Abstract
Cysteine transport by tobacco cells (Nicotiana tabacum L. var. Xanthi) cultured on liquid B-5 medium was examined.
Transport was linear with time or amount of tissue and had a pH optimum of 4.5. Cysteine transport over a wide concentration range was biphasic. The isotherm, for descriptive convenience, was divided into two segments both of which obeyed Michaelis-Menten kinetics. The Km for high affinity transport was in the range 1.7 × 10−5m(±0.17) while the Km for low affinity transport was in the range 3.5 × 10−4m(±0.13). Maximum velocities were 3 to 6 nmoles/g fresh weight/minute and 13 to 16 nmoles/g fresh weight/minute, respectively.
Azide and 2,4-dinitrophenol caused more than 90% inhibition of net transport by either system. N,N′-Dicyclohexylcarbodiimide was not inhibitory while the inhibition by carbonylcyanide m-chlorophenylhydrazone was dependent on the cysteine concentration. Only those compounds that were inhibitory to transport caused significant efflux of labeled material from preloaded cells.
Tobacco cells that had been preincubated in iodoacetamide or N-ethylmaleimide did not transport cysteine while similar treatments with dithiothreitol were only slightly inhibitory or had no effect on transport.
Transport by either system was, to some extent, inhibited by all other tested amino acids and analogs. Alanine, methionine, and S-methyl cysteine were most effective in inhibiting cysteine transport. Both alanine and methionine were competitive inhibitors of cysteine transport by either system with inhibition constants that were similar to the Km for the particular system.
Full text
PDF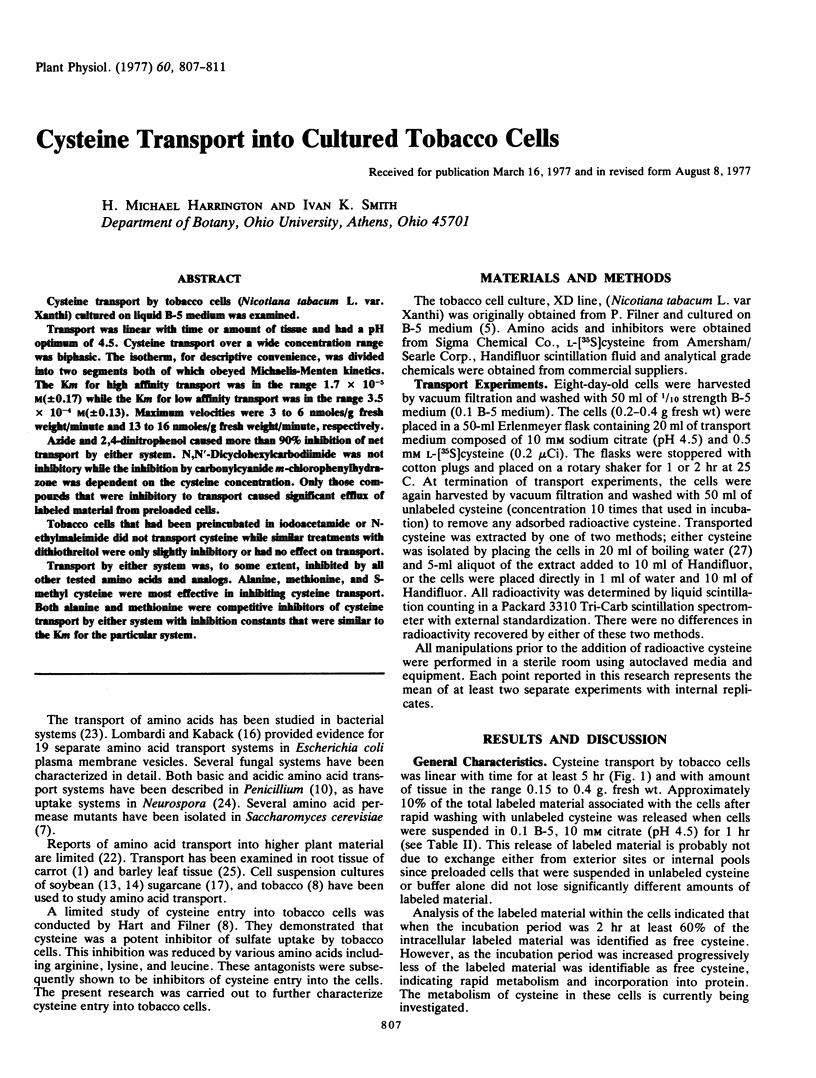
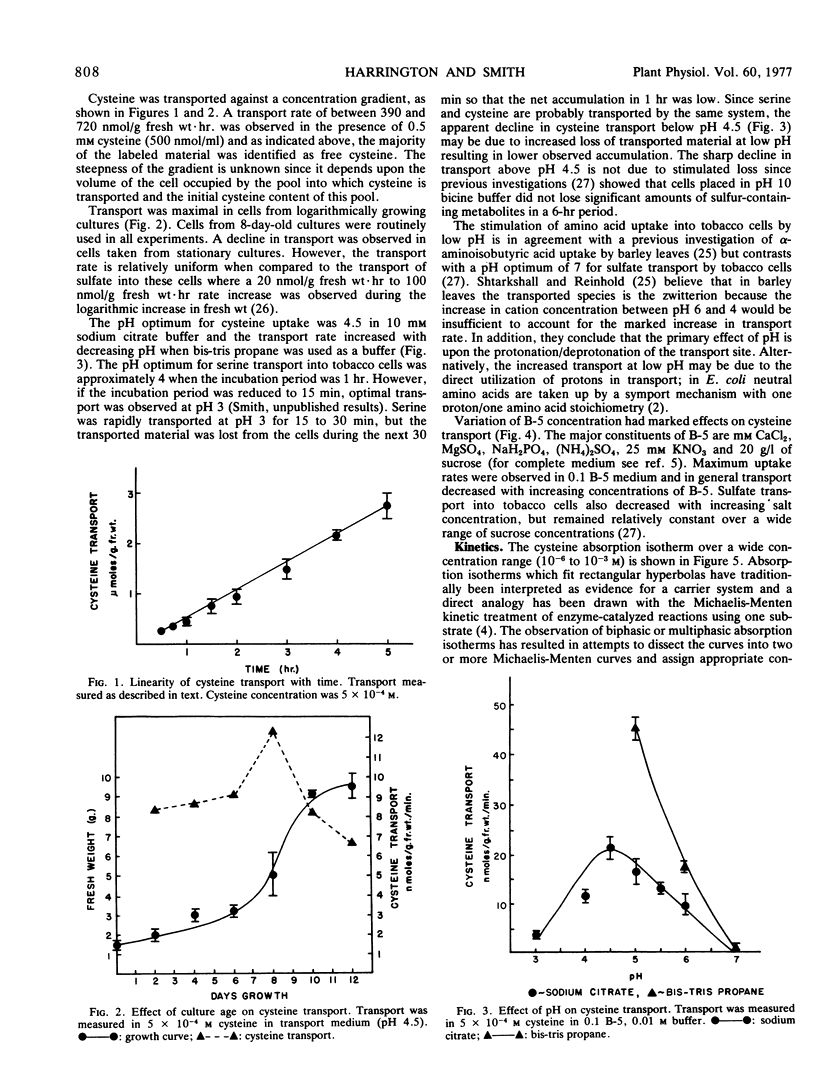
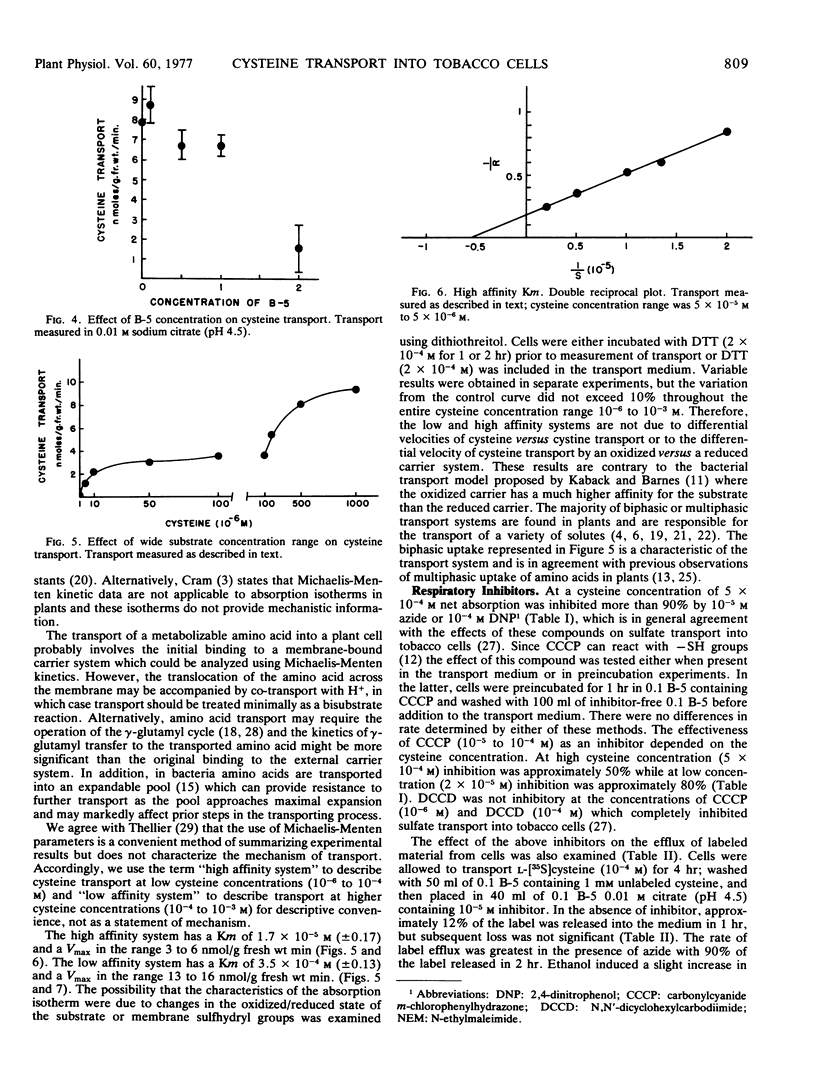
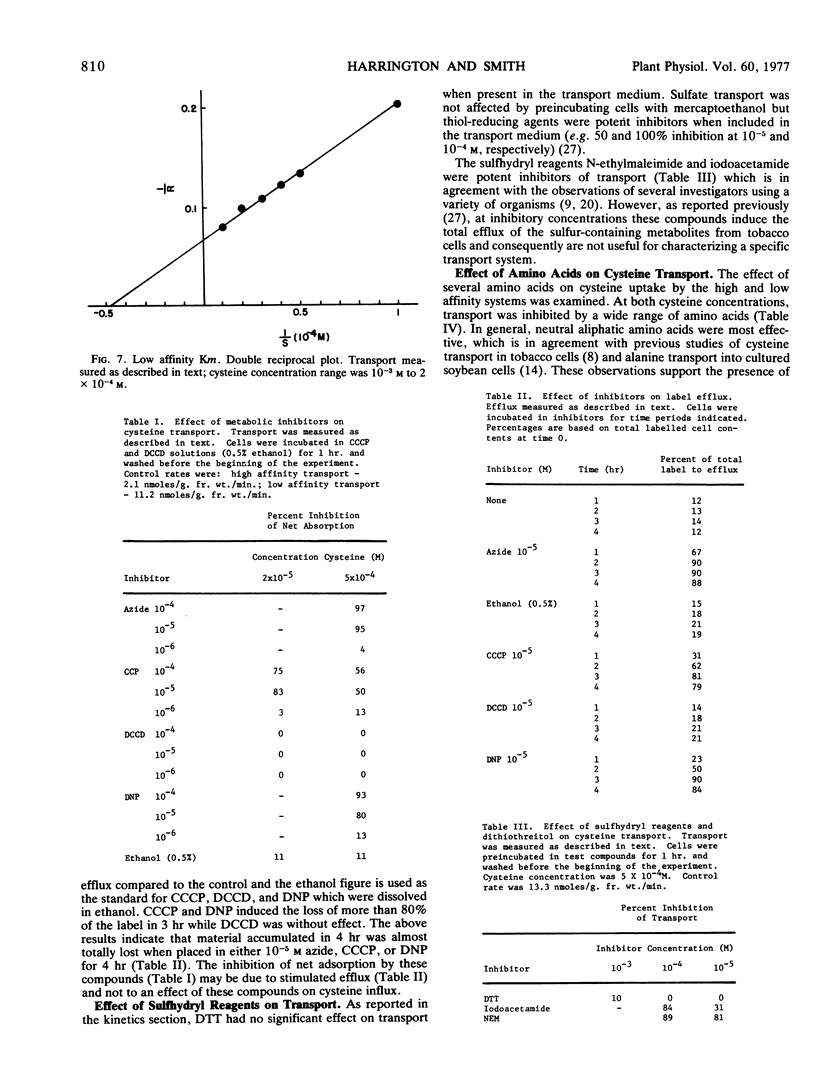
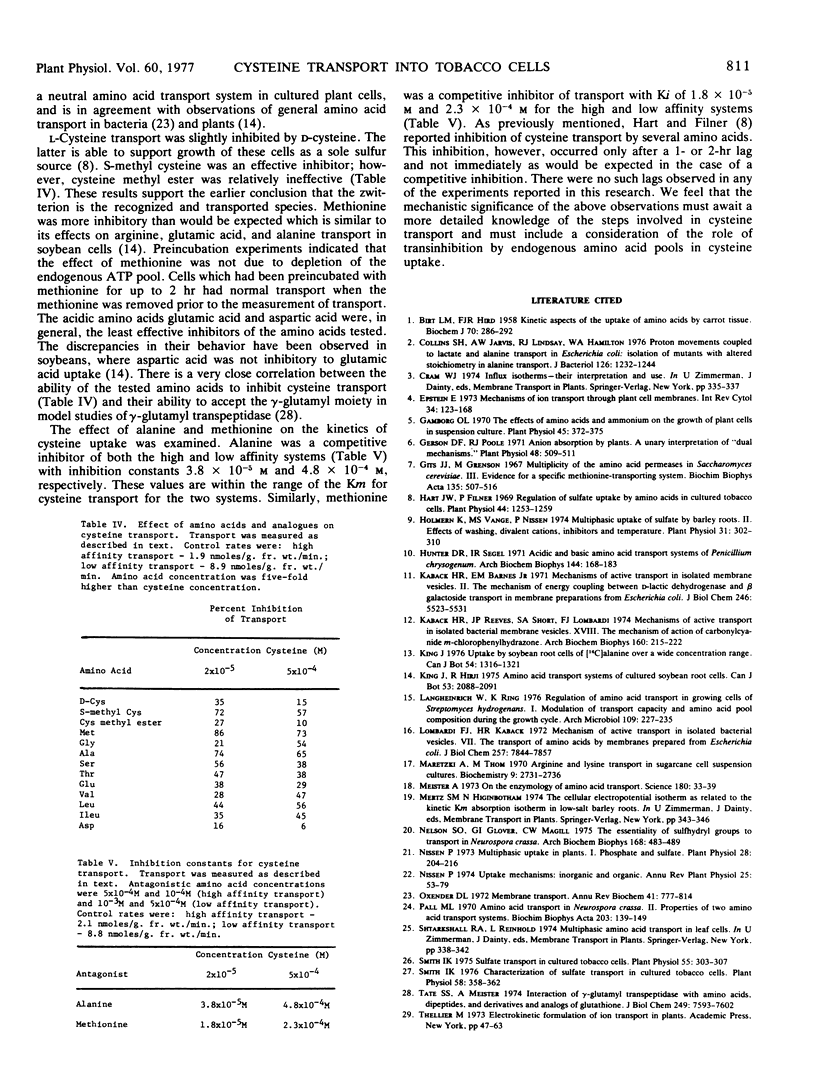
Selected References
These references are in PubMed. This may not be the complete list of references from this article.
- BIRT L. M., HIRD F. J. Kinetic aspects of the uptake of amino acids by carrot tissue. Biochem J. 1958 Oct;70(2):286–292. doi: 10.1042/bj0700286. [DOI] [PMC free article] [PubMed] [Google Scholar]
- Collins S. H., Jarvis A. W., Lindsay R. J., Hamilton W. A. Proton movements coupled to lactate and alanine transport in Escherichia coli: isolation of mutants with altered stoichiometry in alanine transport. J Bacteriol. 1976 Jun;126(3):1232–1244. doi: 10.1128/jb.126.3.1232-1244.1976. [DOI] [PMC free article] [PubMed] [Google Scholar]
- Gamborg O. L. The effects of amino acids and ammonium on the growth of plant cells in suspension culture. Plant Physiol. 1970 Apr;45(4):372–375. doi: 10.1104/pp.45.4.372. [DOI] [PMC free article] [PubMed] [Google Scholar]
- Gerson D. F., Poole R. J. Anion absorption by plants: a unary interpretation of "dual mechanisms". Plant Physiol. 1971 Oct;48(4):509–511. doi: 10.1104/pp.48.4.509. [DOI] [PMC free article] [PubMed] [Google Scholar]
- Hart J. W., Filner P. Regulation of sulfate uptake by amino acids in cultured tobacco cells. Plant Physiol. 1969 Sep;44(9):1253–1259. doi: 10.1104/pp.44.9.1253. [DOI] [PMC free article] [PubMed] [Google Scholar]
- Hunter D. R., Segel I. H. Acidic and basic amino acid transport systems of Penicillium chrysogenum. Arch Biochem Biophys. 1971 May;144(1):168–183. doi: 10.1016/0003-9861(71)90466-8. [DOI] [PubMed] [Google Scholar]
- Kaback H. R., Barnes E. M., Jr Mechanisms of active transport in isolated membrane vesicles. II. The mechanism of energy coupling between D-lactic dehydrogenase and beta-galactoside transport in membrane preparations from Escherichia coli. J Biol Chem. 1971 Sep 10;246(17):5523–5531. [PubMed] [Google Scholar]
- Kaback H. R., Reeves J. P., Short S. A., Lombardi F. J. Mechanisms of active transport in isolated bacterial membrane vesicles. 18. The mechanism of action of carbonylcyanide m-chlorophenylhydrazone. Arch Biochem Biophys. 1974 Jan;160(1):215–222. doi: 10.1016/s0003-9861(74)80028-7. [DOI] [PubMed] [Google Scholar]
- Langheinrich W., Ring K. Regulation of amino acid transport in growing cells of Streptomyces hydrogenans. I. Modulation of transport capacity and amino acid pool composition during the growth cycle. Arch Microbiol. 1976 Sep 1;109(3):227–235. doi: 10.1007/BF00446633. [DOI] [PubMed] [Google Scholar]
- Lombardi F. J., Kaback H. R. Mechanisms of active transport in isolated bacterial membrane vesicles. 8. The transport of amino acids by membranes prepared from Escherichia coli. J Biol Chem. 1972 Dec 25;247(24):7844–7857. [PubMed] [Google Scholar]
- Maretzki A., Thom M. Arginine and lysine transport in sugarcane cell suspension cultures. Biochemistry. 1970 Jun 23;9(13):2731–2736. doi: 10.1021/bi00815a022. [DOI] [PubMed] [Google Scholar]
- Nelson S. O., Glover G. I., Magill C. W. The essentiality of sulfhydryl groups to transport in Neurospora crassa. Arch Biochem Biophys. 1975 Jun;168(2):483–489. doi: 10.1016/0003-9861(75)90278-7. [DOI] [PubMed] [Google Scholar]
- Oxender D. L. Membrane transport. Annu Rev Biochem. 1972;41(10):777–814. doi: 10.1146/annurev.bi.41.070172.004021. [DOI] [PubMed] [Google Scholar]
- Pall M. L. Amino acid transport in Neurospora crassa. II. Properties of a basic amino acid transport system. Biochim Biophys Acta. 1970 Mar 17;203(1):139–149. doi: 10.1016/0005-2736(70)90044-1. [DOI] [PubMed] [Google Scholar]
- Smith I. K. Characterization of sulfate transport in cultured tobacco cells. Plant Physiol. 1976 Sep;58(3):358–362. doi: 10.1104/pp.58.3.358. [DOI] [PMC free article] [PubMed] [Google Scholar]
- Smith I. K. Sulfate transport in cultured tobacco cells. Plant Physiol. 1975 Feb;55(2):303–307. doi: 10.1104/pp.55.2.303. [DOI] [PMC free article] [PubMed] [Google Scholar]
- Tate S. S., Meister A. Interaction of gamma-glutamyl transpeptidase with amino acids, dipeptides, and derivatives and analogs of glutathione. J Biol Chem. 1974 Dec 10;249(23):7593–7602. [PubMed] [Google Scholar]