Abstract
Sequence homology and molecular modeling studies have suggested that the N-terminal one-third of the flavirvirus nonstructural protein NS3 functions as a trypsin-like serine protease. To examine the putative proteolytic activity of NS3, segments of the yellow fever virus genome were subcloned into plasmid transcription/translation vectors and cell-free translation products were characterized. The results suggest that a protease activity encoded within NS2B and the N-terminal one-third of yellow fever virus NS3 is capable of cis-acting site-specific proteolysis at the NS2B-NS3 cleavage site and dilution-insensitive cleavage of the NS2A-NS2B site. Site-directed mutagenesis of the His-53, Asp-77, and Ser-138 residues of NS3 that compose the proposed catalytic triad implicates this domain as a serine protease. Infectious virus was not recovered from mammalian cells transfected with RNAs transcribed from full-length yellow fever virus cDNA templates containing mutations at Ser-138 (which abolish or dramatically reduce protease activity in vitro), suggesting that the protease is required for viral replication.
Full text
PDF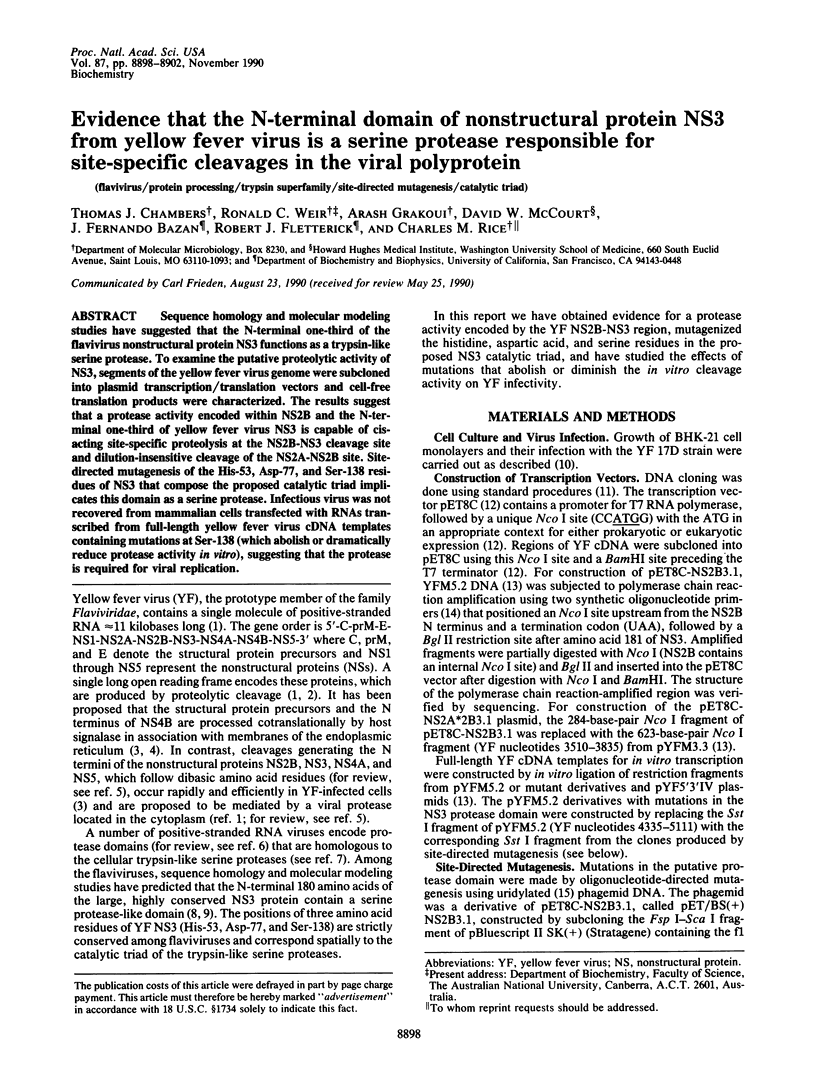
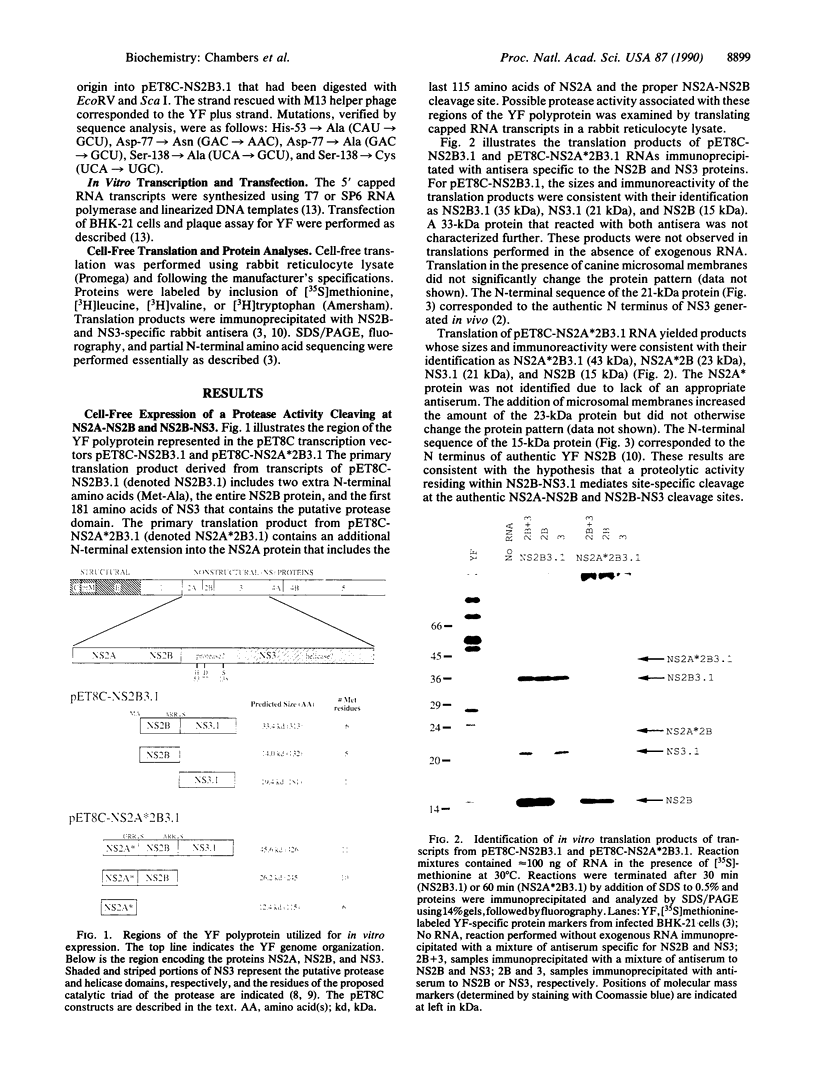
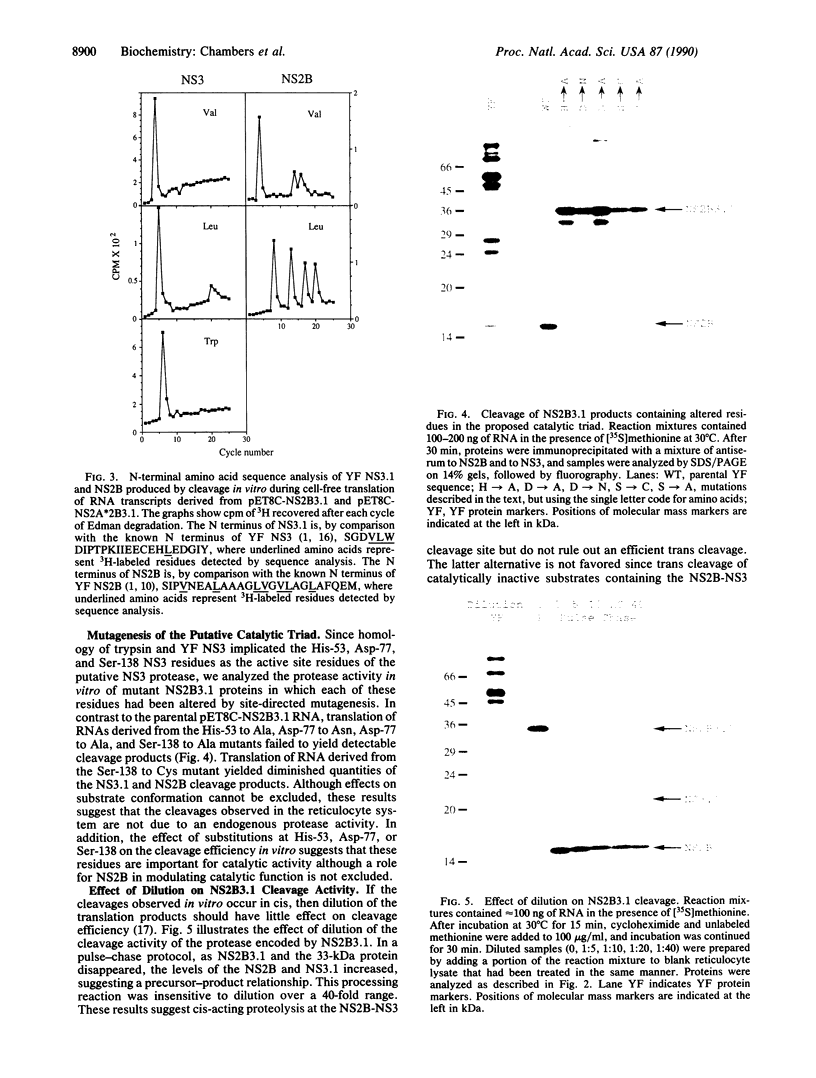
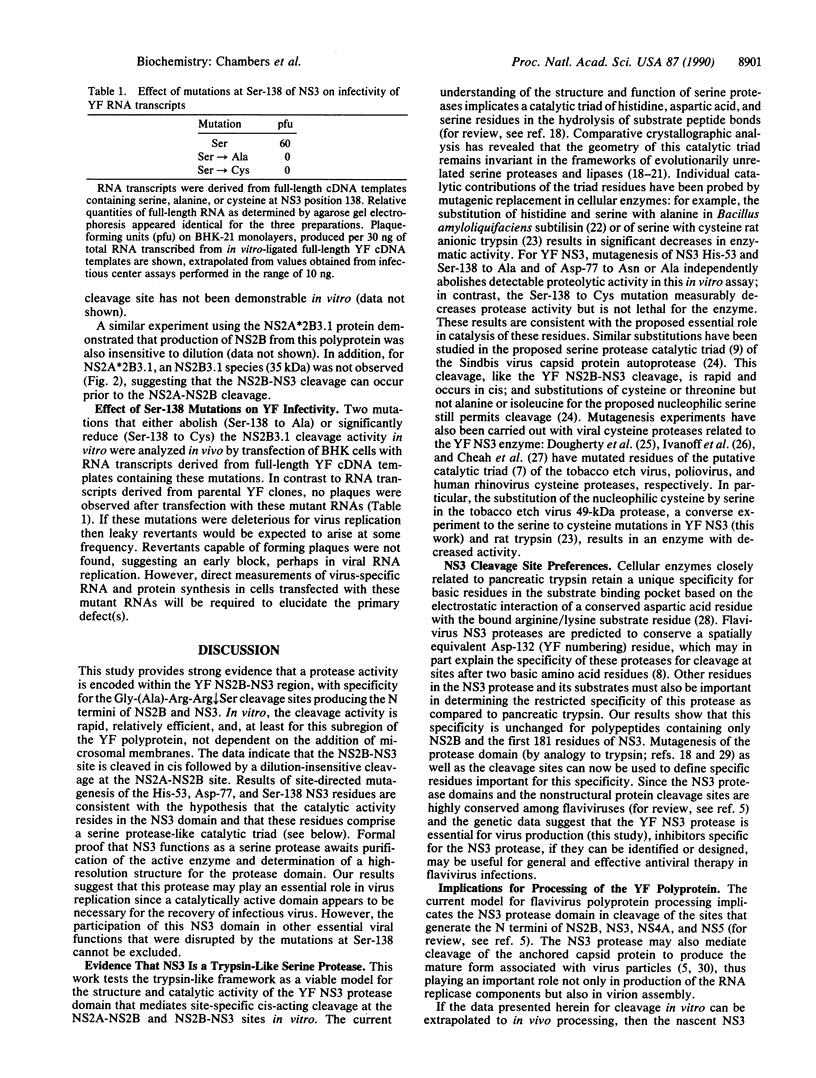
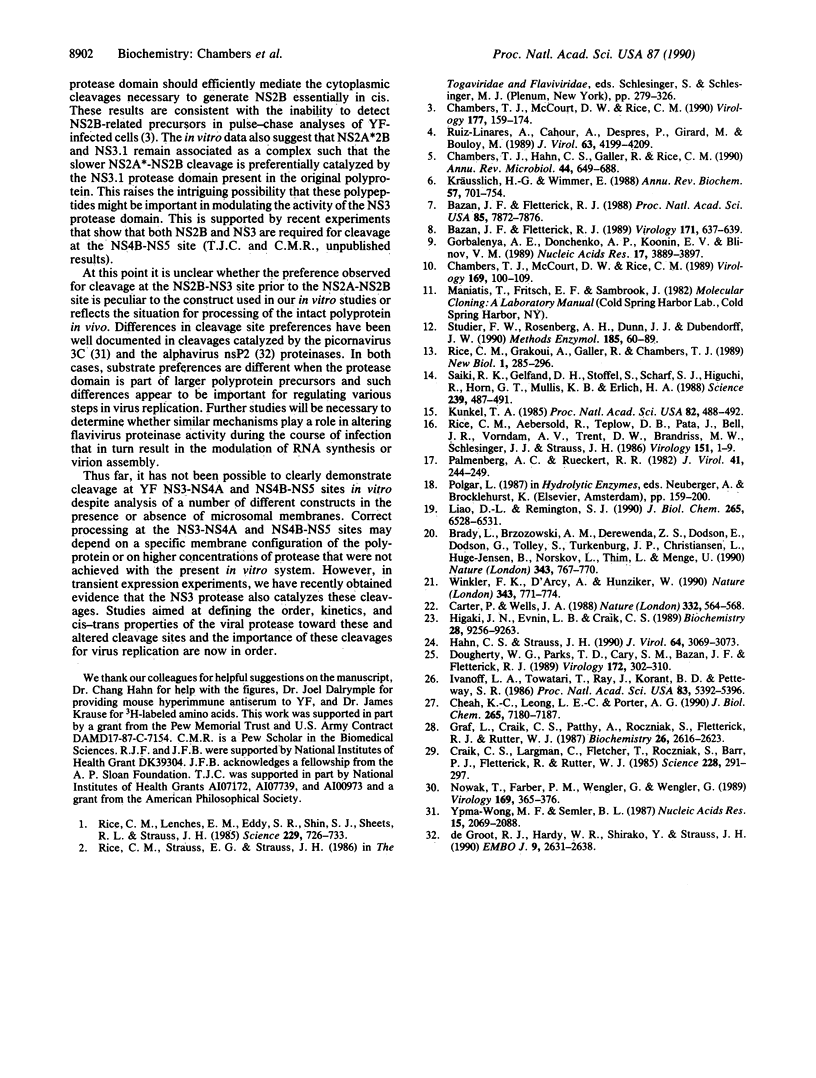
Images in this article
Selected References
These references are in PubMed. This may not be the complete list of references from this article.
- Bazan J. F., Fletterick R. J. Detection of a trypsin-like serine protease domain in flaviviruses and pestiviruses. Virology. 1989 Aug;171(2):637–639. doi: 10.1016/0042-6822(89)90639-9. [DOI] [PubMed] [Google Scholar]
- Bazan J. F., Fletterick R. J. Viral cysteine proteases are homologous to the trypsin-like family of serine proteases: structural and functional implications. Proc Natl Acad Sci U S A. 1988 Nov;85(21):7872–7876. doi: 10.1073/pnas.85.21.7872. [DOI] [PMC free article] [PubMed] [Google Scholar]
- Brady L., Brzozowski A. M., Derewenda Z. S., Dodson E., Dodson G., Tolley S., Turkenburg J. P., Christiansen L., Huge-Jensen B., Norskov L. A serine protease triad forms the catalytic centre of a triacylglycerol lipase. Nature. 1990 Feb 22;343(6260):767–770. doi: 10.1038/343767a0. [DOI] [PubMed] [Google Scholar]
- Carter P., Wells J. A. Dissecting the catalytic triad of a serine protease. Nature. 1988 Apr 7;332(6164):564–568. doi: 10.1038/332564a0. [DOI] [PubMed] [Google Scholar]
- Chambers T. J., Hahn C. S., Galler R., Rice C. M. Flavivirus genome organization, expression, and replication. Annu Rev Microbiol. 1990;44:649–688. doi: 10.1146/annurev.mi.44.100190.003245. [DOI] [PubMed] [Google Scholar]
- Chambers T. J., McCourt D. W., Rice C. M. Production of yellow fever virus proteins in infected cells: identification of discrete polyprotein species and analysis of cleavage kinetics using region-specific polyclonal antisera. Virology. 1990 Jul;177(1):159–174. doi: 10.1016/0042-6822(90)90470-c. [DOI] [PubMed] [Google Scholar]
- Chambers T. J., McCourt D. W., Rice C. M. Yellow fever virus proteins NS2A, NS2B, and NS4B: identification and partial N-terminal amino acid sequence analysis. Virology. 1989 Mar;169(1):100–109. doi: 10.1016/0042-6822(89)90045-7. [DOI] [PubMed] [Google Scholar]
- Cheah K. C., Leong L. E., Porter A. G. Site-directed mutagenesis suggests close functional relationship between a human rhinovirus 3C cysteine protease and cellular trypsin-like serine proteases. J Biol Chem. 1990 May 5;265(13):7180–7187. [PubMed] [Google Scholar]
- Craik C. S., Largman C., Fletcher T., Roczniak S., Barr P. J., Fletterick R., Rutter W. J. Redesigning trypsin: alteration of substrate specificity. Science. 1985 Apr 19;228(4697):291–297. doi: 10.1126/science.3838593. [DOI] [PubMed] [Google Scholar]
- Dougherty W. G., Parks T. D., Cary S. M., Bazan J. F., Fletterick R. J. Characterization of the catalytic residues of the tobacco etch virus 49-kDa proteinase. Virology. 1989 Sep;172(1):302–310. doi: 10.1016/0042-6822(89)90132-3. [DOI] [PubMed] [Google Scholar]
- Gorbalenya A. E., Donchenko A. P., Koonin E. V., Blinov V. M. N-terminal domains of putative helicases of flavi- and pestiviruses may be serine proteases. Nucleic Acids Res. 1989 May 25;17(10):3889–3897. doi: 10.1093/nar/17.10.3889. [DOI] [PMC free article] [PubMed] [Google Scholar]
- Graf L., Craik C. S., Patthy A., Roczniak S., Fletterick R. J., Rutter W. J. Selective alteration of substrate specificity by replacement of aspartic acid-189 with lysine in the binding pocket of trypsin. Biochemistry. 1987 May 5;26(9):2616–2623. doi: 10.1021/bi00383a031. [DOI] [PubMed] [Google Scholar]
- Hahn C. S., Strauss J. H. Site-directed mutagenesis of the proposed catalytic amino acids of the Sindbis virus capsid protein autoprotease. J Virol. 1990 Jun;64(6):3069–3073. doi: 10.1128/jvi.64.6.3069-3073.1990. [DOI] [PMC free article] [PubMed] [Google Scholar]
- Higaki J. N., Evnin L. B., Craik C. S. Introduction of a cysteine protease active site into trypsin. Biochemistry. 1989 Nov 28;28(24):9256–9263. doi: 10.1021/bi00450a004. [DOI] [PubMed] [Google Scholar]
- Ivanoff L. A., Towatari T., Ray J., Korant B. D., Petteway S. R., Jr Expression and site-specific mutagenesis of the poliovirus 3C protease in Escherichia coli. Proc Natl Acad Sci U S A. 1986 Aug;83(15):5392–5396. doi: 10.1073/pnas.83.15.5392. [DOI] [PMC free article] [PubMed] [Google Scholar]
- Kräusslich H. G., Wimmer E. Viral proteinases. Annu Rev Biochem. 1988;57:701–754. doi: 10.1146/annurev.bi.57.070188.003413. [DOI] [PubMed] [Google Scholar]
- Kunkel T. A. Rapid and efficient site-specific mutagenesis without phenotypic selection. Proc Natl Acad Sci U S A. 1985 Jan;82(2):488–492. doi: 10.1073/pnas.82.2.488. [DOI] [PMC free article] [PubMed] [Google Scholar]
- Liao D. I., Remington S. J. Structure of wheat serine carboxypeptidase II at 3.5-A resolution. A new class of serine proteinase. J Biol Chem. 1990 Apr 25;265(12):6528–6531. doi: 10.2210/pdb2sc2/pdb. [DOI] [PubMed] [Google Scholar]
- Nowak T., Färber P. M., Wengler G., Wengler G. Analyses of the terminal sequences of West Nile virus structural proteins and of the in vitro translation of these proteins allow the proposal of a complete scheme of the proteolytic cleavages involved in their synthesis. Virology. 1989 Apr;169(2):365–376. doi: 10.1016/0042-6822(89)90162-1. [DOI] [PubMed] [Google Scholar]
- Palmenberg A. C., Rueckert R. R. Evidence for intramolecular self-cleavage of picornaviral replicase precursors. J Virol. 1982 Jan;41(1):244–249. doi: 10.1128/jvi.41.1.244-249.1982. [DOI] [PMC free article] [PubMed] [Google Scholar]
- Rice C. M., Aebersold R., Teplow D. B., Pata J., Bell J. R., Vorndam A. V., Trent D. W., Brandriss M. W., Schlesinger J. J., Strauss J. H. Partial N-terminal amino acid sequences of three nonstructural proteins of two flaviviruses. Virology. 1986 May;151(1):1–9. doi: 10.1016/0042-6822(86)90098-x. [DOI] [PubMed] [Google Scholar]
- Rice C. M., Grakoui A., Galler R., Chambers T. J. Transcription of infectious yellow fever RNA from full-length cDNA templates produced by in vitro ligation. New Biol. 1989 Dec;1(3):285–296. [PubMed] [Google Scholar]
- Rice C. M., Lenches E. M., Eddy S. R., Shin S. J., Sheets R. L., Strauss J. H. Nucleotide sequence of yellow fever virus: implications for flavivirus gene expression and evolution. Science. 1985 Aug 23;229(4715):726–733. doi: 10.1126/science.4023707. [DOI] [PubMed] [Google Scholar]
- Ruiz-Linares A., Cahour A., Després P., Girard M., Bouloy M. Processing of yellow fever virus polyprotein: role of cellular proteases in maturation of the structural proteins. J Virol. 1989 Oct;63(10):4199–4209. doi: 10.1128/jvi.63.10.4199-4209.1989. [DOI] [PMC free article] [PubMed] [Google Scholar]
- Saiki R. K., Gelfand D. H., Stoffel S., Scharf S. J., Higuchi R., Horn G. T., Mullis K. B., Erlich H. A. Primer-directed enzymatic amplification of DNA with a thermostable DNA polymerase. Science. 1988 Jan 29;239(4839):487–491. doi: 10.1126/science.2448875. [DOI] [PubMed] [Google Scholar]
- Studier F. W., Rosenberg A. H., Dunn J. J., Dubendorff J. W. Use of T7 RNA polymerase to direct expression of cloned genes. Methods Enzymol. 1990;185:60–89. doi: 10.1016/0076-6879(90)85008-c. [DOI] [PubMed] [Google Scholar]
- Winkler F. K., D'Arcy A., Hunziker W. Structure of human pancreatic lipase. Nature. 1990 Feb 22;343(6260):771–774. doi: 10.1038/343771a0. [DOI] [PubMed] [Google Scholar]
- Ypma-Wong M. F., Semler B. L. In vitro molecular genetics as a tool for determining the differential cleavage specificities of the poliovirus 3C proteinase. Nucleic Acids Res. 1987 Mar 11;15(5):2069–2088. doi: 10.1093/nar/15.5.2069. [DOI] [PMC free article] [PubMed] [Google Scholar]
- de Groot R. J., Hardy W. R., Shirako Y., Strauss J. H. Cleavage-site preferences of Sindbis virus polyproteins containing the non-structural proteinase. Evidence for temporal regulation of polyprotein processing in vivo. EMBO J. 1990 Aug;9(8):2631–2638. doi: 10.1002/j.1460-2075.1990.tb07445.x. [DOI] [PMC free article] [PubMed] [Google Scholar]