Abstract
Both troponin C (TnC) and calmodulin share a remarkably similar tertiary motif that may be common to other Ca2(+)-binding proteins with activator activity. TnC plays a critical role in regulating muscle contraction and is particularly well-suited for structural analysis by site-directed mutation. Fast-twitch skeletal muscle TnC has two low-affinity Ca2(+)-binding sites (sites I and II), while in cardiac and slow-twitch skeletal muscle TnC site I is inactive. Recently, using protein engineering, we directly demonstrated that binding of Ca2+ to the low-affinity site(s) initiates muscle contraction. In the present study, we use mutagenesis to determine whether either of the low-affinity sites in cardiac TnC can trigger contraction in slow-twitch skeletal muscle fibers. In one Ca2(+)-binding mutant, Ca2(+)-binding to the dormant low-affinity site I was restored (CBM+I). In a second mutant, site I was activated while site II was inactivated (CBM+I-IIA). Both proteins had the predicted CA2(+)-binding characteristics, and both were able to associate with troponin I and troponin T to form a troponin complex and integrate into permeabilized slow-twitch skeletal muscle fibers. A comparison of NMR spectra shows the aromatic regions in the two proteins to be qualitatively similar without divalent cations but markedly different with Ca2+. Mutant CBM+I supported force generation in skinned slow skeletal muscle fibers but had Sr2+ and Ca2+ sensitivities similar to fast skeletal TnC. Mutant CBM+I-IIA was unable to restore Ca2(+)-dependent contraction to TnC-depleted skinned slow muscle fibers. The data directly demonstrate that low-affinity sites I and II have distinct functions and that only site II in cardiac TnC can trigger muscle contraction in slow-twitch skeletal muscle fibers. This principle of distinct, modular activities for Ca2(+)-binding sites in the same protein may apply to other members of the TnC/calmodulin family.
Full text
PDF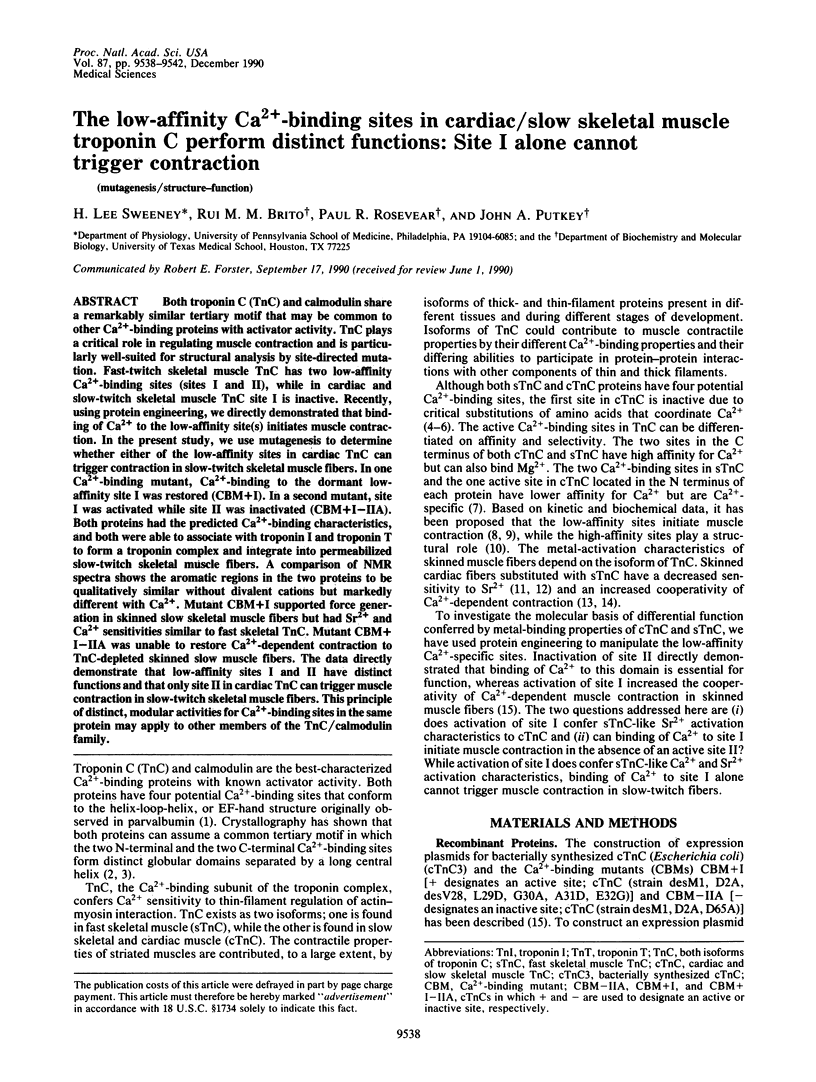
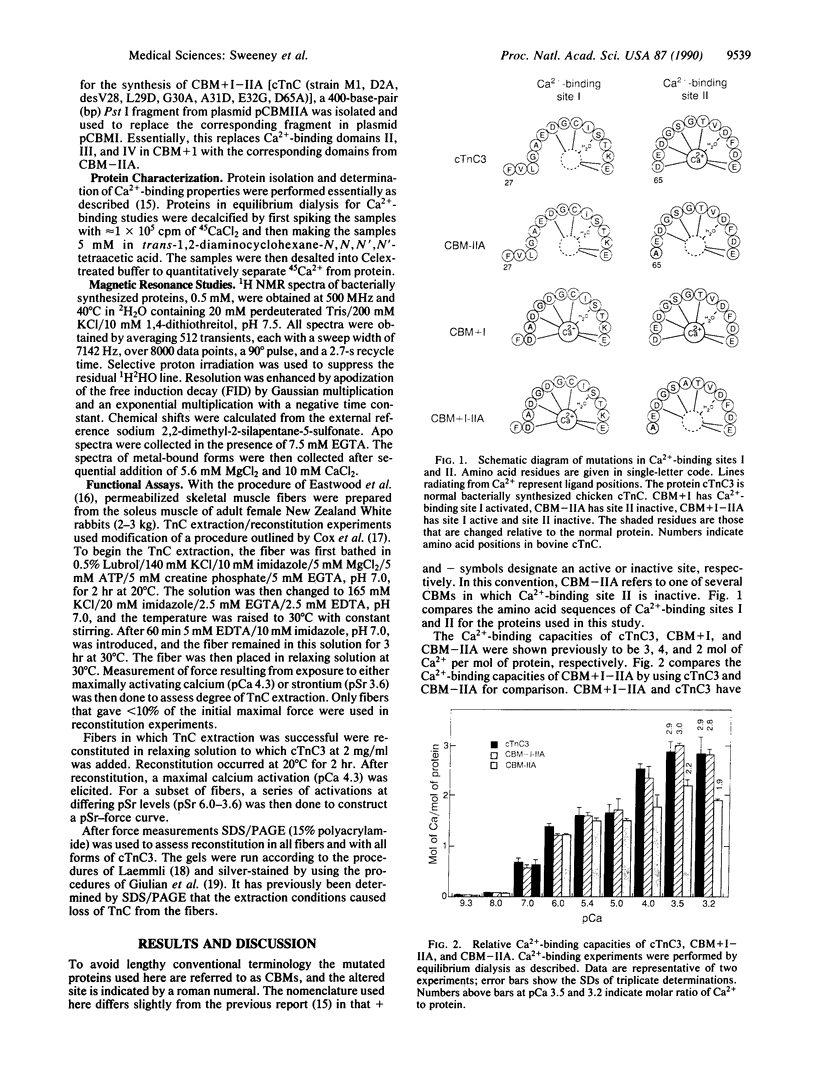
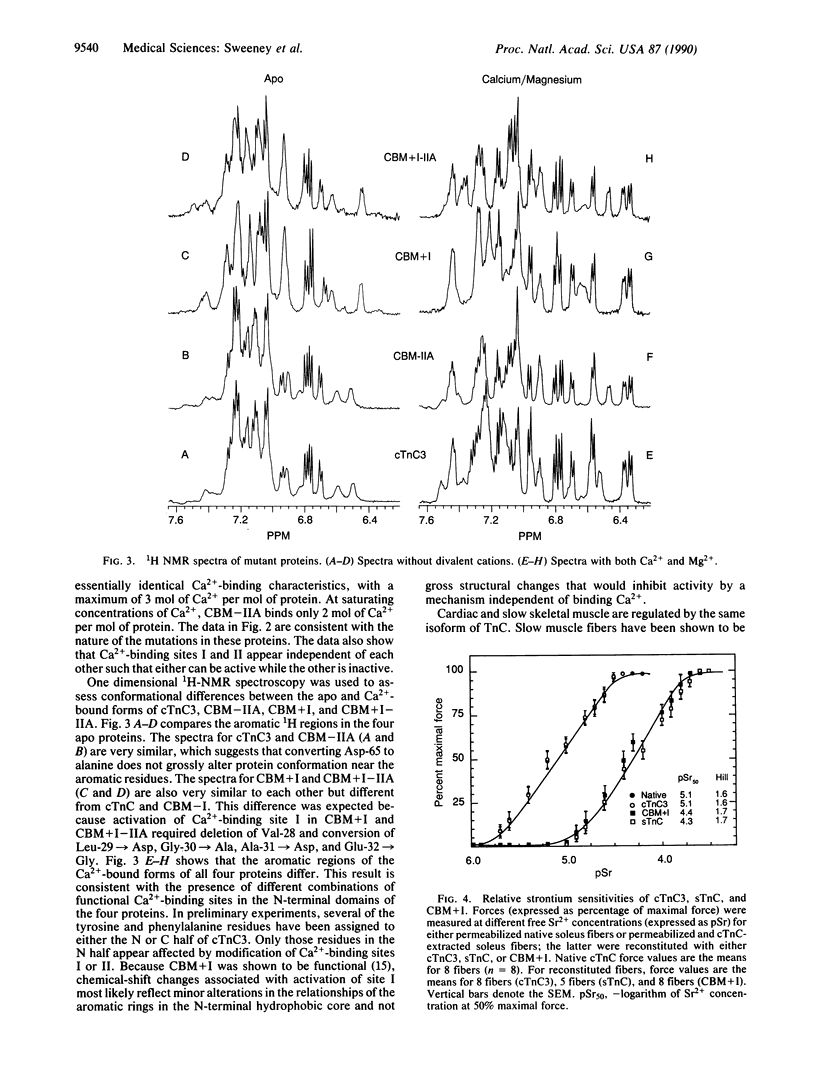
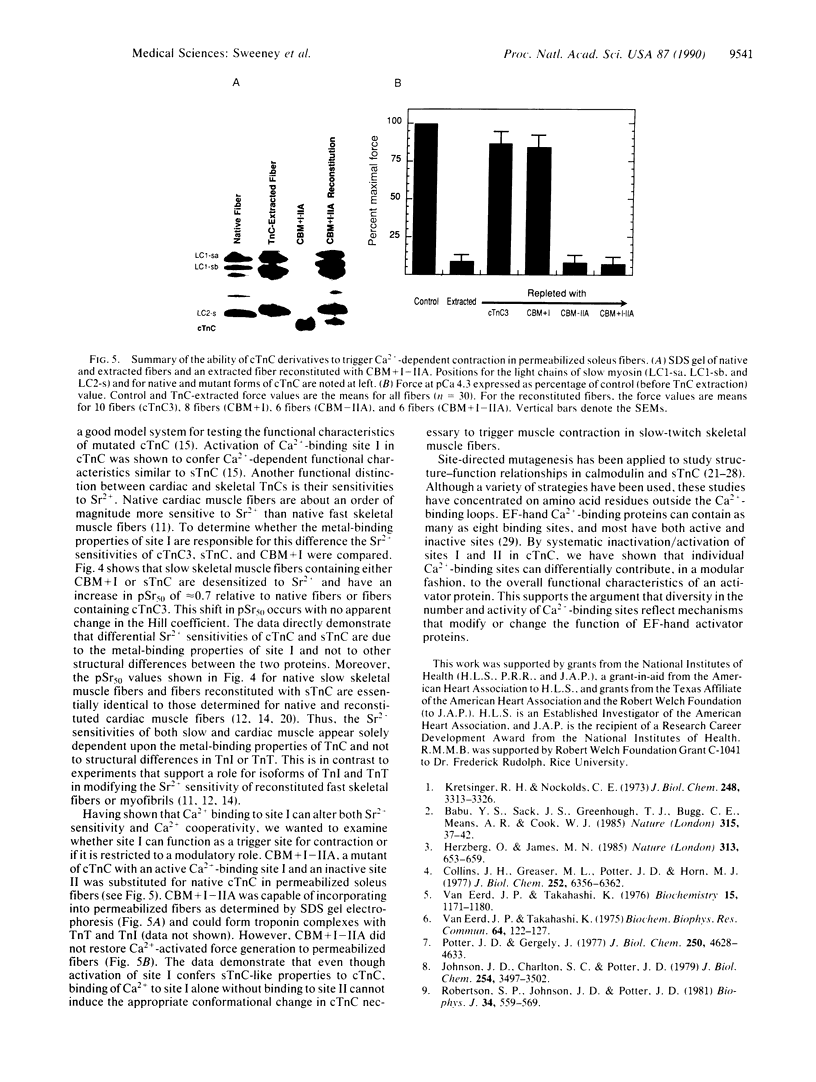
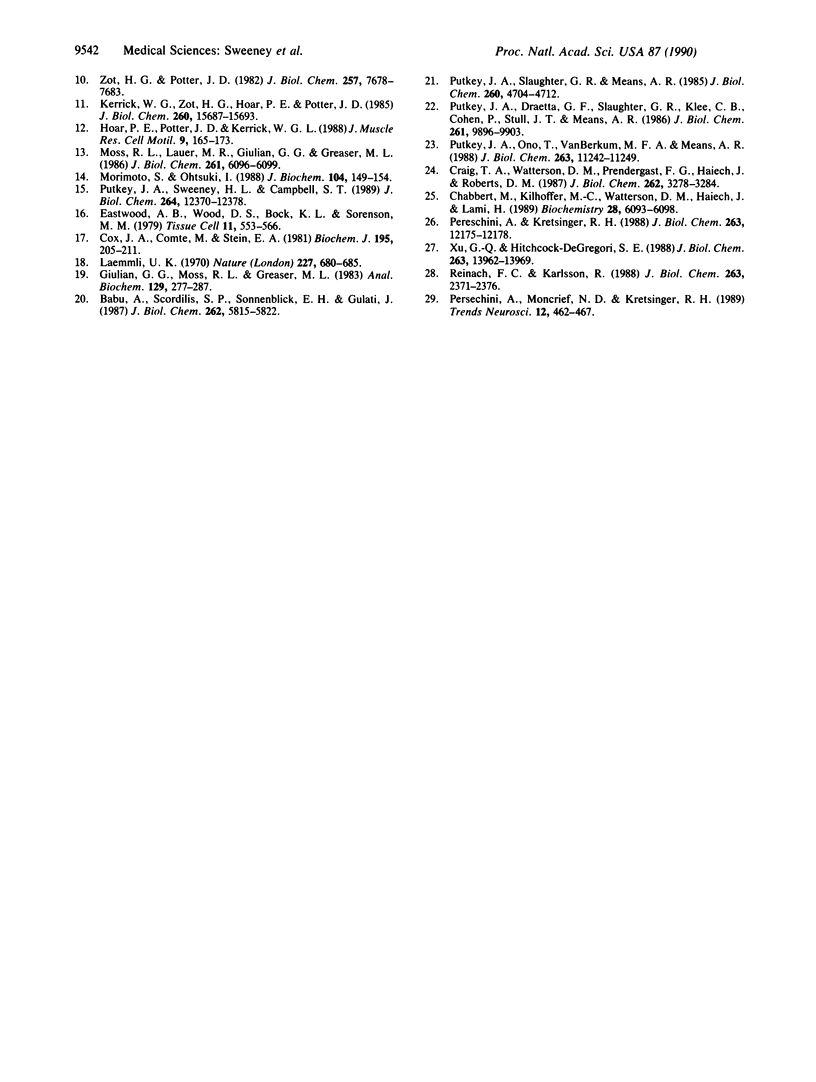
Images in this article
Selected References
These references are in PubMed. This may not be the complete list of references from this article.
- Babu A., Scordilis S. P., Sonnenblick E. H., Gulati J. The control of myocardial contraction with skeletal fast muscle troponin C. J Biol Chem. 1987 Apr 25;262(12):5815–5822. [PubMed] [Google Scholar]
- Babu Y. S., Sack J. S., Greenhough T. J., Bugg C. E., Means A. R., Cook W. J. Three-dimensional structure of calmodulin. Nature. 1985 May 2;315(6014):37–40. doi: 10.1038/315037a0. [DOI] [PubMed] [Google Scholar]
- Chabbert M., Kilhoffer M. C., Watterson D. M., Haiech J., Lami H. Time-resolved fluorescence study of VU-9 calmodulin, an engineered calmodulin possessing a single tryptophan residue. Biochemistry. 1989 Jul 11;28(14):6093–6098. doi: 10.1021/bi00440a054. [DOI] [PubMed] [Google Scholar]
- Cox J. A., Comte M., Stein E. A. Calmodulin-free skeletal-muscle troponin C prepared in the absence of urea. Biochem J. 1981 Apr 1;195(1):205–211. doi: 10.1042/bj1950205. [DOI] [PMC free article] [PubMed] [Google Scholar]
- Craig T. A., Watterson D. M., Prendergast F. G., Haiech J., Roberts D. M. Site-specific mutagenesis of the alpha-helices of calmodulin. Effects of altering a charge cluster in the helix that links the two halves of calmodulin. J Biol Chem. 1987 Mar 5;262(7):3278–3284. [PubMed] [Google Scholar]
- Eastwood A. B., Wood D. S., Bock K. L., Sorenson M. M. Chemically skinned mammalian skeletal muscle. I. The structure of skinned rabbit psoas. Tissue Cell. 1979;11(3):553–566. doi: 10.1016/0040-8166(79)90062-4. [DOI] [PubMed] [Google Scholar]
- Giulian G. G., Moss R. L., Greaser M. Improved methodology for analysis and quantitation of proteins on one-dimensional silver-stained slab gels. Anal Biochem. 1983 Mar;129(2):277–287. doi: 10.1016/0003-2697(83)90551-1. [DOI] [PubMed] [Google Scholar]
- Herzberg O., James M. N. Structure of the calcium regulatory muscle protein troponin-C at 2.8 A resolution. Nature. 1985 Feb 21;313(6004):653–659. doi: 10.1038/313653a0. [DOI] [PubMed] [Google Scholar]
- Hoar P. E., Potter J. D., Kerrick W. G. Skinned ventricular fibres: troponin C extraction is species-dependent and its replacement with skeletal troponin C changes Sr2+ activation properties. J Muscle Res Cell Motil. 1988 Apr;9(2):165–173. doi: 10.1007/BF01773738. [DOI] [PubMed] [Google Scholar]
- Johnson J. D., Charlton S. C., Potter J. D. A fluorescence stopped flow analysis of Ca2+ exchange with troponin C. J Biol Chem. 1979 May 10;254(9):3497–3502. [PubMed] [Google Scholar]
- Kerrick W. G., Zot H. G., Hoar P. E., Potter J. D. Evidence that the Sr2+ activation properties of cardiac troponin C are altered when substituted into skinned skeletal muscle fibers. J Biol Chem. 1985 Dec 15;260(29):15687–15693. [PubMed] [Google Scholar]
- Kretsinger R. H., Nockolds C. E. Carp muscle calcium-binding protein. II. Structure determination and general description. J Biol Chem. 1973 May 10;248(9):3313–3326. [PubMed] [Google Scholar]
- Laemmli U. K. Cleavage of structural proteins during the assembly of the head of bacteriophage T4. Nature. 1970 Aug 15;227(5259):680–685. doi: 10.1038/227680a0. [DOI] [PubMed] [Google Scholar]
- Morimoto S., Ohtsuki I. Effect of substitution of troponin C in cardiac myofibrils with skeletal troponin C or calmodulin on the Ca2+- and Sr2+-sensitive ATPase activity. J Biochem. 1988 Jul;104(1):149–154. doi: 10.1093/oxfordjournals.jbchem.a122412. [DOI] [PubMed] [Google Scholar]
- Moss R. L., Lauer M. R., Giulian G. G., Greaser M. L. Altered Ca2+ dependence of tension development in skinned skeletal muscle fibers following modification of troponin by partial substitution with cardiac troponin C. J Biol Chem. 1986 May 5;261(13):6096–6099. [PubMed] [Google Scholar]
- Persechini A., Kretsinger R. H. The central helix of calmodulin functions as a flexible tether. J Biol Chem. 1988 Sep 5;263(25):12175–12178. [PubMed] [Google Scholar]
- Persechini A., Moncrief N. D., Kretsinger R. H. The EF-hand family of calcium-modulated proteins. Trends Neurosci. 1989 Nov;12(11):462–467. doi: 10.1016/0166-2236(89)90097-0. [DOI] [PubMed] [Google Scholar]
- Potter J. D., Gergely J. The calcium and magnesium binding sites on troponin and their role in the regulation of myofibrillar adenosine triphosphatase. J Biol Chem. 1975 Jun 25;250(12):4628–4633. [PubMed] [Google Scholar]
- Putkey J. A., Draetta G. F., Slaughter G. R., Klee C. B., Cohen P., Stull J. T., Means A. R. Genetically engineered calmodulins differentially activate target enzymes. J Biol Chem. 1986 Jul 25;261(21):9896–9903. [PubMed] [Google Scholar]
- Putkey J. A., Ono T., VanBerkum M. F., Means A. R. Functional significance of the central helix in calmodulin. J Biol Chem. 1988 Aug 15;263(23):11242–11249. [PubMed] [Google Scholar]
- Putkey J. A., Slaughter G. R., Means A. R. Bacterial expression and characterization of proteins derived from the chicken calmodulin cDNA and a calmodulin processed gene. J Biol Chem. 1985 Apr 25;260(8):4704–4712. [PubMed] [Google Scholar]
- Putkey J. A., Sweeney H. L., Campbell S. T. Site-directed mutation of the trigger calcium-binding sites in cardiac troponin C. J Biol Chem. 1989 Jul 25;264(21):12370–12378. [PubMed] [Google Scholar]
- Reinach F. C., Karlsson R. Cloning, expression, and site-directed mutagenesis of chicken skeletal muscle troponin C. J Biol Chem. 1988 Feb 15;263(5):2371–2376. [PubMed] [Google Scholar]
- Robertson S. P., Johnson J. D., Potter J. D. The time-course of Ca2+ exchange with calmodulin, troponin, parvalbumin, and myosin in response to transient increases in Ca2+. Biophys J. 1981 Jun;34(3):559–569. doi: 10.1016/S0006-3495(81)84868-0. [DOI] [PMC free article] [PubMed] [Google Scholar]
- Xu G. Q., Hitchcock-DeGregori S. E. Synthesis of a troponin C cDNA and expression of wild-type and mutant proteins in Escherichia coli. J Biol Chem. 1988 Sep 25;263(27):13962–13969. [PubMed] [Google Scholar]
- Zot H. G., Potter J. D. A structural role for the Ca2+-Mg2+ sites on troponin C in the regulation of muscle contraction. Preparation and properties of troponin C depleted myofibrils. J Biol Chem. 1982 Jul 10;257(13):7678–7683. [PubMed] [Google Scholar]
- van Eerd J. P., Takahashi K. The amino acid sequence of bovine cardiac tamponin-C. Comparison with rabbit skeletal troponin-C. Biochem Biophys Res Commun. 1975 May 5;64(1):122–127. doi: 10.1016/0006-291x(75)90227-2. [DOI] [PubMed] [Google Scholar]
- van Eerd J. P., Takahshi K. Determination of the complete amino acid sequence of bovine cardiac troponin C. Biochemistry. 1976 Mar 9;15(5):1171–1180. doi: 10.1021/bi00650a033. [DOI] [PubMed] [Google Scholar]