Abstract
The principal forces of protein folding--hydrophobicity and conformational entropy--are nonspecific. A long-standing puzzle has, therefore, been: What forces drive the formation of the specific internal architectures in globular proteins? We find that any self-avoiding flexible polymer molecule will develop large amounts of secondary structure, helices and parallel and antiparallel sheets, as it is driven to increasing compactness by any force of attraction among the chain monomers. Thus structure formation arises from the severity of steric constraints in compact polymers. This steric principle of organization can account for why short helices are stable in globular proteins, why there are parallel and anti-parallel sheets in proteins, and why weakly unfolded proteins have some secondary structure. On this basis, it should be possible to construct copolymers, not necessarily using amino acids, that can collapse to maximum compactness in incompatible solvents and that should then have structural organization resembling that of proteins.
Full text
PDF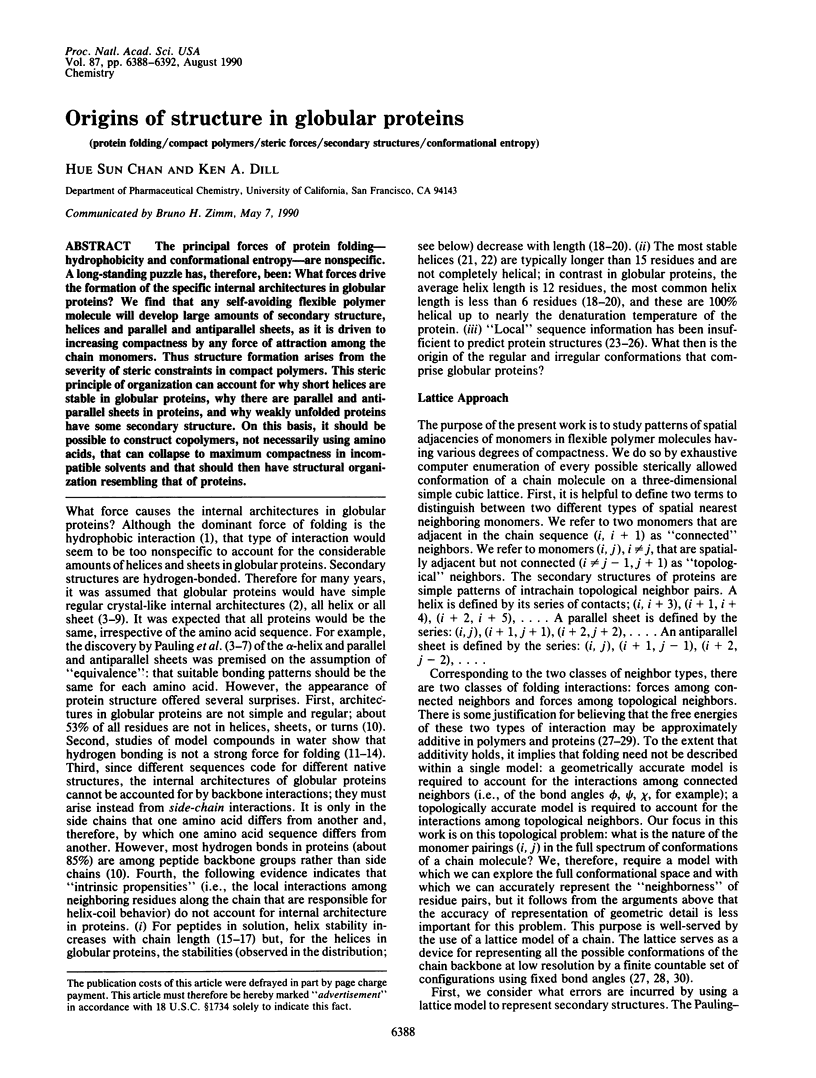
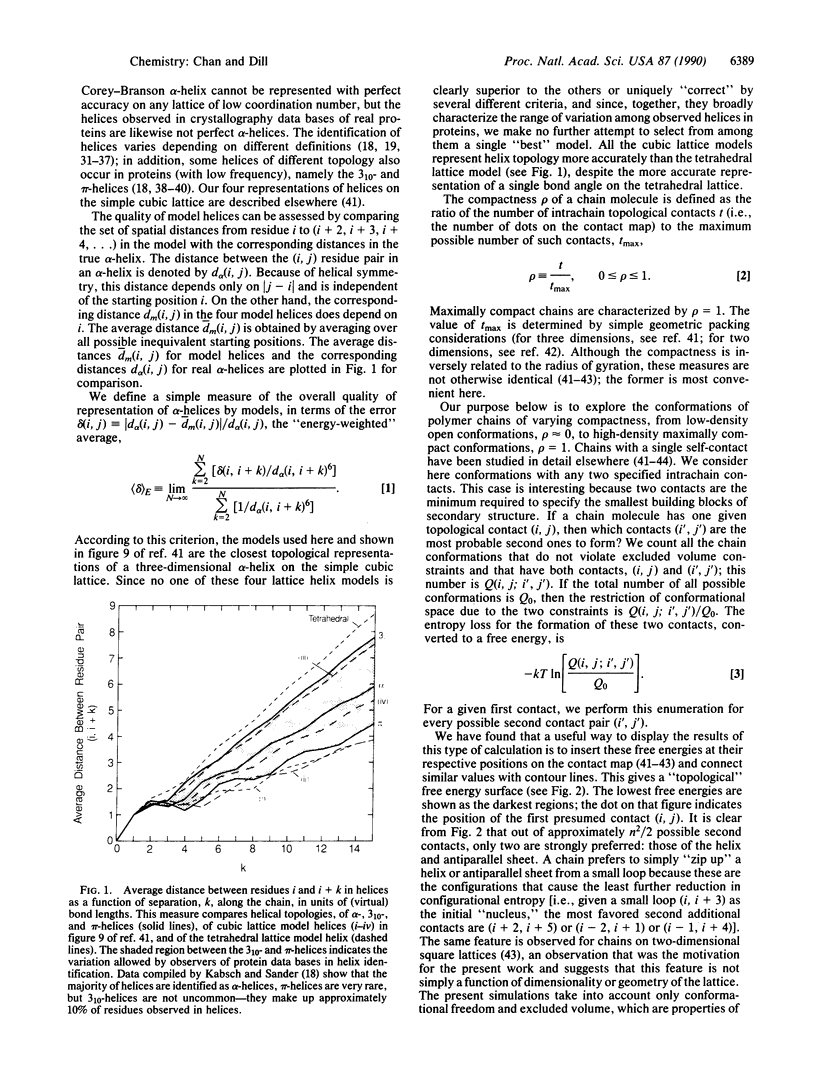
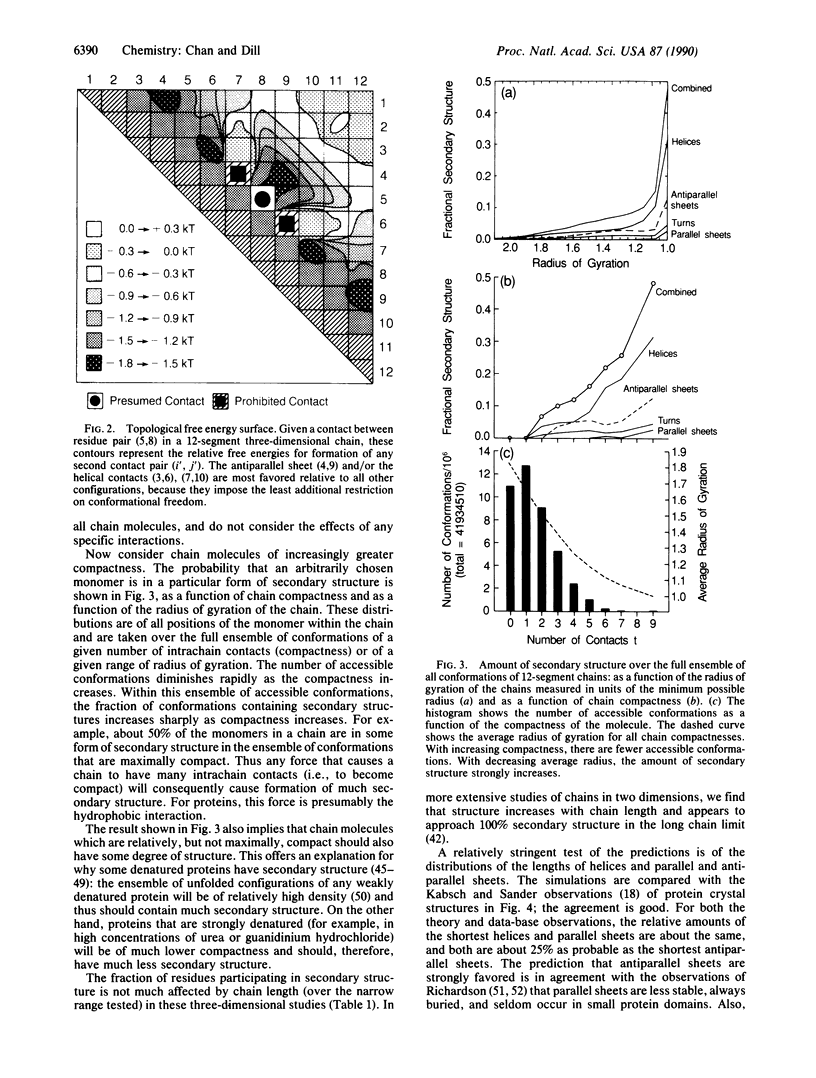
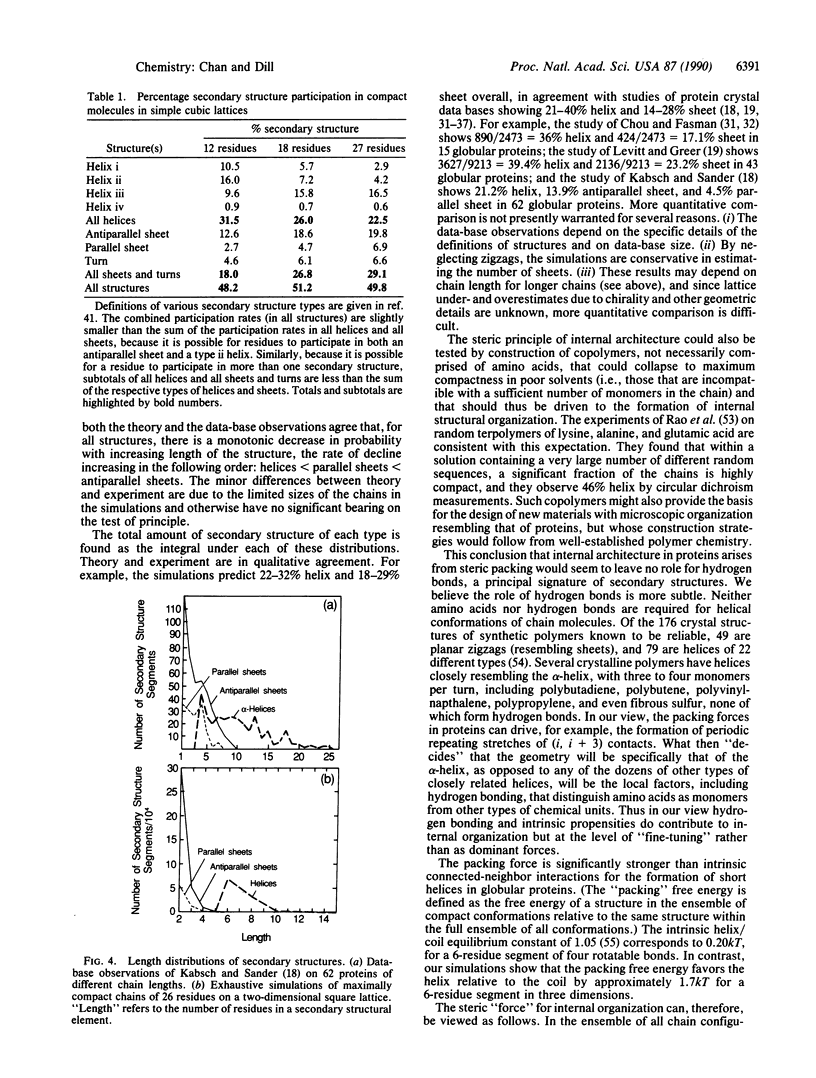
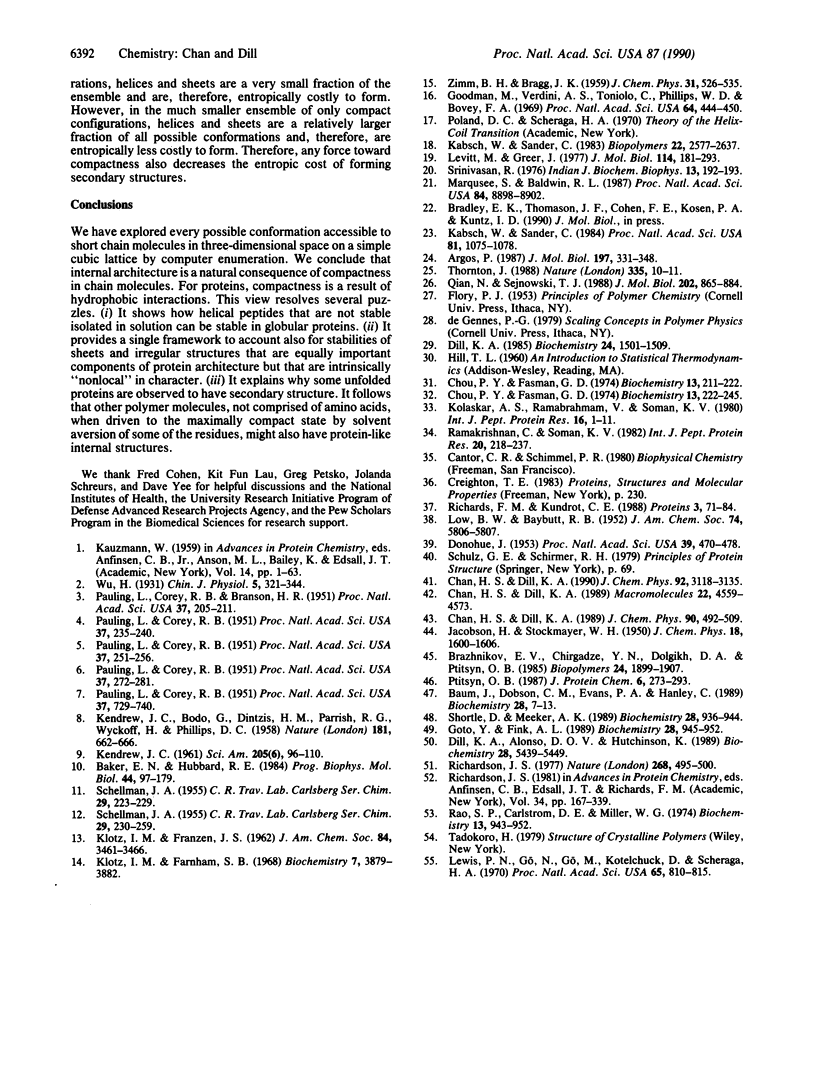
Images in this article
Selected References
These references are in PubMed. This may not be the complete list of references from this article.
- Argos P. Analysis of sequence-similar pentapeptides in unrelated protein tertiary structures. Strategies for protein folding and a guide for site-directed mutagenesis. J Mol Biol. 1987 Sep 20;197(2):331–348. doi: 10.1016/0022-2836(87)90127-6. [DOI] [PubMed] [Google Scholar]
- Baker E. N., Hubbard R. E. Hydrogen bonding in globular proteins. Prog Biophys Mol Biol. 1984;44(2):97–179. doi: 10.1016/0079-6107(84)90007-5. [DOI] [PubMed] [Google Scholar]
- Baum J., Dobson C. M., Evans P. A., Hanley C. Characterization of a partly folded protein by NMR methods: studies on the molten globule state of guinea pig alpha-lactalbumin. Biochemistry. 1989 Jan 10;28(1):7–13. doi: 10.1021/bi00427a002. [DOI] [PubMed] [Google Scholar]
- Brazhnikov E. V., Chirgadze YuN, Dolgikh D. A., Ptitsyn O. B. Noncooperative temperature melting of a globular protein without specific tertiary structure: acid form of bovine carbonic anhydrase B. Biopolymers. 1985 Oct;24(10):1899–1907. doi: 10.1002/bip.360241005. [DOI] [PubMed] [Google Scholar]
- Chou P. Y., Fasman G. D. Conformational parameters for amino acids in helical, beta-sheet, and random coil regions calculated from proteins. Biochemistry. 1974 Jan 15;13(2):211–222. doi: 10.1021/bi00699a001. [DOI] [PubMed] [Google Scholar]
- Chou P. Y., Fasman G. D. Prediction of protein conformation. Biochemistry. 1974 Jan 15;13(2):222–245. doi: 10.1021/bi00699a002. [DOI] [PubMed] [Google Scholar]
- Dill K. A., Alonso D. O., Hutchinson K. Thermal stabilities of globular proteins. Biochemistry. 1989 Jun 27;28(13):5439–5449. doi: 10.1021/bi00439a019. [DOI] [PubMed] [Google Scholar]
- Dill K. A. Theory for the folding and stability of globular proteins. Biochemistry. 1985 Mar 12;24(6):1501–1509. doi: 10.1021/bi00327a032. [DOI] [PubMed] [Google Scholar]
- Donohue J. Hydrogen Bonded Helical Configurations of the Polypeptide Chain. Proc Natl Acad Sci U S A. 1953 Jun;39(6):470–478. doi: 10.1073/pnas.39.6.470. [DOI] [PMC free article] [PubMed] [Google Scholar]
- Goodman M., Verdini A. S., Toniolo C., Phillips W. D., Bovey F. A. Sensitive criteria for the critical size for helix formation in oligopeptides. Proc Natl Acad Sci U S A. 1969 Oct;64(2):444–450. doi: 10.1073/pnas.64.2.444. [DOI] [PMC free article] [PubMed] [Google Scholar]
- Goto Y., Fink A. L. Conformational states of beta-lactamase: molten-globule states at acidic and alkaline pH with high salt. Biochemistry. 1989 Feb 7;28(3):945–952. doi: 10.1021/bi00429a004. [DOI] [PubMed] [Google Scholar]
- KENDREW J. C., BODO G., DINTZIS H. M., PARRISH R. G., WYCKOFF H., PHILLIPS D. C. A three-dimensional model of the myoglobin molecule obtained by x-ray analysis. Nature. 1958 Mar 8;181(4610):662–666. doi: 10.1038/181662a0. [DOI] [PubMed] [Google Scholar]
- KENDREW J. C. The three-dimensional structure of a protein molecule. Sci Am. 1961 Dec;205:96–110. doi: 10.1038/scientificamerican1261-96. [DOI] [PubMed] [Google Scholar]
- Kabsch W., Sander C. Dictionary of protein secondary structure: pattern recognition of hydrogen-bonded and geometrical features. Biopolymers. 1983 Dec;22(12):2577–2637. doi: 10.1002/bip.360221211. [DOI] [PubMed] [Google Scholar]
- Kabsch W., Sander C. On the use of sequence homologies to predict protein structure: identical pentapeptides can have completely different conformations. Proc Natl Acad Sci U S A. 1984 Feb;81(4):1075–1078. doi: 10.1073/pnas.81.4.1075. [DOI] [PMC free article] [PubMed] [Google Scholar]
- Klotz I. M., Farnham S. B. Stability of an amide-hydrogen bond in an apolar environment. Biochemistry. 1968 Nov;7(11):3879–3882. doi: 10.1021/bi00851a013. [DOI] [PubMed] [Google Scholar]
- Kolaskar A. S., Ramabrahmam V., Soman K. V. Reversals of polypeptide chain in globular proteins. Int J Pept Protein Res. 1980 Jul;16(1):1–11. doi: 10.1111/j.1399-3011.1980.tb02929.x. [DOI] [PubMed] [Google Scholar]
- Levitt M., Greer J. Automatic identification of secondary structure in globular proteins. J Mol Biol. 1977 Aug 5;114(2):181–239. doi: 10.1016/0022-2836(77)90207-8. [DOI] [PubMed] [Google Scholar]
- Lewis P. N., Go N., Go M., Kotelchuck D., Scheraga H. A. Helix probability profiles of denatured proteins and their correlation with native structures. Proc Natl Acad Sci U S A. 1970 Apr;65(4):810–815. doi: 10.1073/pnas.65.4.810. [DOI] [PMC free article] [PubMed] [Google Scholar]
- Marqusee S., Baldwin R. L. Helix stabilization by Glu-...Lys+ salt bridges in short peptides of de novo design. Proc Natl Acad Sci U S A. 1987 Dec;84(24):8898–8902. doi: 10.1073/pnas.84.24.8898. [DOI] [PMC free article] [PubMed] [Google Scholar]
- PAULING L., COREY R. B. Atomic coordinates and structure factors for two helical configurations of polypeptide chains. Proc Natl Acad Sci U S A. 1951 May;37(5):235–240. doi: 10.1073/pnas.37.5.235. [DOI] [PMC free article] [PubMed] [Google Scholar]
- PAULING L., COREY R. B., BRANSON H. R. The structure of proteins; two hydrogen-bonded helical configurations of the polypeptide chain. Proc Natl Acad Sci U S A. 1951 Apr;37(4):205–211. doi: 10.1073/pnas.37.4.205. [DOI] [PMC free article] [PubMed] [Google Scholar]
- PAULING L., COREY R. B. The pleated sheet, a new layer configuration of polypeptide chains. Proc Natl Acad Sci U S A. 1951 May;37(5):251–256. doi: 10.1073/pnas.37.5.251. [DOI] [PMC free article] [PubMed] [Google Scholar]
- PAULING L., COREY R. B. The structure of fibrous proteins of the collagen-gelatin group. Proc Natl Acad Sci U S A. 1951 May;37(5):272–281. doi: 10.1073/pnas.37.5.272. [DOI] [PMC free article] [PubMed] [Google Scholar]
- Pauling L., Corey R. B. Configurations of Polypeptide Chains With Favored Orientations Around Single Bonds: Two New Pleated Sheets. Proc Natl Acad Sci U S A. 1951 Nov;37(11):729–740. doi: 10.1073/pnas.37.11.729. [DOI] [PMC free article] [PubMed] [Google Scholar]
- Qian N., Sejnowski T. J. Predicting the secondary structure of globular proteins using neural network models. J Mol Biol. 1988 Aug 20;202(4):865–884. doi: 10.1016/0022-2836(88)90564-5. [DOI] [PubMed] [Google Scholar]
- Ramakrishnan C., Soman K. V. Identification of secondary structures in globular proteins--a new algorithm. Int J Pept Protein Res. 1982 Sep;20(3):218–237. doi: 10.1111/j.1399-3011.1982.tb03052.x. [DOI] [PubMed] [Google Scholar]
- Rao S. P., Carlstrom D. E., Miller W. G. Collapsed structure polymers. A scattergun approach to amino acid copolymers. Biochemistry. 1974 Feb 26;13(5):943–952. doi: 10.1021/bi00702a019. [DOI] [PubMed] [Google Scholar]
- Richards F. M., Kundrot C. E. Identification of structural motifs from protein coordinate data: secondary structure and first-level supersecondary structure. Proteins. 1988;3(2):71–84. doi: 10.1002/prot.340030202. [DOI] [PubMed] [Google Scholar]
- Richardson J. S. beta-Sheet topology and the relatedness of proteins. Nature. 1977 Aug 11;268(5620):495–500. doi: 10.1038/268495a0. [DOI] [PubMed] [Google Scholar]
- SCHELLMAN J. A. The stability of hydrogen-bonded peptide structures in aqueous solution. C R Trav Lab Carlsberg Chim. 1955;29(14-15):230–259. [PubMed] [Google Scholar]
- SCHELLMAN J. A. The thermodynamics of urea solutions and the heat of formation of the peptide hydrogen bond. C R Trav Lab Carlsberg Chim. 1955;29(14-15):223–229. [PubMed] [Google Scholar]
- Shortle D., Meeker A. K. Residual structure in large fragments of staphylococcal nuclease: effects of amino acid substitutions. Biochemistry. 1989 Feb 7;28(3):936–944. doi: 10.1021/bi00429a003. [DOI] [PubMed] [Google Scholar]
- Srinivasan R. Helical length distribution from protein crystallographic data. Indian J Biochem Biophys. 1976 Jun;13(2):192–193. [PubMed] [Google Scholar]
- Thornton J. M. Protein structure. The shape of things to come? Nature. 1988 Sep 1;335(6185):10–11. doi: 10.1038/335010a0. [DOI] [PubMed] [Google Scholar]