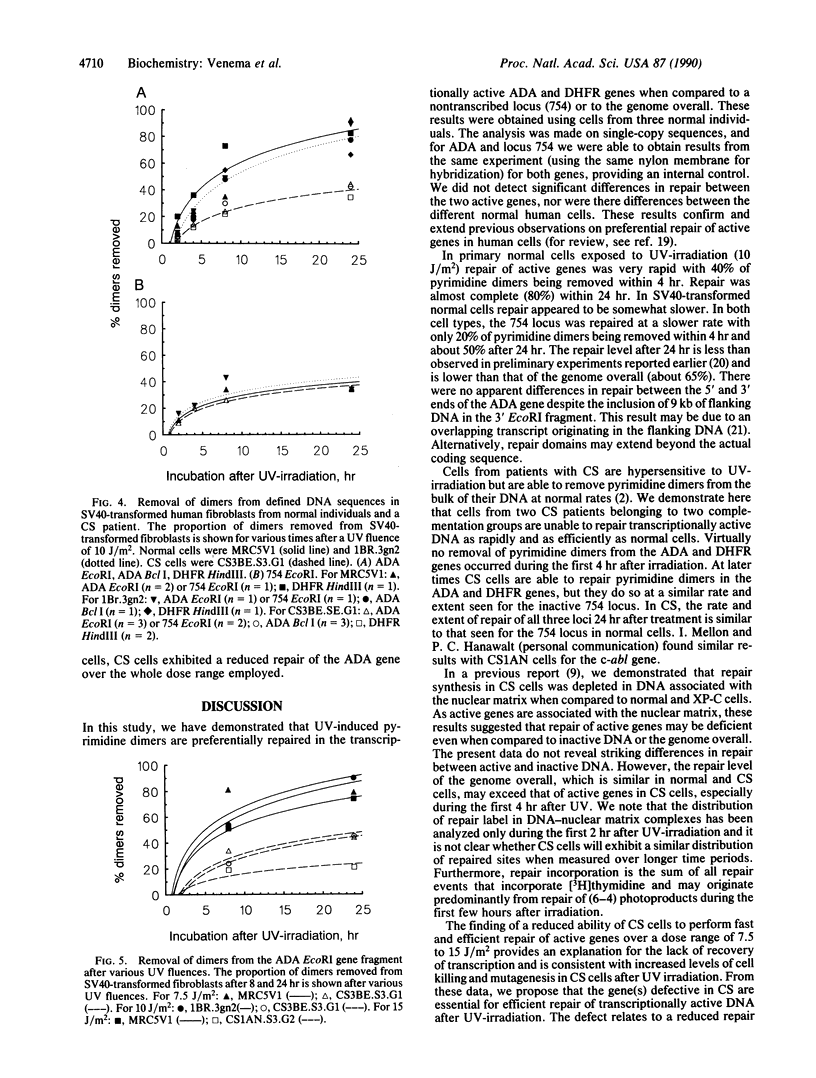
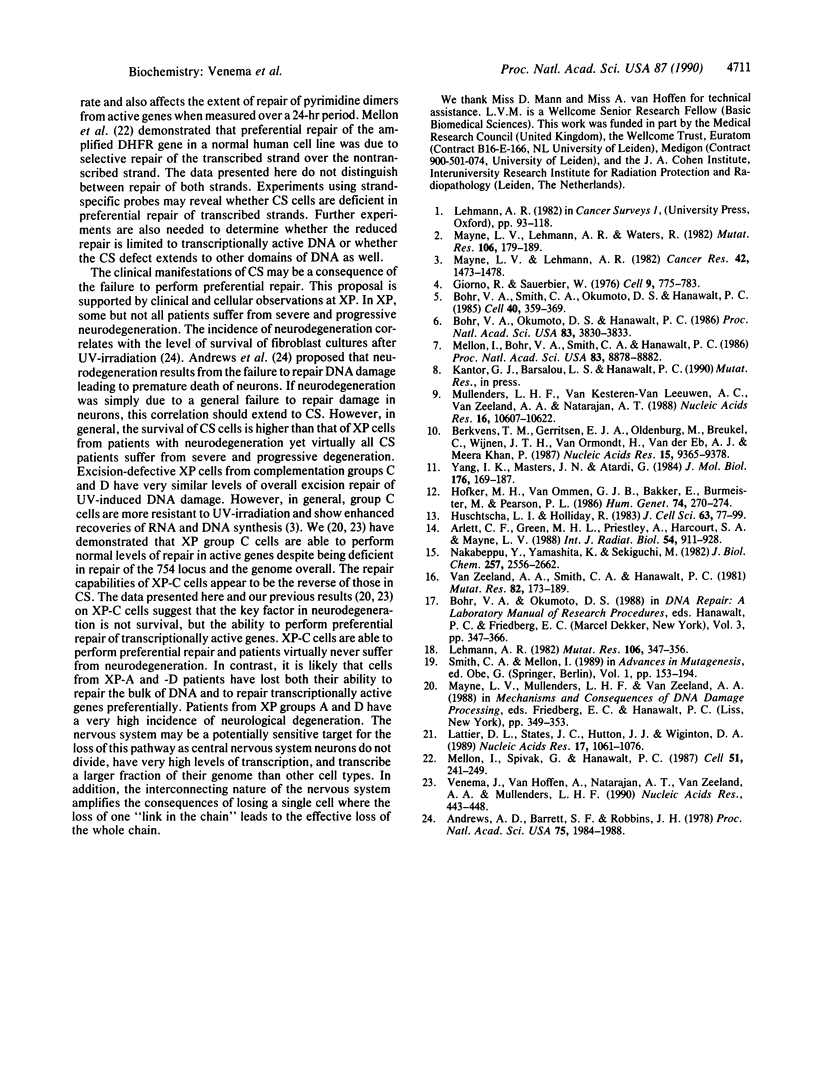
Images in this article
Selected References
These references are in PubMed. This may not be the complete list of references from this article.
- Andrews A. D., Barrett S. F., Robbins J. H. Xeroderma pigmentosum neurological abnormalities correlate with colony-forming ability after ultraviolet radiation. Proc Natl Acad Sci U S A. 1978 Apr;75(4):1984–1988. doi: 10.1073/pnas.75.4.1984. [DOI] [PMC free article] [PubMed] [Google Scholar]
- Arlett C. F., Green M. H., Priestley A., Harcourt S. A., Mayne L. V. Comparative human cellular radiosensitivity: I. The effect of SV40 transformation and immortalisation on the gamma-irradiation survival of skin derived fibroblasts from normal individuals and from ataxia-telangiectasia patients and heterozygotes. Int J Radiat Biol. 1988 Dec;54(6):911–928. doi: 10.1080/09553008814552321. [DOI] [PubMed] [Google Scholar]
- Berkvens T. M., Gerritsen E. J., Oldenburg M., Breukel C., Wijnen J. T., van Ormondt H., Vossen J. M., van der Eb A. J., Meera Khan P. Severe combined immune deficiency due to a homozygous 3.2-kb deletion spanning the promoter and first exon of the adenosine deaminase gene. Nucleic Acids Res. 1987 Nov 25;15(22):9365–9378. doi: 10.1093/nar/15.22.9365. [DOI] [PMC free article] [PubMed] [Google Scholar]
- Bohr V. A., Okumoto D. S., Hanawalt P. C. Survival of UV-irradiated mammalian cells correlates with efficient DNA repair in an essential gene. Proc Natl Acad Sci U S A. 1986 Jun;83(11):3830–3833. doi: 10.1073/pnas.83.11.3830. [DOI] [PMC free article] [PubMed] [Google Scholar]
- Bohr V. A., Smith C. A., Okumoto D. S., Hanawalt P. C. DNA repair in an active gene: removal of pyrimidine dimers from the DHFR gene of CHO cells is much more efficient than in the genome overall. Cell. 1985 Feb;40(2):359–369. doi: 10.1016/0092-8674(85)90150-3. [DOI] [PubMed] [Google Scholar]
- Giorno R., Sauerbier W. A radiological analysis of the transcription units for heterogeneous nuclear RNA in cultured murine cells. Cell. 1976 Dec;9(4 Pt 2):775–783. doi: 10.1016/0092-8674(76)90140-9. [DOI] [PubMed] [Google Scholar]
- Hofker M. H., van Ommen G. J., Bakker E., Burmeister M., Pearson P. L. Development of additional RFLP probes near the locus for Duchenne muscular dystrophy by cosmid cloning of the DXS84 (754) locus. Hum Genet. 1986 Nov;74(3):270–274. doi: 10.1007/BF00282547. [DOI] [PubMed] [Google Scholar]
- Huschtscha L. I., Holliday R. Limited and unlimited growth of SV40-transformed cells from human diploid MRC-5 fibroblasts. J Cell Sci. 1983 Sep;63:77–99. doi: 10.1242/jcs.63.1.77. [DOI] [PubMed] [Google Scholar]
- Lattier D. L., States J. C., Hutton J. J., Wiginton D. A. Cell type-specific transcriptional regulation of the human adenosine deaminase gene. Nucleic Acids Res. 1989 Feb 11;17(3):1061–1076. doi: 10.1093/nar/17.3.1061. [DOI] [PMC free article] [PubMed] [Google Scholar]
- Lehmann A. R. Three complementation groups in Cockayne syndrome. Mutat Res. 1982 Dec;106(2):347–356. doi: 10.1016/0027-5107(82)90115-4. [DOI] [PubMed] [Google Scholar]
- Mayne L. V., Lehmann A. R. Failure of RNA synthesis to recover after UV irradiation: an early defect in cells from individuals with Cockayne's syndrome and xeroderma pigmentosum. Cancer Res. 1982 Apr;42(4):1473–1478. [PubMed] [Google Scholar]
- Mayne L. V., Lehmann A. R., Waters R. Excision repair in Cockayne syndrome. Mutat Res. 1982 Nov;106(1):179–189. doi: 10.1016/0027-5107(82)90200-7. [DOI] [PubMed] [Google Scholar]
- Mellon I., Bohr V. A., Smith C. A., Hanawalt P. C. Preferential DNA repair of an active gene in human cells. Proc Natl Acad Sci U S A. 1986 Dec;83(23):8878–8882. doi: 10.1073/pnas.83.23.8878. [DOI] [PMC free article] [PubMed] [Google Scholar]
- Mellon I., Spivak G., Hanawalt P. C. Selective removal of transcription-blocking DNA damage from the transcribed strand of the mammalian DHFR gene. Cell. 1987 Oct 23;51(2):241–249. doi: 10.1016/0092-8674(87)90151-6. [DOI] [PubMed] [Google Scholar]
- Mullenders L. H., van Kesteren van Leeuwen A. C., van Zeeland A. A., Natarajan A. T. Nuclear matrix associated DNA is preferentially repaired in normal human fibroblasts, exposed to a low dose of ultraviolet light but not in Cockayne's syndrome fibroblasts. Nucleic Acids Res. 1988 Nov 25;16(22):10607–10622. doi: 10.1093/nar/16.22.10607. [DOI] [PMC free article] [PubMed] [Google Scholar]
- Nakabeppu Y., Yamashita K., Sekiguchi M. Purification and characterization of normal and mutant forms of T4 endonuclease V. J Biol Chem. 1982 Mar 10;257(5):2556–2562. [PubMed] [Google Scholar]
- Venema J., van Hoffen A., Natarajan A. T., van Zeeland A. A., Mullenders L. H. The residual repair capacity of xeroderma pigmentosum complementation group C fibroblasts is highly specific for transcriptionally active DNA. Nucleic Acids Res. 1990 Feb 11;18(3):443–448. doi: 10.1093/nar/18.3.443. [DOI] [PMC free article] [PubMed] [Google Scholar]
- Yang J. K., Masters J. N., Attardi G. Human dihydrofolate reductase gene organization. Extensive conservation of the G + C-rich 5' non-coding sequence and strong intron size divergence from homologous mammalian genes. J Mol Biol. 1984 Jun 25;176(2):169–187. doi: 10.1016/0022-2836(84)90419-4. [DOI] [PubMed] [Google Scholar]
- van Zeeland A. A., Smith C. A., Hanawalt P. C. Sensitive determination of pyrimidine dimers in DNA of UV-irradiated mammalian cells. Introduction of T4 endonuclease V into frozen and thawed cells. Mutat Res. 1981 Jun;82(1):173–189. doi: 10.1016/0027-5107(81)90148-2. [DOI] [PubMed] [Google Scholar]