Abstract
DNA fragments encoding a red light-inducible phycocyanin gene and a green light-inducible phycoerythrin gene have been used to investigate the effect of red and green pulses on the accumulation of phycocyanin and phycoerythrin mRNA in subsequent darkness. A red pulse promotes phycocyanin and suppresses phycoerythrin mRNA accumulation while a green pulse has an opposite effect on both transcript levels. The effect of a saturating light pulse is canceled by a subsequently given pulse of the other light quality. For a given mRNA, the positive and negative effects require the same fluence for saturation, whereas to saturate the phycoerythrin mRNA response requires at least twice as much light as to saturate the phycocyanin mRNA response. Calculations of the apparent extinction coefficients for the pigments mediating the light-regulated mRNA increase and decrease are of the order of 2 × 104 for phycocyanin mRNA and less than 104 for phycoerythrin mRNA. The data are consistent with the hypothesis that the light-induced increase and decrease of a particular phycobiliprotein mRNA is controlled by a single red/green photoreversible photosystem, but that phycoerythrin and phycocyanin mRNA levels are either controlled by two distinct photoreversible systems or that marked differences occur in the chain of events leading from photoperception to gene activation. These system(s) differ from most phytochrome systems in several ways: First, they remain fully on or off depending upon the light quality of the terminal irradiation. Second, they can be completely reversed by light of the appropriate wavelength after several hours of darkness without diminution of the effectiveness of the reversing light pulse. These two features argue against the existence of dark reversion or dark destruction of the biologically active moiety. Third, signal transduction is rapid—measurable mRNA changes occur even during a 10 minute irradiation.
Full text
PDF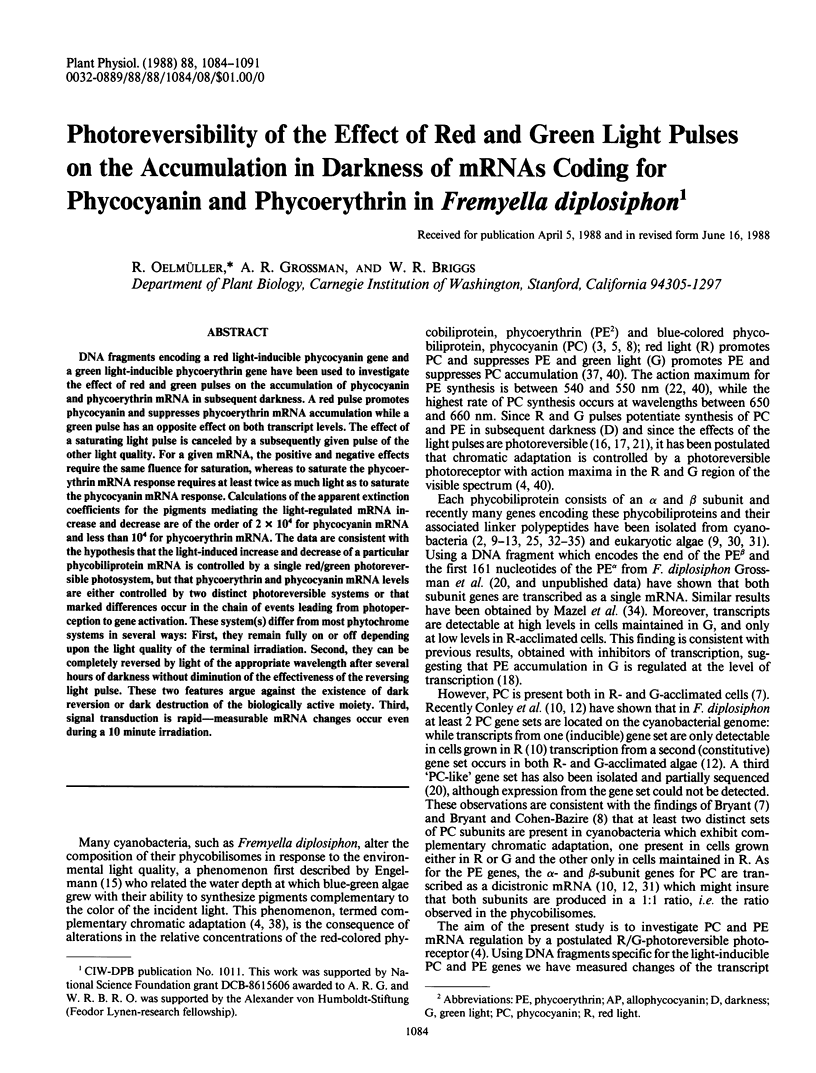
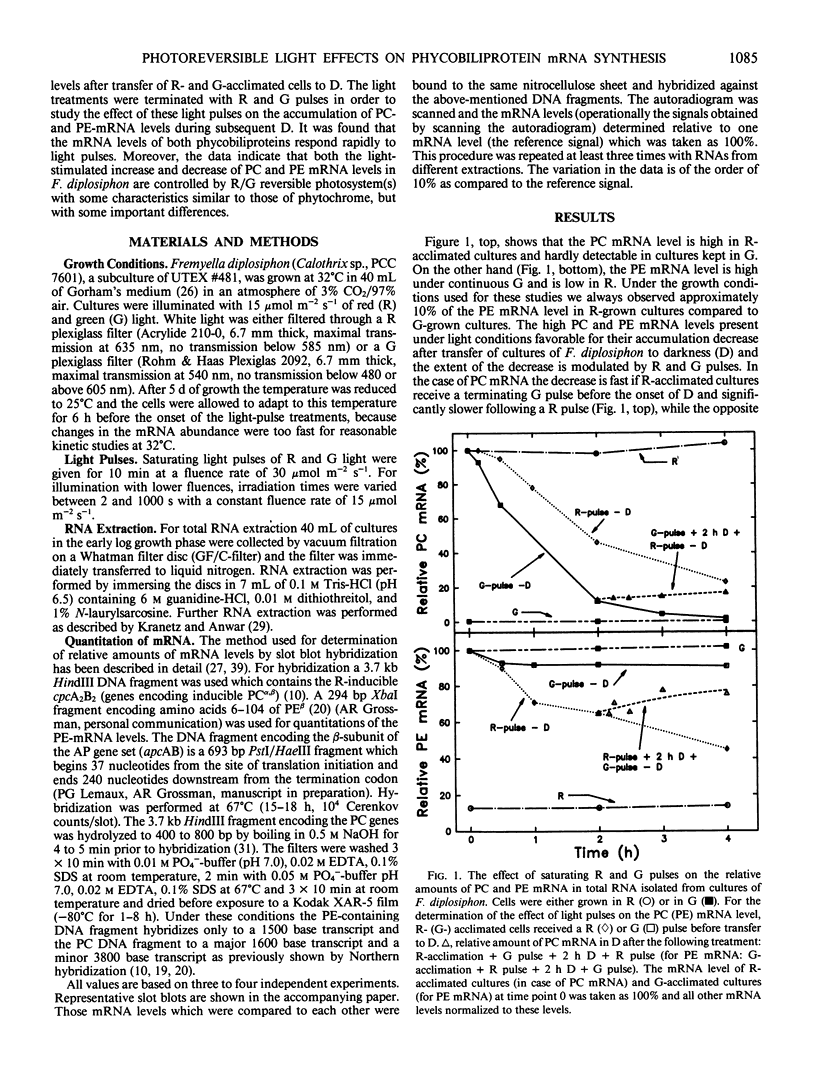
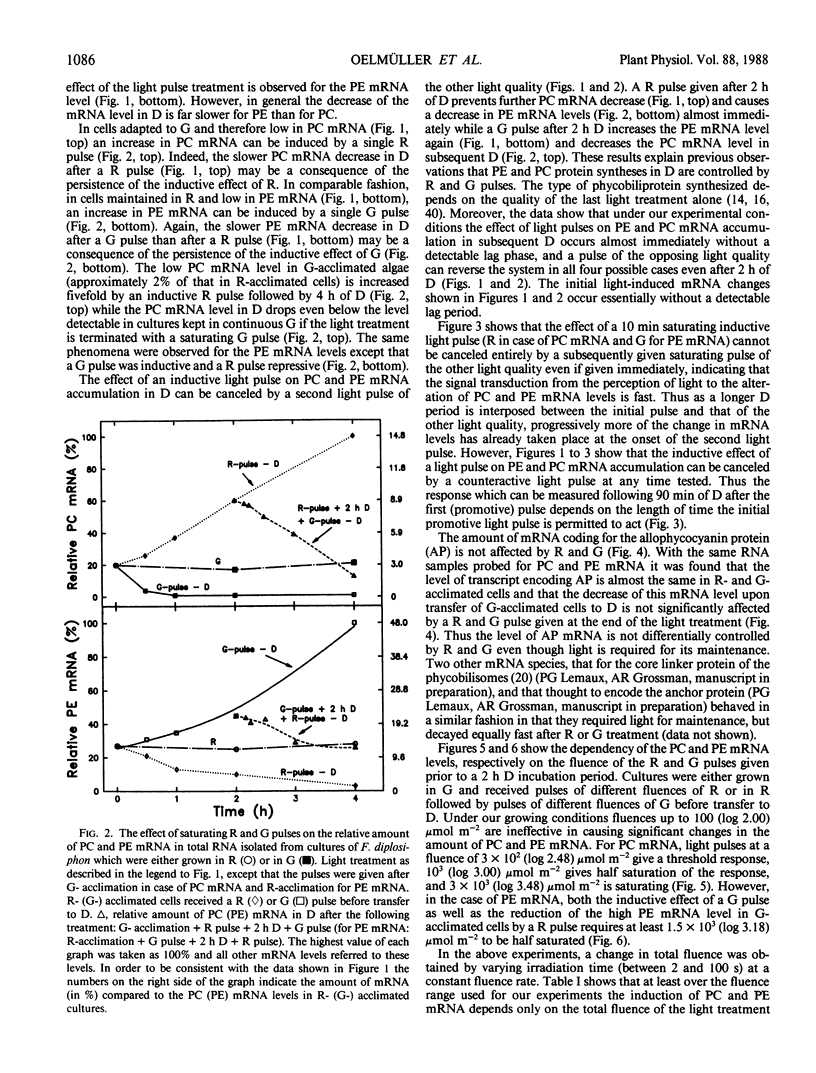
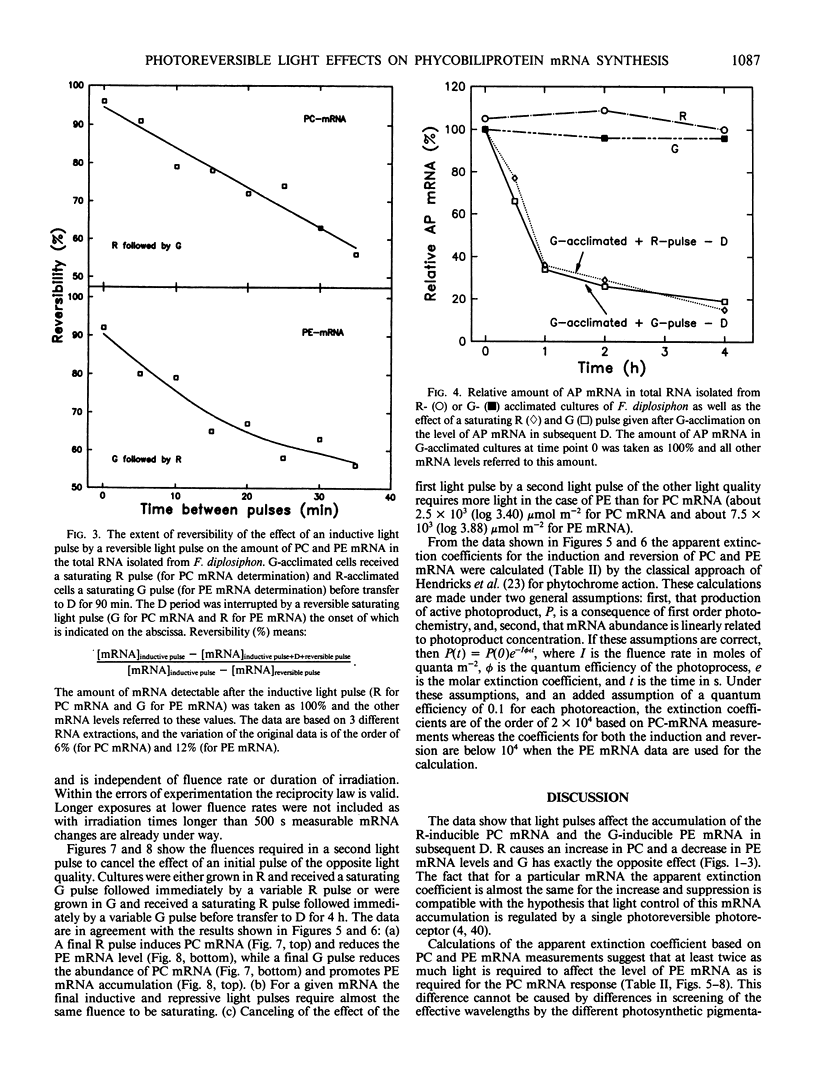
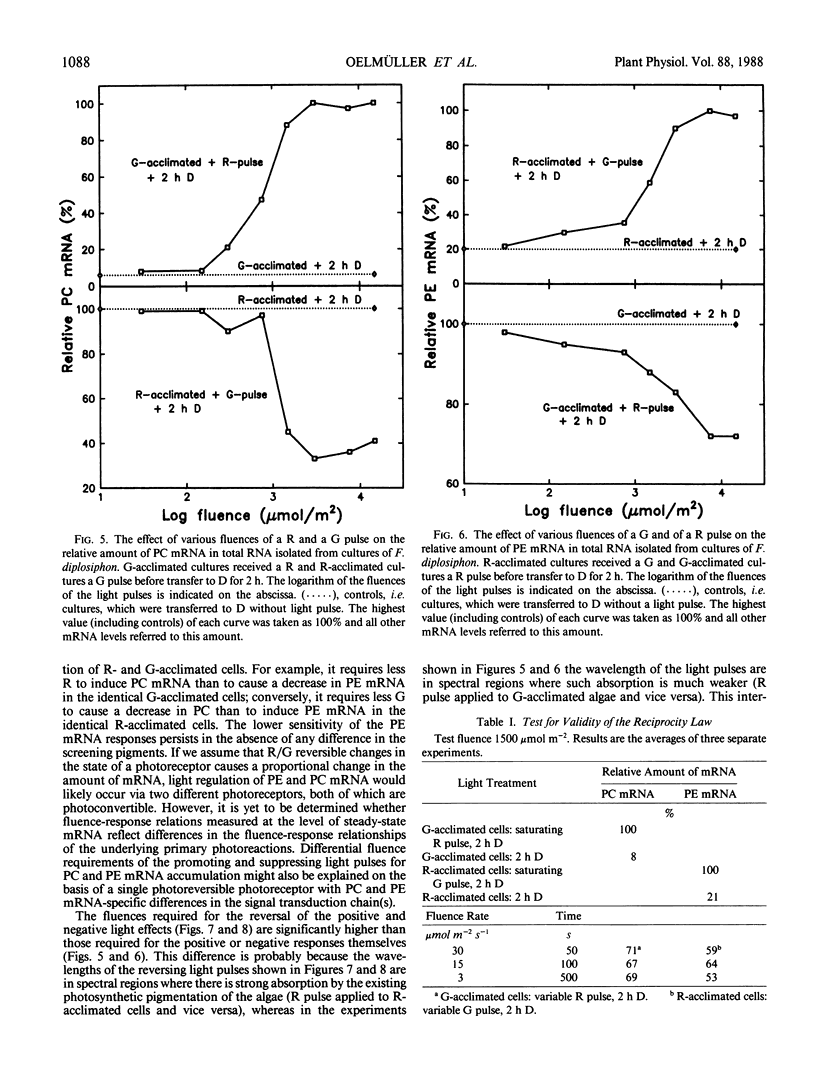
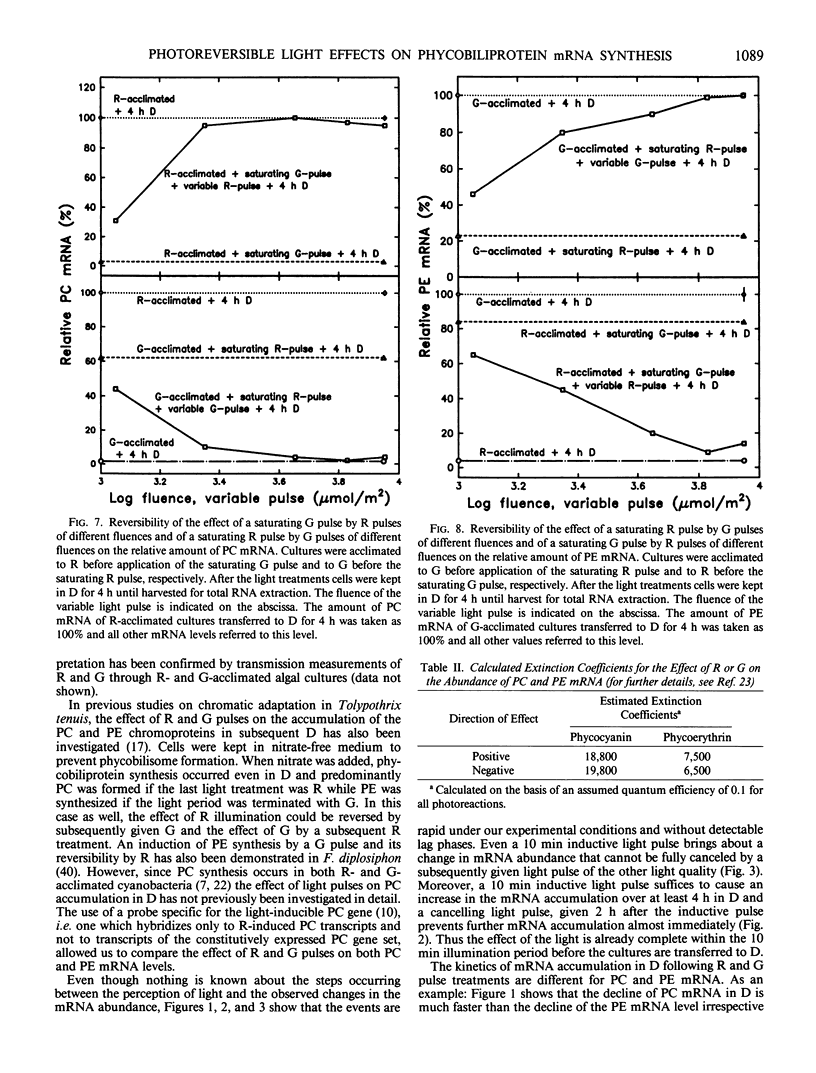
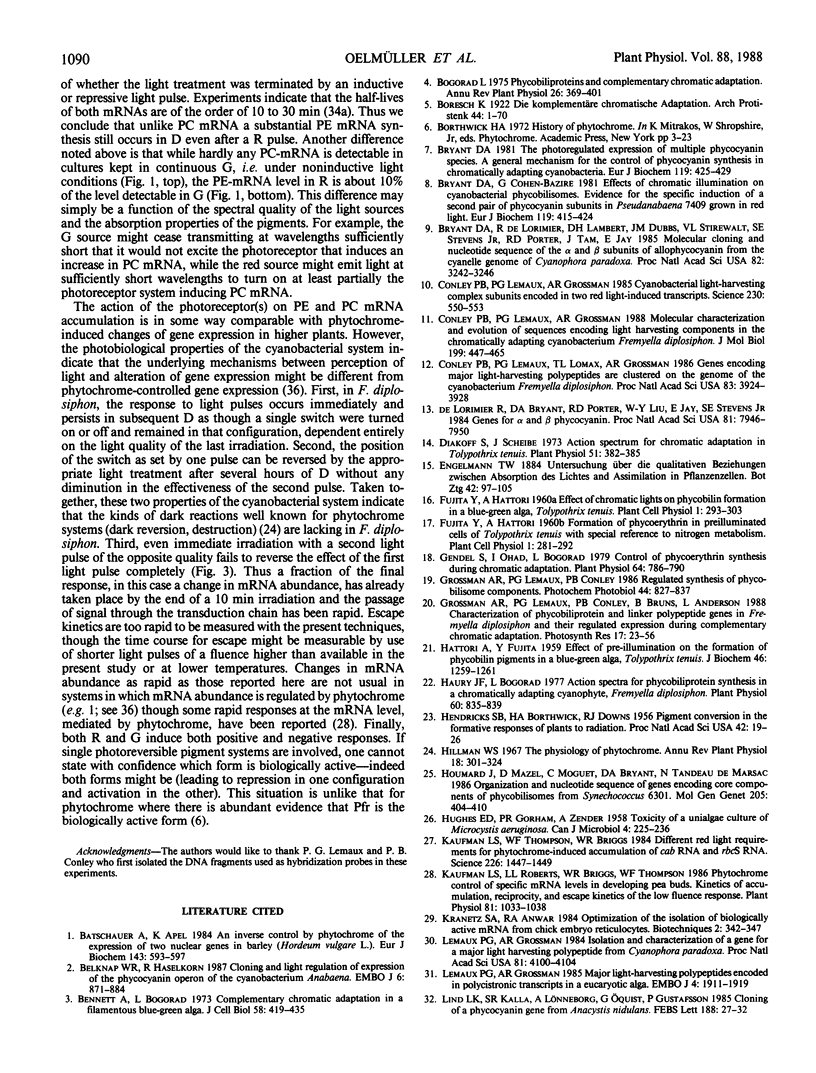
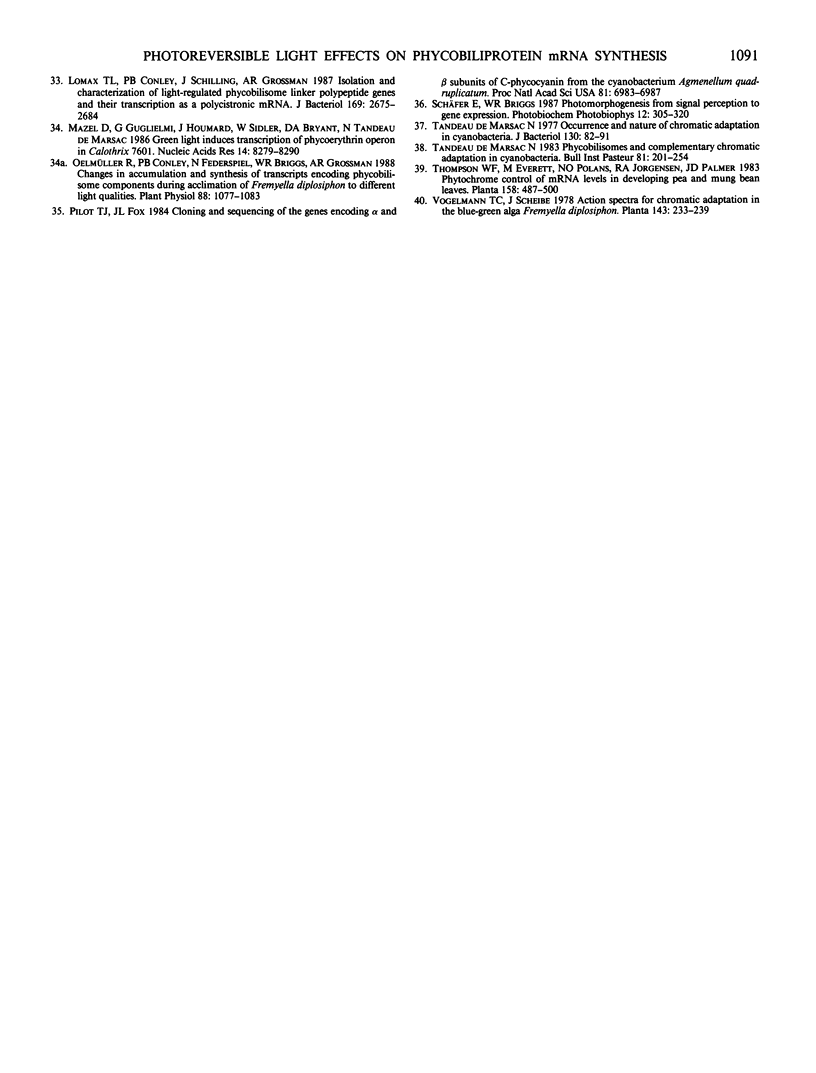
Selected References
These references are in PubMed. This may not be the complete list of references from this article.
- Batschauer A., Apel K. An inverse control by phytochrome of the expression of two nuclear genes in barley (Hordeum vulgare L.). Eur J Biochem. 1984 Sep 17;143(3):593–597. doi: 10.1111/j.1432-1033.1984.tb08411.x. [DOI] [PubMed] [Google Scholar]
- Belknap W. R., Haselkorn R. Cloning and light regulation of expression of the phycocyanin operon of the cyanobacterium Anabaena. EMBO J. 1987 Apr;6(4):871–884. doi: 10.1002/j.1460-2075.1987.tb04833.x. [DOI] [PMC free article] [PubMed] [Google Scholar]
- Bennett A., Bogorad L. Complementary chromatic adaptation in a filamentous blue-green alga. J Cell Biol. 1973 Aug;58(2):419–435. doi: 10.1083/jcb.58.2.419. [DOI] [PMC free article] [PubMed] [Google Scholar]
- Bryant D. A., Cohen-Bazire G. Effects of chromatic illumination on cyanobacterial phycobilisomes. Evidence for the specific induction of a second pair of phycocyanin subunits in Pseudanabaena 7409 grown in red light. Eur J Biochem. 1981 Oct;119(2):415–424. doi: 10.1111/j.1432-1033.1981.tb05624.x. [DOI] [PubMed] [Google Scholar]
- Bryant D. A. The photoregulated expression of multiple phycocyanin species. A general mechanism for the control of phycocyanin synthesis in chromatically adapting cyanobacteria. Eur J Biochem. 1981 Oct;119(2):425–429. doi: 10.1111/j.1432-1033.1981.tb05625.x. [DOI] [PubMed] [Google Scholar]
- Bryant D. A., de Lorimier R., Lambert D. H., Dubbs J. M., Stirewalt V. L., Stevens S. E., Jr, Porter R. D., Tam J., Jay E. Molecular cloning and nucleotide sequence of the alpha and beta subunits of allophycocyanin from the cyanelle genome of Cyanophora paradoxa. Proc Natl Acad Sci U S A. 1985 May;82(10):3242–3246. doi: 10.1073/pnas.82.10.3242. [DOI] [PMC free article] [PubMed] [Google Scholar]
- Conley P. B., Lemaux P. G., Grossman A. R. Cyanobacterial light-harvesting complex subunits encoded in two red light-induced transcripts. Science. 1985 Nov 1;230(4725):550–553. doi: 10.1126/science.3931221. [DOI] [PubMed] [Google Scholar]
- Conley P. B., Lemaux P. G., Grossman A. Molecular characterization and evolution of sequences encoding light-harvesting components in the chromatically adapting cyanobacterium Fremyella diplosiphon. J Mol Biol. 1988 Feb 5;199(3):447–465. doi: 10.1016/0022-2836(88)90617-1. [DOI] [PubMed] [Google Scholar]
- Conley P. B., Lemaux P. G., Lomax T. L., Grossman A. R. Genes encoding major light-harvesting polypeptides are clustered on the genome of the cyanobacterium Fremyella diplosiphon. Proc Natl Acad Sci U S A. 1986 Jun;83(11):3924–3928. doi: 10.1073/pnas.83.11.3924. [DOI] [PMC free article] [PubMed] [Google Scholar]
- Diakoff S., Scheibe J. Action Spectra for Chromatic Adaptation in Tolypothrix tenuis. Plant Physiol. 1973 Feb;51(2):382–385. doi: 10.1104/pp.51.2.382. [DOI] [PMC free article] [PubMed] [Google Scholar]
- Gendel S., Ohad I., Bogorad L. Control of Phycoerythrin Synthesis during Chromatic Adaptation. Plant Physiol. 1979 Nov;64(5):786–790. doi: 10.1104/pp.64.5.786. [DOI] [PMC free article] [PubMed] [Google Scholar]
- Grossman A. R., Lemaux P. G., Conley P. B. Regulated synthesis of phycobilisome components. Photochem Photobiol. 1986 Dec;44(6):827–837. doi: 10.1111/j.1751-1097.1986.tb05543.x. [DOI] [PubMed] [Google Scholar]
- HUGHES E. O., GORHAM P. R., ZEHNDER A. Toxicity of a unialgal culture of Microcystis aeruginosa. Can J Microbiol. 1958 Jun;4(3):225–236. doi: 10.1139/m58-024. [DOI] [PubMed] [Google Scholar]
- Haury J. F., Bogorad L. Action Spectra for Phycobiliprotein Synthesis in a Chromatically Adapting Cyanophyte, Fremyella diplosiphon. Plant Physiol. 1977 Dec;60(6):835–839. doi: 10.1104/pp.60.6.835. [DOI] [PMC free article] [PubMed] [Google Scholar]
- Hendricks S. B., Borthwick H. A., Downs R. J. PIGMENT CONVERSION IN THE FORMATIVE RESPONSES OF PLANTS TO RADIATION. Proc Natl Acad Sci U S A. 1956 Jan;42(1):19–26. doi: 10.1073/pnas.42.1.19. [DOI] [PMC free article] [PubMed] [Google Scholar]
- Houmard J., Mazel D., Moguet C., Bryant D. A., Tandeau de Marsac N. Organization and nucleotide sequence of genes encoding core components of the phycobilisomes from Synechococcus 6301. Mol Gen Genet. 1986 Dec;205(3):404–410. doi: 10.1007/BF00338074. [DOI] [PubMed] [Google Scholar]
- Kaufman L. S., Roberts L. L., Briggs W. R., Thompson W. F. Phytochrome Control of Specific mRNA levels in Developing Pea Buds : Kinetics of Accumulation, Reciprocity, and Escape Kinetics of the Low Fluence Response. Plant Physiol. 1986 Aug;81(4):1033–1038. doi: 10.1104/pp.81.4.1033. [DOI] [PMC free article] [PubMed] [Google Scholar]
- Kaufman L. S., Thompson W. F., Briggs W. R. Different Red Light Requirements for Phytochrome-Induced Accumulation of cab RNA and rbcS RNA. Science. 1984 Dec 21;226(4681):1447–1449. doi: 10.1126/science.226.4681.1447. [DOI] [PubMed] [Google Scholar]
- Lemaux P. G., Grossman A. R. Major light-harvesting polypeptides encoded in polycistronic transcripts in a eukaryotic alga. EMBO J. 1985 Aug;4(8):1911–1919. doi: 10.1002/j.1460-2075.1985.tb03870.x. [DOI] [PMC free article] [PubMed] [Google Scholar]
- Lemaux P. G., Grossman A. Isolation and characterization of a gene for a major light-harvesting polypeptide from Cyanophora paradoxa. Proc Natl Acad Sci U S A. 1984 Jul;81(13):4100–4104. doi: 10.1073/pnas.81.13.4100. [DOI] [PMC free article] [PubMed] [Google Scholar]
- Lomax T. L., Conley P. B., Schilling J., Grossman A. R. Isolation and characterization of light-regulated phycobilisome linker polypeptide genes and their transcription as a polycistronic mRNA. J Bacteriol. 1987 Jun;169(6):2675–2684. doi: 10.1128/jb.169.6.2675-2684.1987. [DOI] [PMC free article] [PubMed] [Google Scholar]
- Mazel D., Guglielmi G., Houmard J., Sidler W., Bryant D. A., Tandeau de Marsac N. Green light induces transcription of the phycoerythrin operon in the cyanobacterium Calothrix 7601. Nucleic Acids Res. 1986 Nov 11;14(21):8279–8290. doi: 10.1093/nar/14.21.8279. [DOI] [PMC free article] [PubMed] [Google Scholar]
- Oelmüller R., Conley P. B., Federspiel N., Briggs W. R., Grossman A. R. Changes in Accumulation and Synthesis of Transcripts Encoding Phycobilisome Components during Acclimation of Fremyella diplosiphon to Different Light Qualities. Plant Physiol. 1988 Dec;88(4):1077–1083. doi: 10.1104/pp.88.4.1077. [DOI] [PMC free article] [PubMed] [Google Scholar]
- Pilot T. J., Fox J. L. Cloning and sequencing of the genes encoding the alpha and beta subunits of C-phycocyanin from the cyanobacterium Agmenellum quadruplicatum. Proc Natl Acad Sci U S A. 1984 Nov;81(22):6983–6987. doi: 10.1073/pnas.81.22.6983. [DOI] [PMC free article] [PubMed] [Google Scholar]
- Tandeau de Marsac N. Occurrence and nature of chromatic adaptation in cyanobacteria. J Bacteriol. 1977 Apr;130(1):82–91. doi: 10.1128/jb.130.1.82-91.1977. [DOI] [PMC free article] [PubMed] [Google Scholar]
- de Lorimier R., Bryant D. A., Porter R. D., Liu W. Y., Jay E., Stevens S. E., Jr Genes for the alpha and beta subunits of phycocyanin. Proc Natl Acad Sci U S A. 1984 Dec;81(24):7946–7950. doi: 10.1073/pnas.81.24.7946. [DOI] [PMC free article] [PubMed] [Google Scholar]