Abstract
Lipid and fatty acid analyses were performed on whole leaf extracts and isolated thylakoids from winter rye (Secale cereale L. cv Puma) grown at 5°C cold-hardened rye (RH) and 20°C nonhardened rye (RNH). Although no significant change in total lipid content was observed, growth at low, cold-hardening temperature resulted in a specific 67% (thylakoids) to 74% (whole leaves) decrease in the trans-Δ3-hexadecenoic acid (trans-16:1) level associated with phosphatidyldiacylglycerol (PG). Electron spin resonance and differential scanning calorimetry (DSC) indicated no significant difference in the fluidity of RH and RNH thylakoids. Separation of chlorophyll-protein complexes by sodium dodecyl sulfate-polyacrylamide gel electrophoresis indicated that the ratio of oligomeric light harvesting complex:monomeric light harvesting complex (LHCII1:LHCII3) was 2-fold higher in RNH than RH thylakoids. The ratio of CP1a:CP1 was also 1.5-fold higher in RNH than RH thylakoids. Analyses of winter rye grown at 20, 15, 10, and 5°C indicated that both, the trans-16:1 acid levels in PG and the LHCII1:LHCII3 decreased concomitantly with a decrease in growth temperature. Above 40°C, differential scanning calorimetry of RNH thylakoids indicated the presence of five major endotherms (47, 60, 67, 73, and 86°C). Although the general features of the temperature transitions observed above 40°C in RH thylakoids were similar to those observed for RNH thylakoids, the transitions at 60 and 73°C were resolved as inflections only and RH thylakoids exhibited transitions at 45 and 84°C which were 2°C lower than those observed in RNH thylakoids. Since polypeptide and lipid compositions of RH and RNH thylakoids were very similar, we suggest that these differences reflect alterations in thylakoid membrane organization. Specifically, it is suggested that low developmental temperature modulates LHCII organization such that oligomeric LHCII predominates in RNH thylakoids whereas a monomeric or an intermediate form of LHCII predominates in RH thylakoids. Furthermore, we conclude that low developmental temperature modulates LHCII organization by specifically altering the fatty composition of thylakoid PG.
Full text
PDF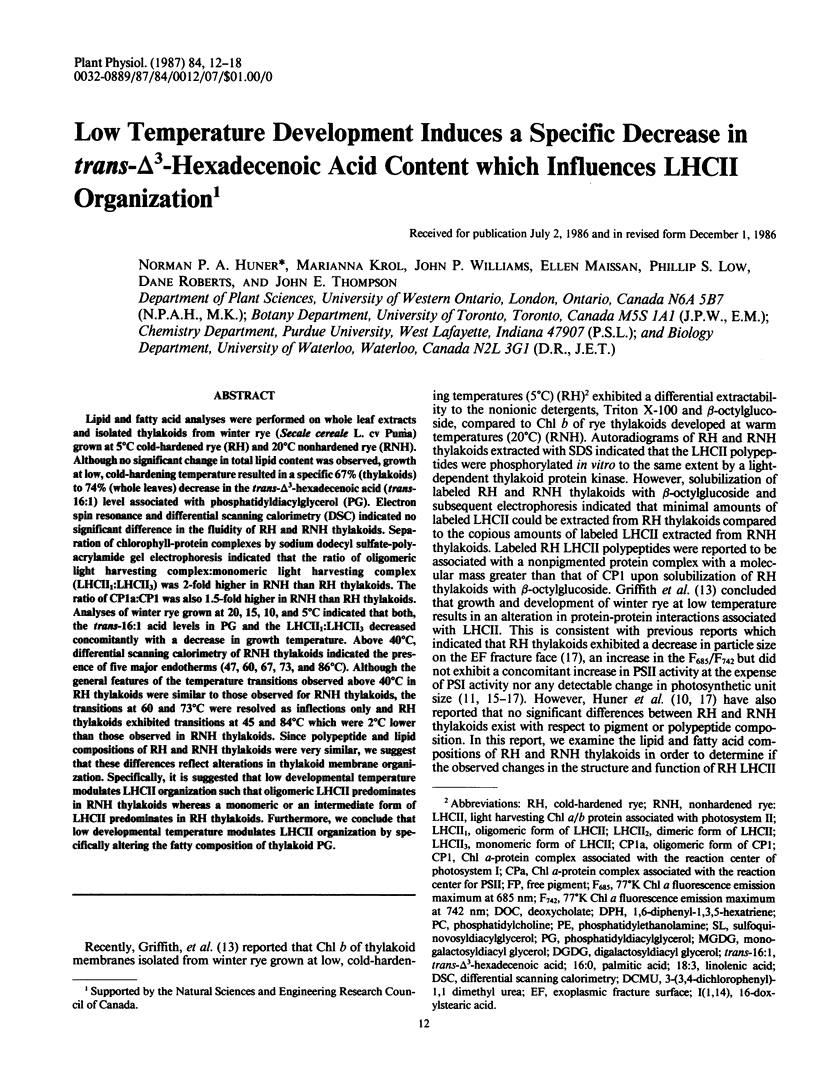
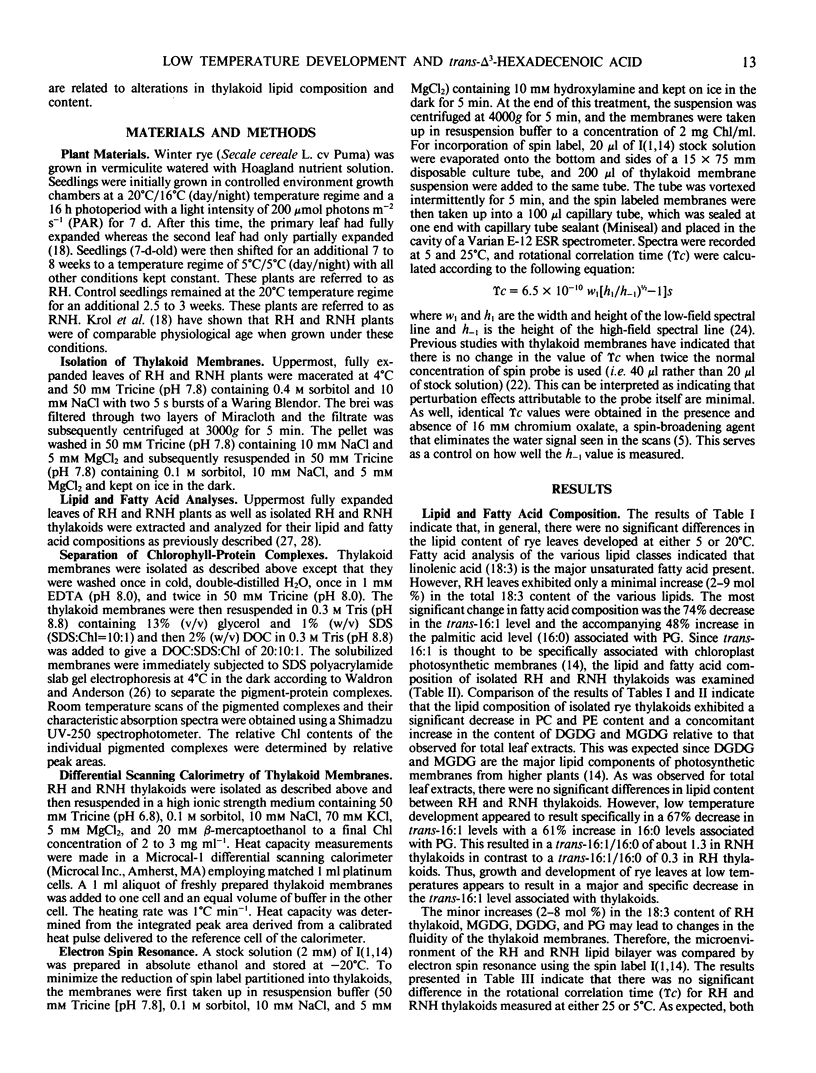
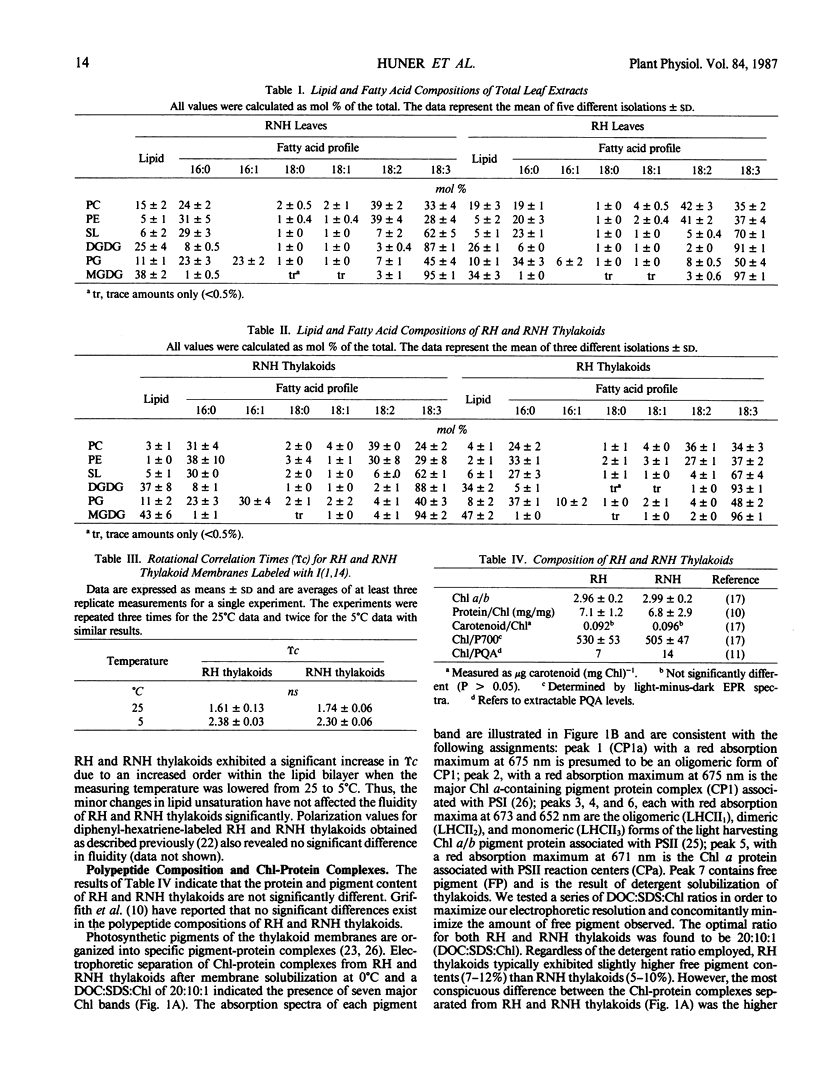
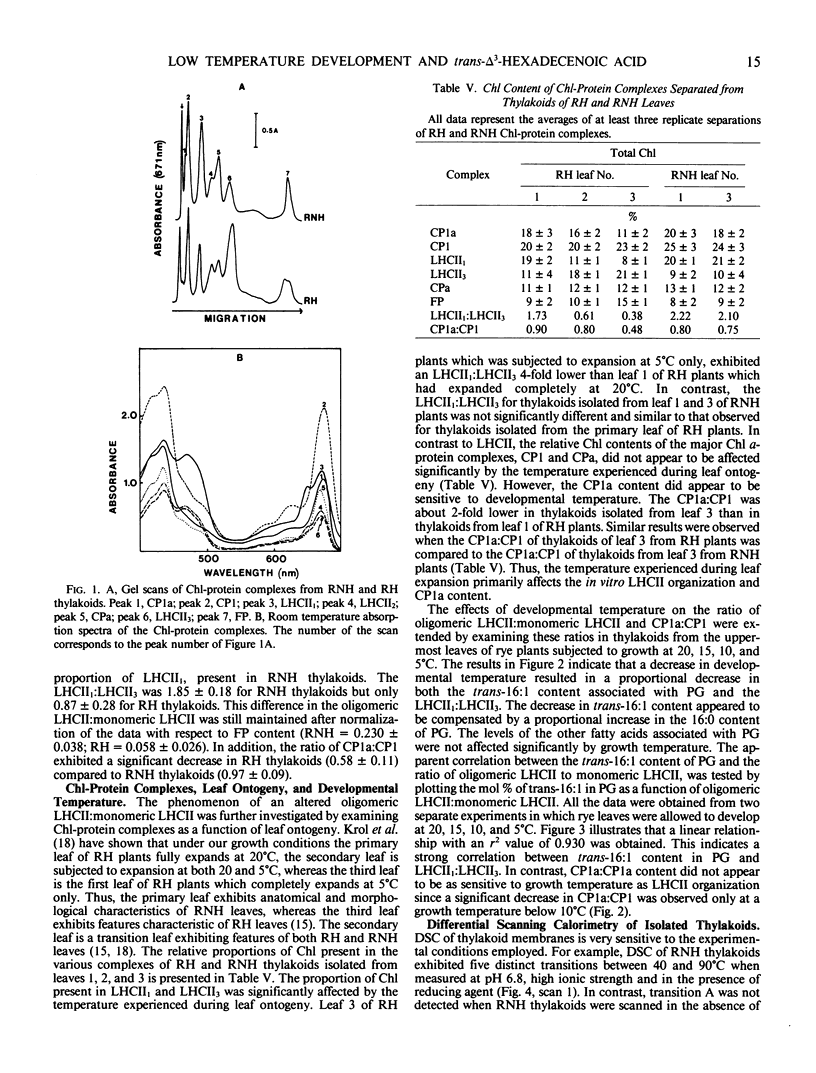
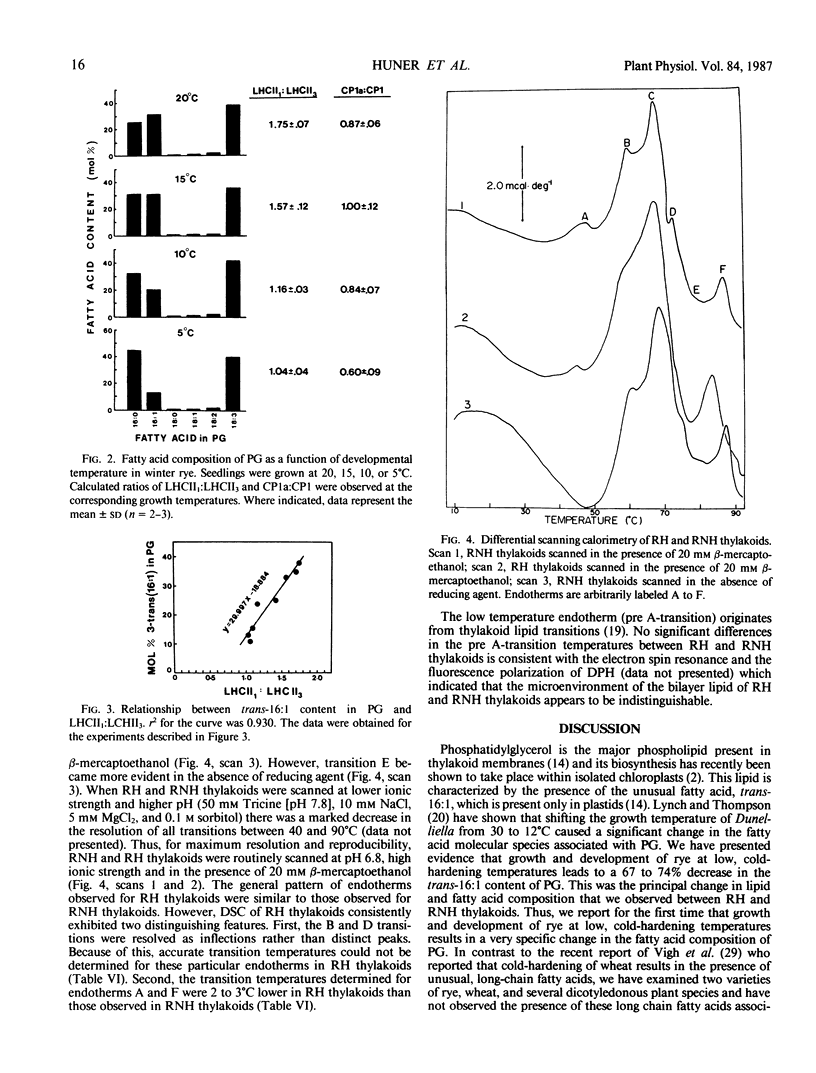
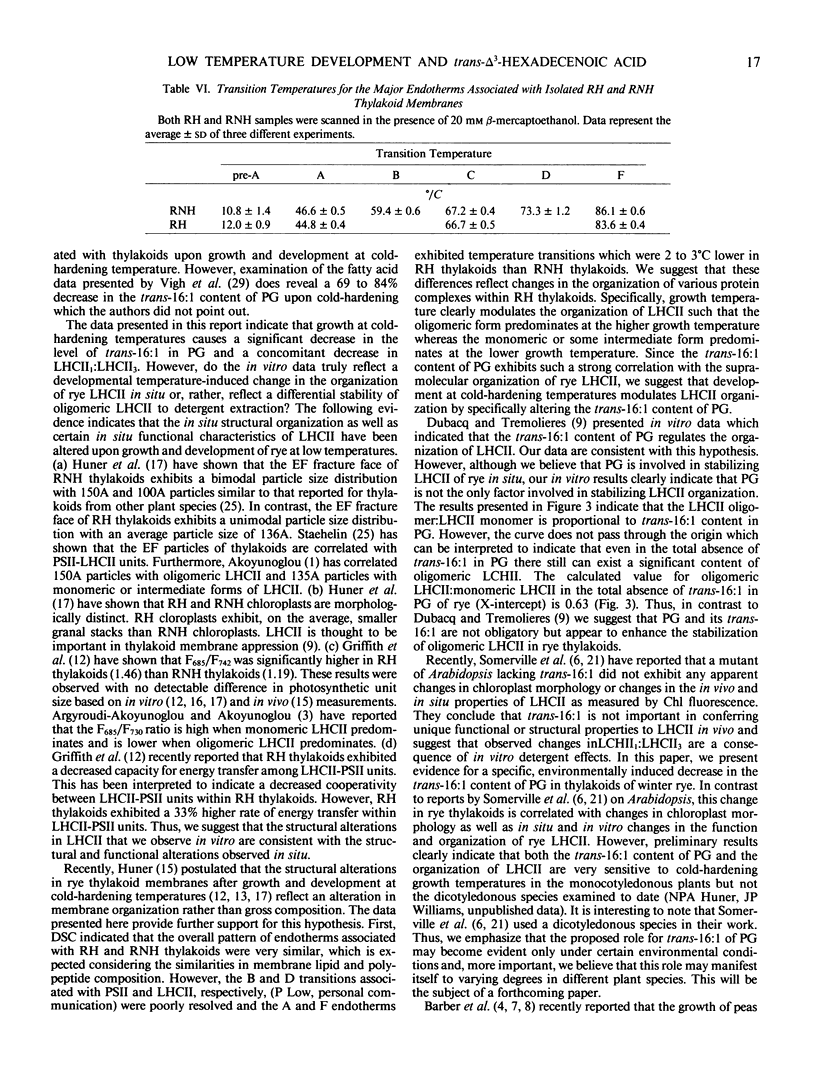
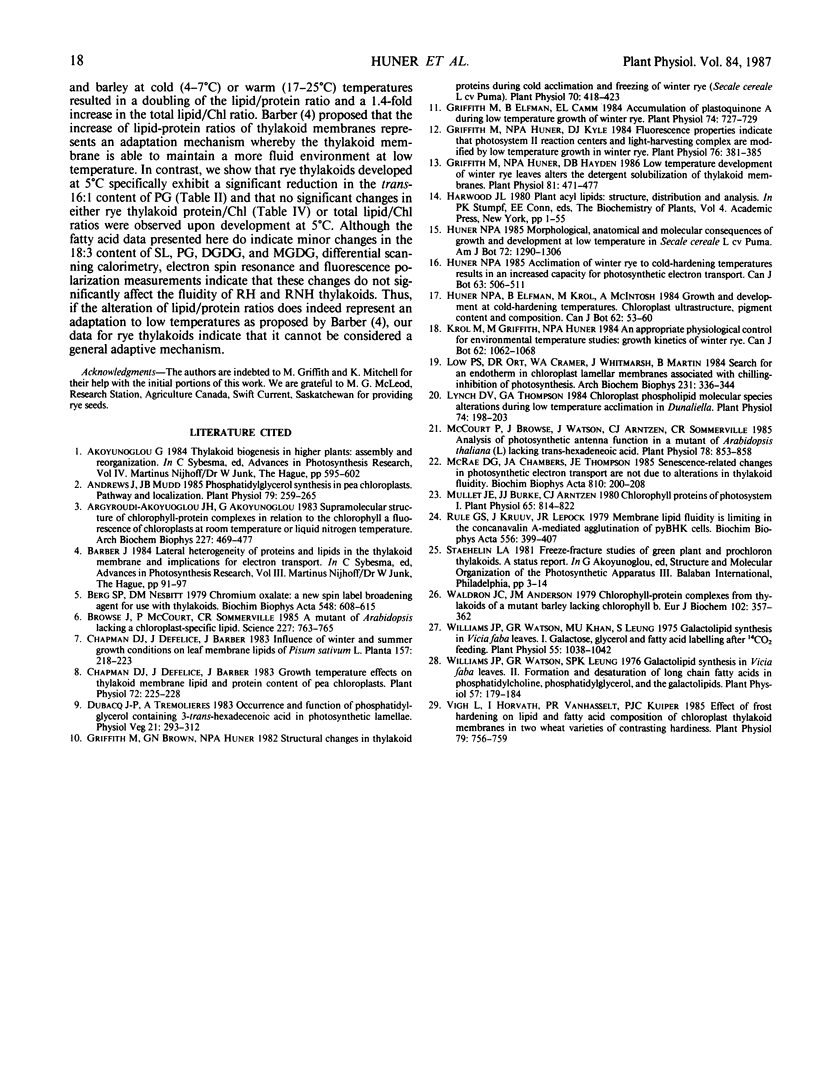
Selected References
These references are in PubMed. This may not be the complete list of references from this article.
- Andrews J., Mudd J. B. Phosphatidylglycerol synthesis in pea chloroplasts: pathway and localization. Plant Physiol. 1985 Sep;79(1):259–265. doi: 10.1104/pp.79.1.259. [DOI] [PMC free article] [PubMed] [Google Scholar]
- Argyroudi A. J., Akoyunoglou G. Supramolecular structure of chlorophyll-protein complexes in relation to the chlorophyll a fluorescence of chloroplasts at room or liquid nitrogen temperature. Arch Biochem Biophys. 1983 Dec;227(2):469–477. doi: 10.1016/0003-9861(83)90477-0. [DOI] [PubMed] [Google Scholar]
- Berg S. P., Nesbitt D. M. Chromium oxalate: a new spin label broadening agent for use with thylakoids. Biochim Biophys Acta. 1979 Dec 6;548(3):608–615. doi: 10.1016/0005-2728(79)90068-9. [DOI] [PubMed] [Google Scholar]
- Browse J., McCourt P., Somerville C. R. A mutant of Arabidopsis lacking a chloroplast-specific lipid. Science. 1985 Feb 15;227(4688):763–765. doi: 10.1126/science.227.4688.763. [DOI] [PubMed] [Google Scholar]
- Chapman D. J., De-Felice J., Barber J. Growth temperature effects on thylakoid membrane lipid and protein content of pea chloroplasts. Plant Physiol. 1983 May;72(1):225–228. doi: 10.1104/pp.72.1.225. [DOI] [PMC free article] [PubMed] [Google Scholar]
- Griffith M., Brown G. N., Huner N. P. Structural Changes in Thylakoid Proteins during Cold Acclimation and Freezing of Winter Rye (Secale cereale L. cv. Puma). Plant Physiol. 1982 Aug;70(2):418–423. doi: 10.1104/pp.70.2.418. [DOI] [PMC free article] [PubMed] [Google Scholar]
- Griffith M., Elfman B., Camm E. L. Accumulation of Plastoquinone A during Low Temperature Growth of Winter Rye. Plant Physiol. 1984 Mar;74(3):727–729. doi: 10.1104/pp.74.3.727. [DOI] [PMC free article] [PubMed] [Google Scholar]
- Griffith M., Huner N. P., Hayden D. B. Low temperature development of winter rye leaves alters the detergent solubilization of thylakoid membranes. Plant Physiol. 1986 Jun;81(2):471–477. doi: 10.1104/pp.81.2.471. [DOI] [PMC free article] [PubMed] [Google Scholar]
- Griffith M., Huner N. P., Kyle D. J. Fluorescence Properties Indicate that Photosystem II Reaction Centers and Light-Harvesting Complex Are Modified by Low Temperature Growth in Winter Rye. Plant Physiol. 1984 Oct;76(2):381–385. doi: 10.1104/pp.76.2.381. [DOI] [PMC free article] [PubMed] [Google Scholar]
- Low P. S., Ort D. R., Cramer W. A., Whitmarsh J., Martin B. Search for an endotherm in chloroplast lamellar membranes associated with chilling-inhibition of photosynthesis. Arch Biochem Biophys. 1984 Jun;231(2):336–344. doi: 10.1016/0003-9861(84)90396-5. [DOI] [PubMed] [Google Scholar]
- Lynch D. V., Thompson G. A. Chloroplast Phospholipid Molecular Species Alterations during Low Temperature Acclimation in Dunaliella. Plant Physiol. 1984 Feb;74(2):198–203. doi: 10.1104/pp.74.2.198. [DOI] [PMC free article] [PubMed] [Google Scholar]
- McCourt P., Browse J., Watson J., Arntzen C. J., Somerville C. R. Analysis of Photosynthetic Antenna Function in a Mutant of Arabidopsis thaliana (L.) Lacking trans-Hexadecenoic Acid. Plant Physiol. 1985 Aug;78(4):853–858. doi: 10.1104/pp.78.4.853. [DOI] [PMC free article] [PubMed] [Google Scholar]
- Mullet J. E., Burke J. J., Arntzen C. J. Chlorophyll proteins of photosystem I. Plant Physiol. 1980 May;65(5):814–822. doi: 10.1104/pp.65.5.814. [DOI] [PMC free article] [PubMed] [Google Scholar]
- Rule G. S., Kruuv J., Lepock J. R. Membrane lipid fluidity as rate limiting in the concanavalin A-mediated agglutination of pyBHK cells. Biochim Biophys Acta. 1979 Oct 5;556(3):399–407. doi: 10.1016/0005-2736(79)90128-7. [DOI] [PubMed] [Google Scholar]
- Vigh L., Horvàth I., van Hasselt P. R., Kuiper P. J. Effect of frost hardening on lipid and Fatty Acid composition of chloroplast thylakoid membranes in two wheat varieties of contrasting hardiness. Plant Physiol. 1985 Nov;79(3):756–759. doi: 10.1104/pp.79.3.756. [DOI] [PMC free article] [PubMed] [Google Scholar]
- Waldron J. C., Anderson J. M. Chlorophyll-protein complexes from thylakoids of a mutant barley lacking chlorophyll b. Eur J Biochem. 1979 Dec 17;102(2):357–362. doi: 10.1111/j.1432-1033.1979.tb04250.x. [DOI] [PubMed] [Google Scholar]
- Williams J. P., Watson G. R., Khan M. U., Leung S. Galactolipid Synthesis in Vicia faba Leaves: I. Galactose, Glycerol, and Fatty Acid Labeling after CO(2) Feeding. Plant Physiol. 1975 Jun;55(6):1038–1042. doi: 10.1104/pp.55.6.1038. [DOI] [PMC free article] [PubMed] [Google Scholar]
- Williams J. P., Watson G. R., Leung S. P. Galactolipid Synthesis in Vicia faba Leaves: II. Formation and Desaturation of Long Chain Fatty Acids in Phosphatidylcholine, Phosphatidylglycerol, and the Galactolipids. Plant Physiol. 1976 Feb;57(2):179–184. doi: 10.1104/pp.57.2.179. [DOI] [PMC free article] [PubMed] [Google Scholar]