Abstract
Heat shock of living tissue induces the synthesis of a unique group of proteins, the heat shock proteins. In plants, the major group of heat shock proteins has a molecular mass of 15 to 25 kilodaltons. Accumulation of these proteins to stainable levels has been reported in only a few species. To examine accumulation of the low molecular weight heat shock proteins in a broader range of species, two-dimensional electrophoresis was used to resolve total protein from the following species: soybean (Glycine max L. Merr., var Wayne), pea (Pisum sativum L., var Early Alaska), sunflower (Helianthus annuus L.), wheat (Triticum aestivum L.), rice (Oryza sativa L., cv IR-36), maize (Zea mays L.), pearl millet (Pennisetum americanum L. Leeke, line 23DB), and Panicum miliaceum L. When identified by both silver staining and incorporation of radiolabel, a diverse array of low molecular weight heat shock proteins was synthesized in each of these species. These proteins accumulated to significant levels after three hours of heat shock but exhibited considerable heterogeneity in isoelectric point, molecular weight, stainability, and radiolabel incorporation. Although most appeared to be synthesized only during heat shock, some were detectable at low levels in control tissue. Compared to the monocots, a higher proportion of low molecular weight heat shock proteins was detectable in control tissues from dicots.
Full text
PDF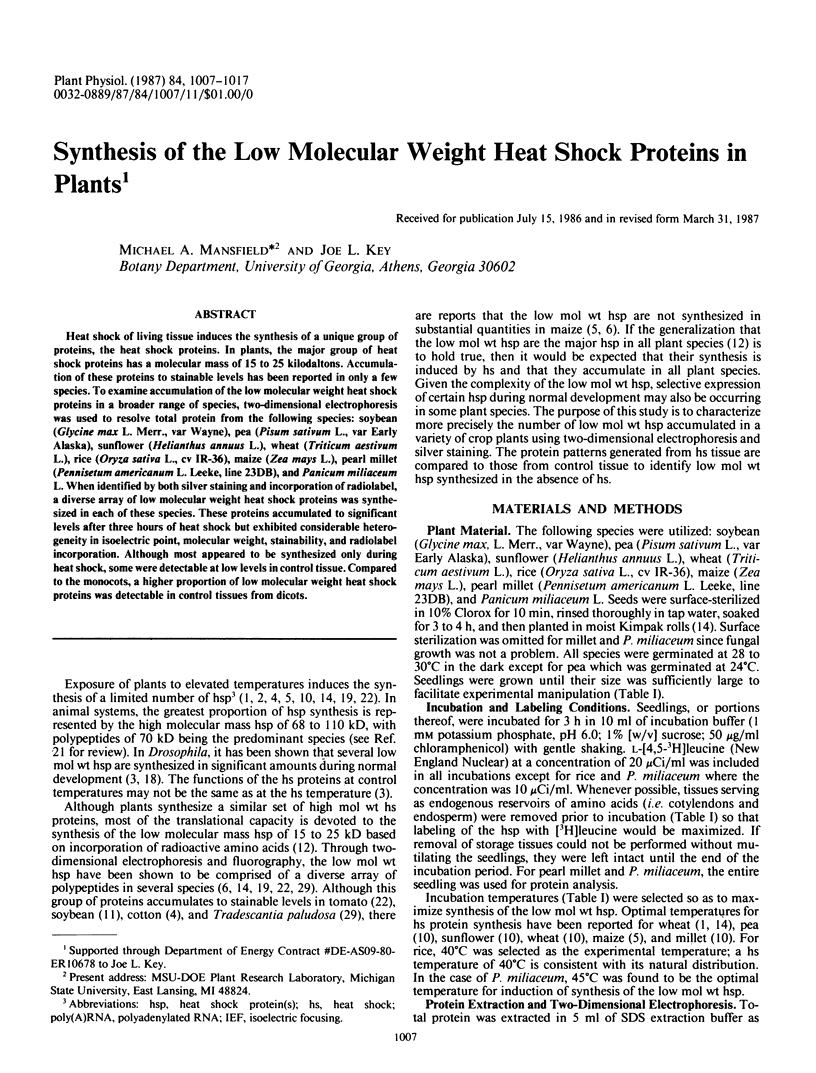
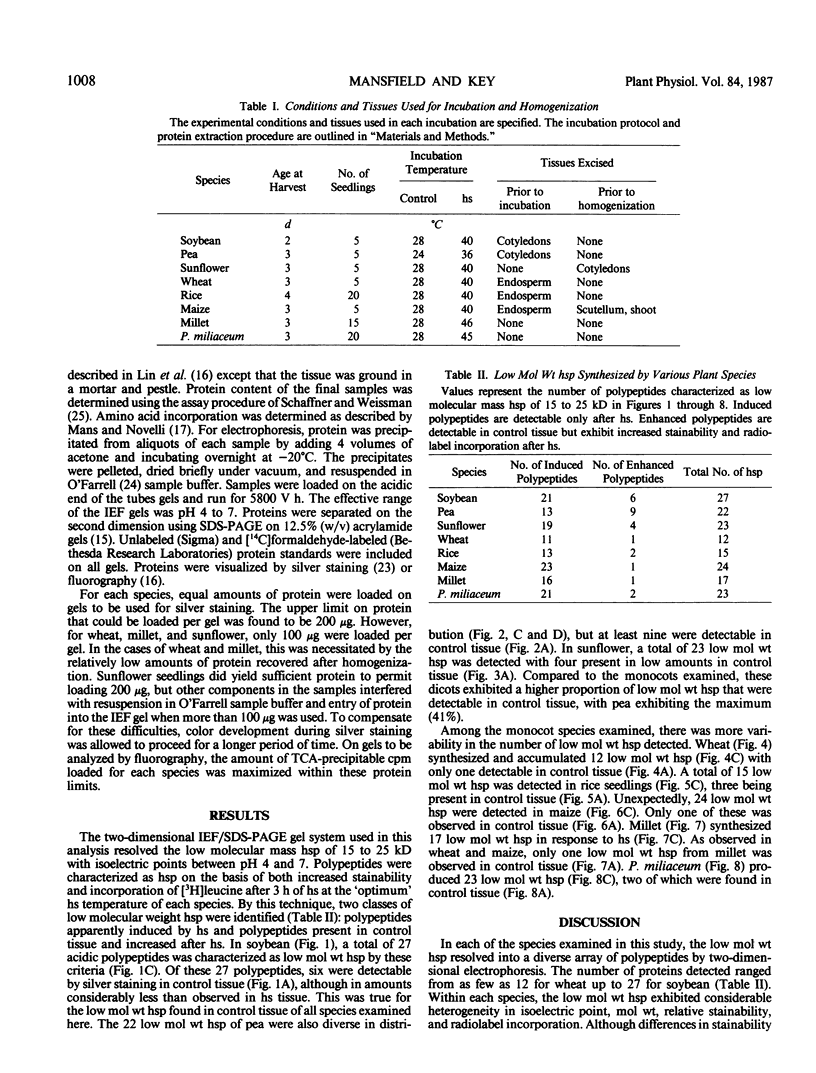
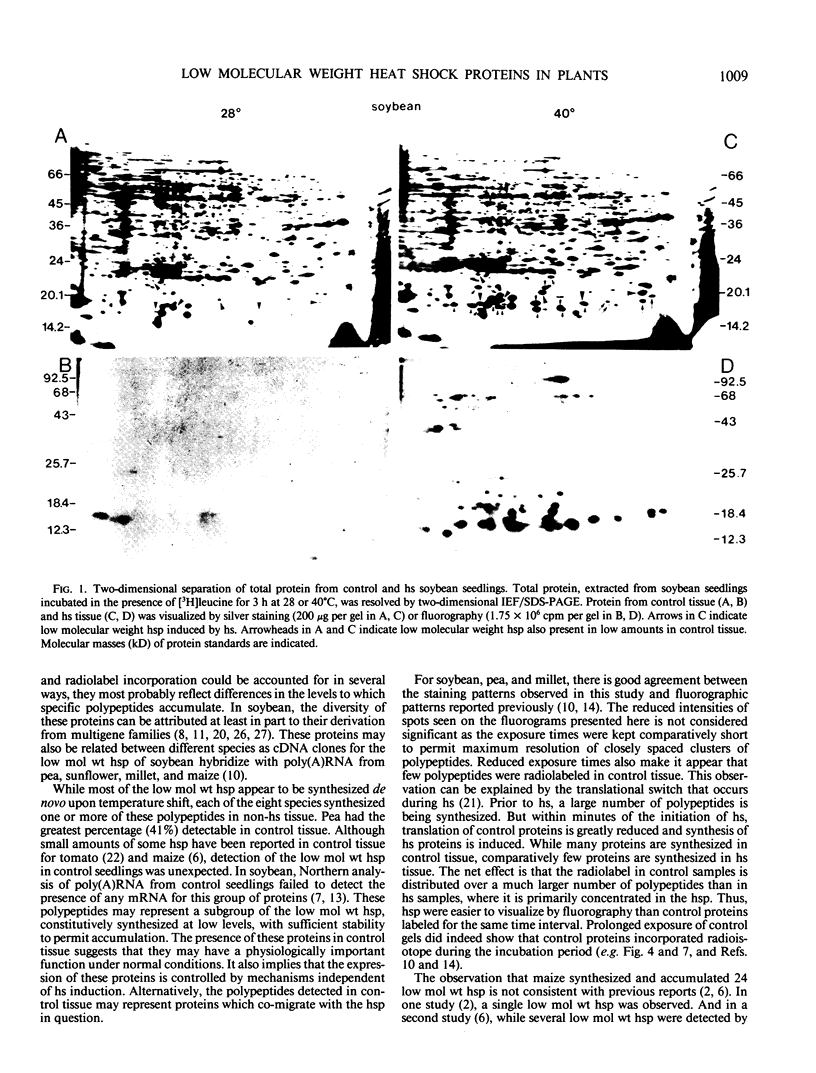
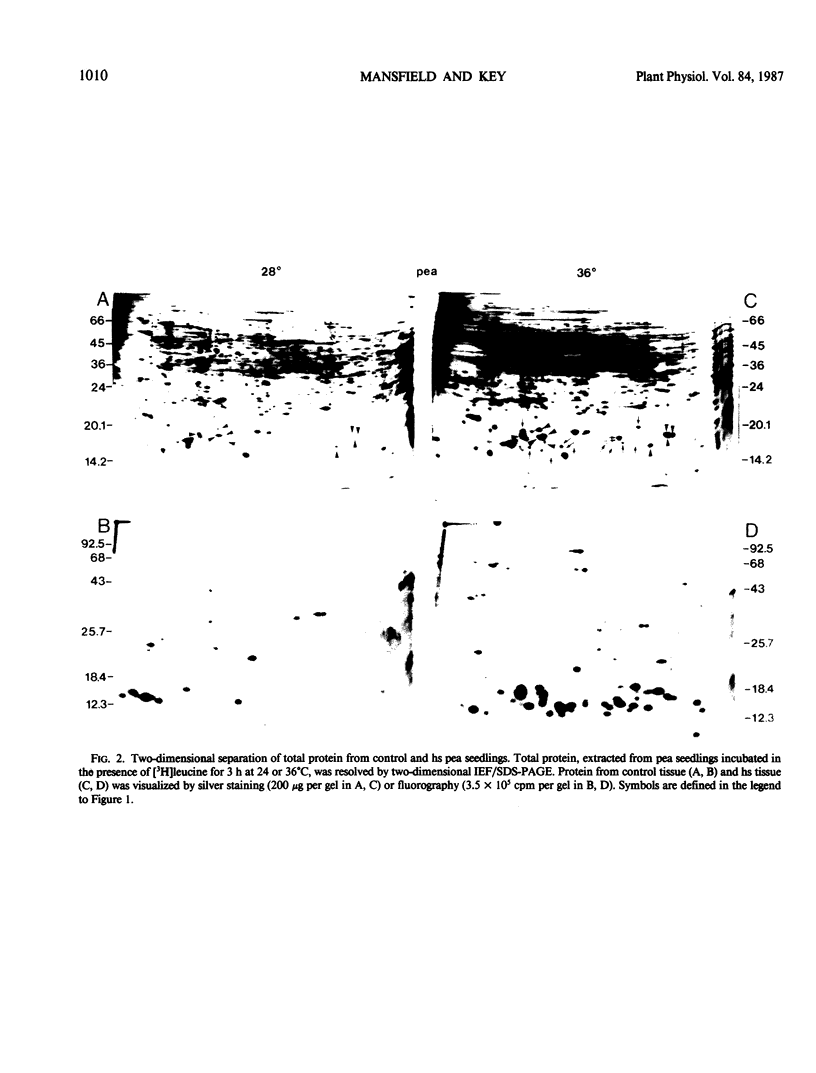
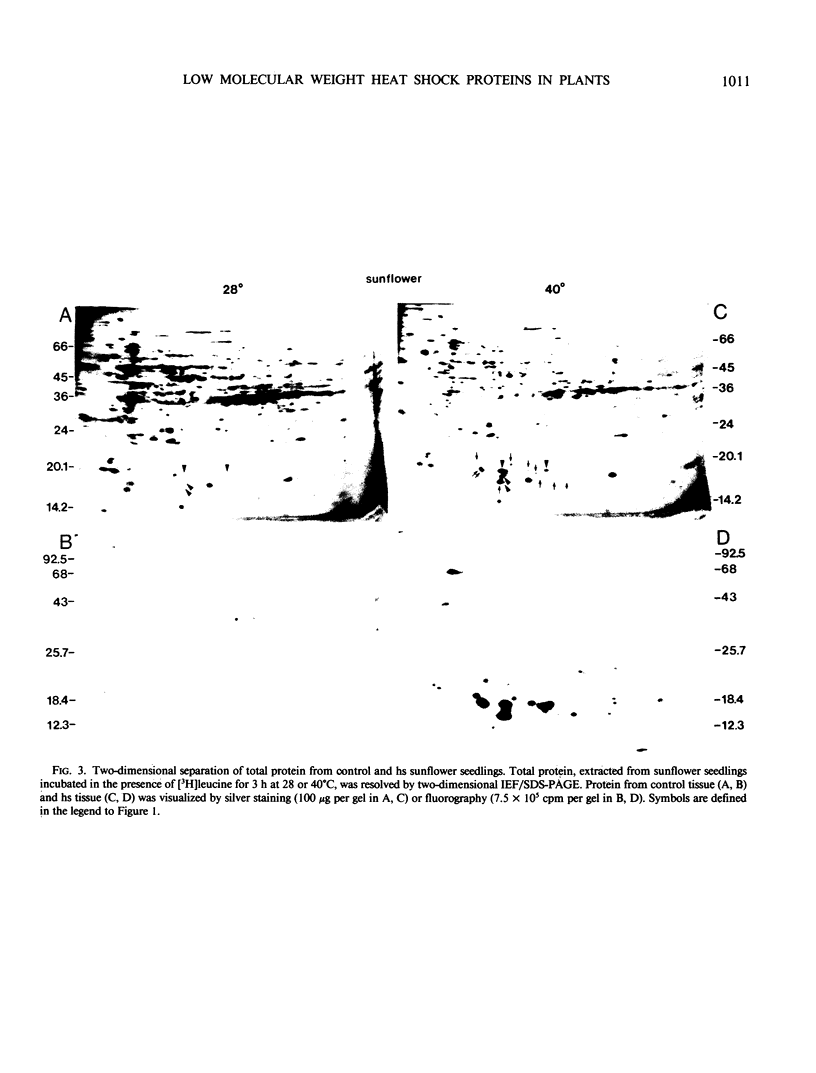
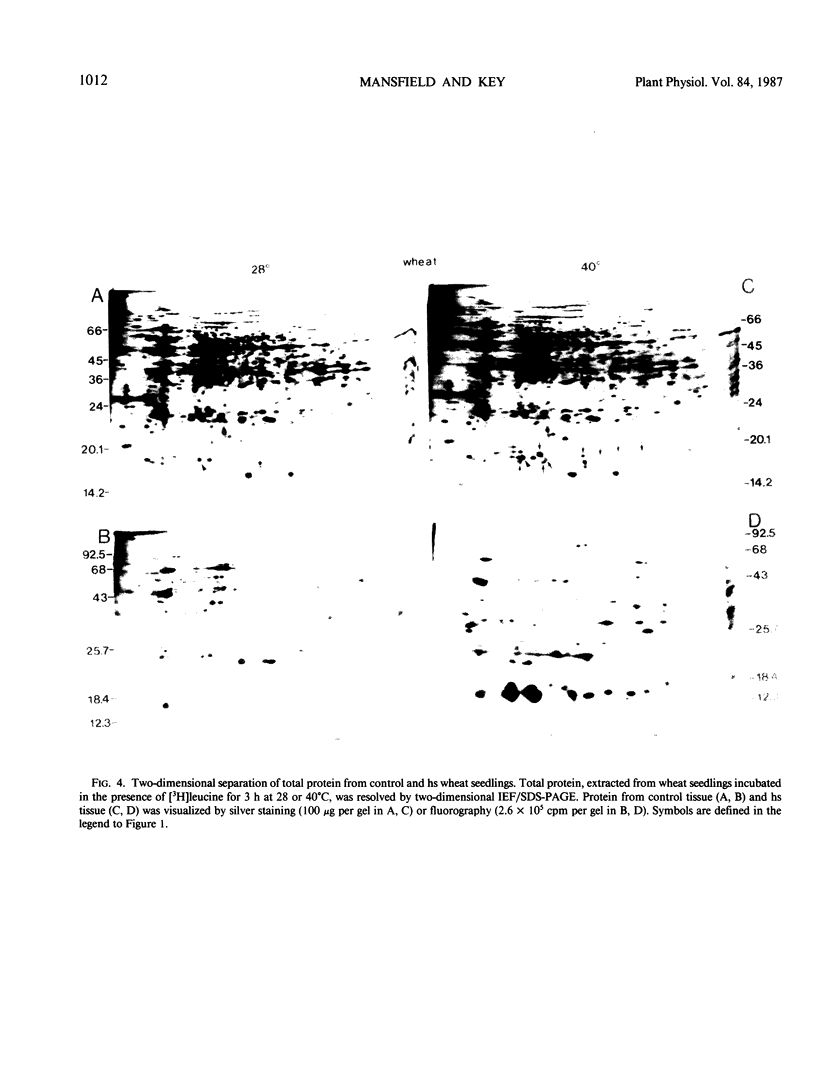
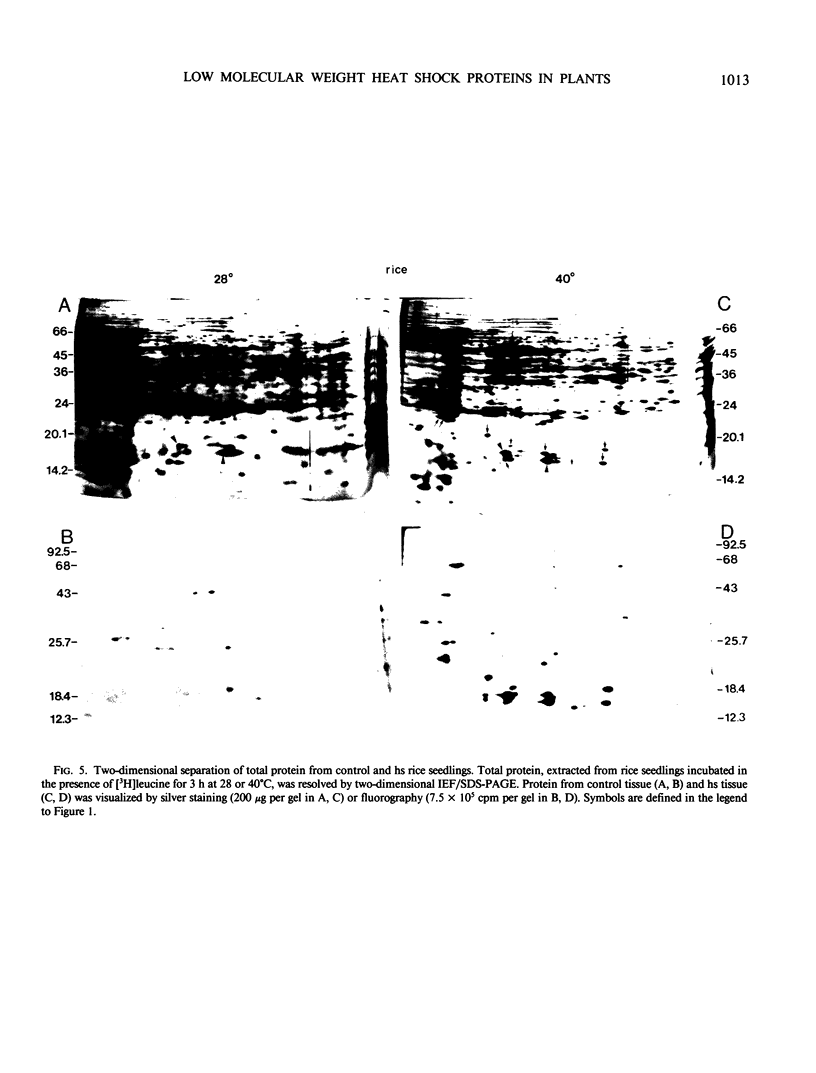
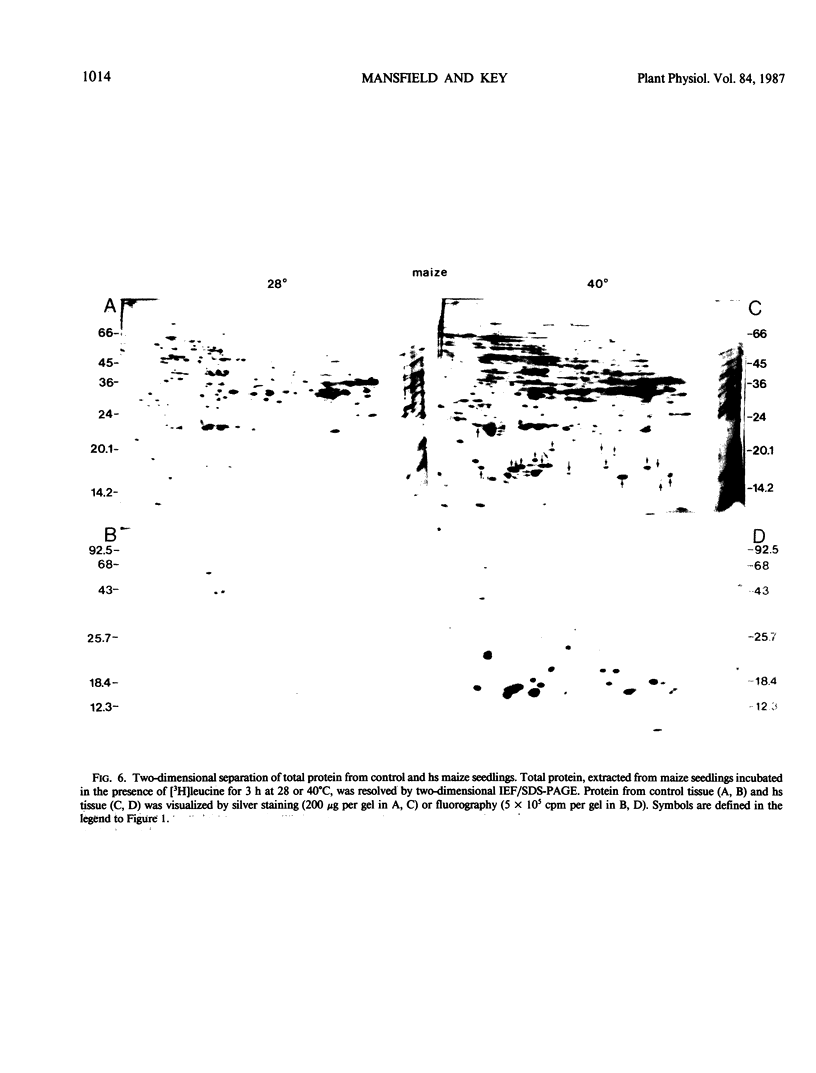
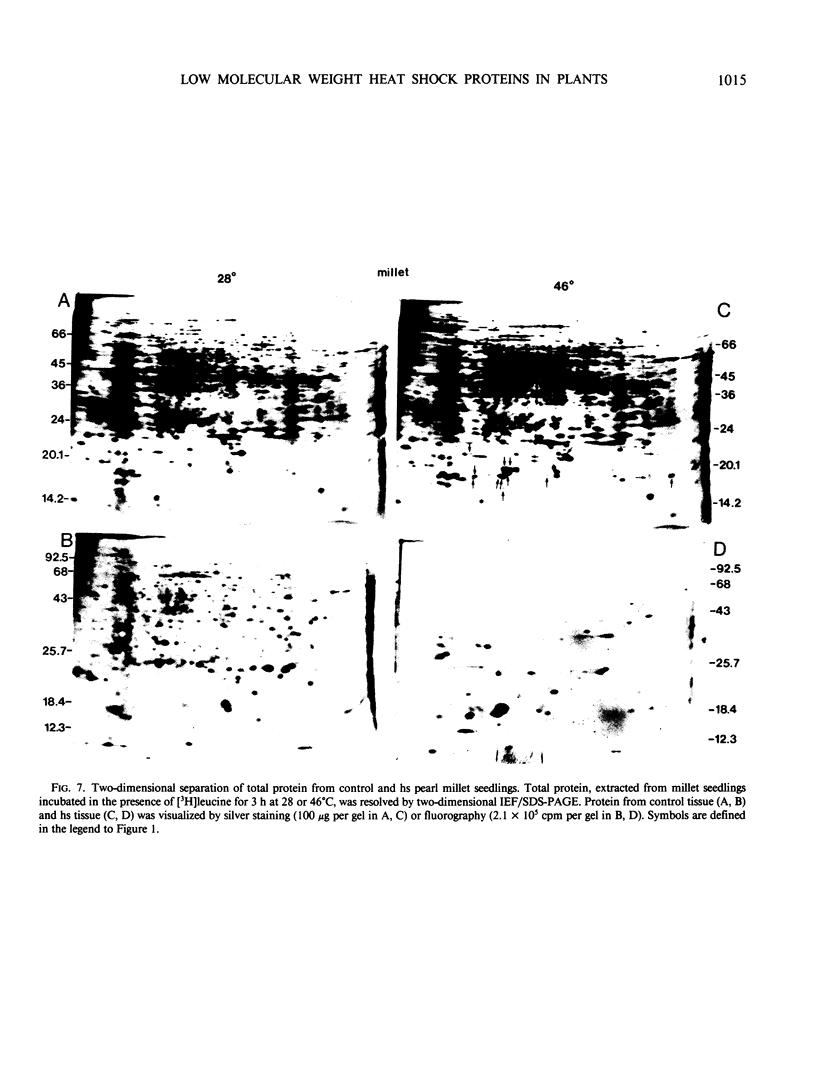
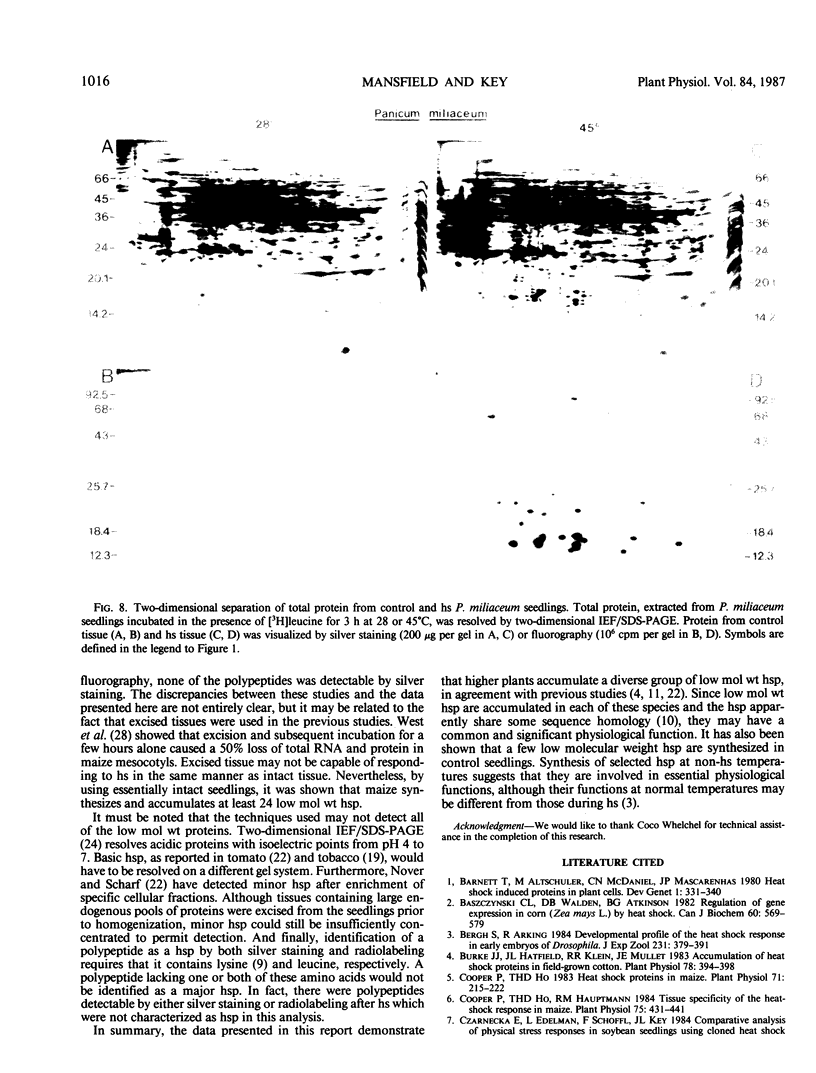
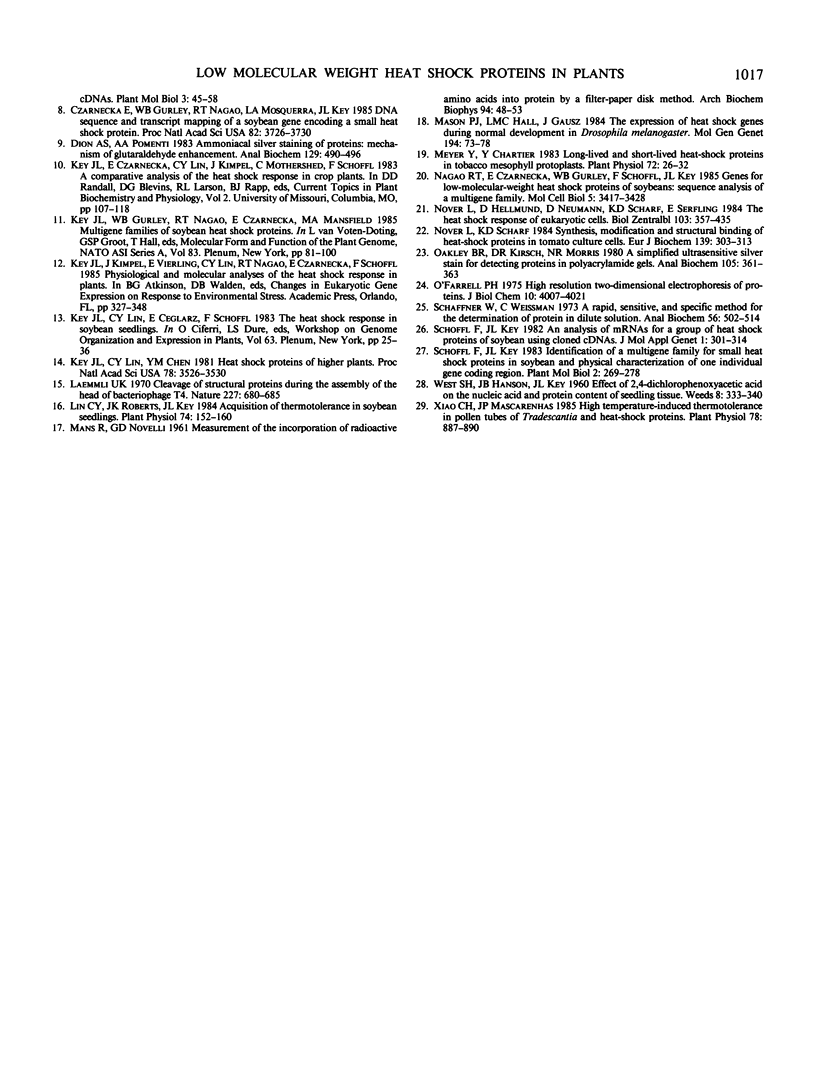
Images in this article
Selected References
These references are in PubMed. This may not be the complete list of references from this article.
- Baszczynski C. L., Walden D. B., Atkinson B. G. Regulation of gene expression in corn (Zea Mays L.) by heat shock. Can J Biochem. 1982 May;60(5):569–579. doi: 10.1139/o82-070. [DOI] [PubMed] [Google Scholar]
- Bergh S., Arking R. Development profile of the heat shock response in early embryos of Drosophila. J Exp Zool. 1984 Sep;231(3):379–391. doi: 10.1002/jez.1402310312. [DOI] [PubMed] [Google Scholar]
- Burke J. J., Hatfield J. L., Klein R. R., Mullet J. E. Accumulation of heat shock proteins in field-grown cotton. Plant Physiol. 1985 Jun;78(2):394–398. doi: 10.1104/pp.78.2.394. [DOI] [PMC free article] [PubMed] [Google Scholar]
- Cooper P., Ho T. H., Hauptmann R. M. Tissue specificity of the heat-shock response in maize. Plant Physiol. 1984 Jun;75(2):431–441. doi: 10.1104/pp.75.2.431. [DOI] [PMC free article] [PubMed] [Google Scholar]
- Cooper P., Ho T. H. Heat shock proteins in maize. Plant Physiol. 1983 Feb;71(2):215–222. doi: 10.1104/pp.71.2.215. [DOI] [PMC free article] [PubMed] [Google Scholar]
- Czarnecka E., Gurley W. B., Nagao R. T., Mosquera L. A., Key J. L. DNA sequence and transcript mapping of a soybean gene encoding a small heat shock protein. Proc Natl Acad Sci U S A. 1985 Jun;82(11):3726–3730. doi: 10.1073/pnas.82.11.3726. [DOI] [PMC free article] [PubMed] [Google Scholar]
- Dion A. S., Pomenti A. A. Ammoniacal silver staining of proteins: mechanism of glutaraldehyde enhancement. Anal Biochem. 1983 Mar;129(2):490–496. doi: 10.1016/0003-2697(83)90582-1. [DOI] [PubMed] [Google Scholar]
- Key J. L., Lin C. Y., Chen Y. M. Heat shock proteins of higher plants. Proc Natl Acad Sci U S A. 1981 Jun;78(6):3526–3530. doi: 10.1073/pnas.78.6.3526. [DOI] [PMC free article] [PubMed] [Google Scholar]
- Laemmli U. K. Cleavage of structural proteins during the assembly of the head of bacteriophage T4. Nature. 1970 Aug 15;227(5259):680–685. doi: 10.1038/227680a0. [DOI] [PubMed] [Google Scholar]
- Lin C. Y., Roberts J. K., Key J. L. Acquisition of Thermotolerance in Soybean Seedlings : Synthesis and Accumulation of Heat Shock Proteins and their Cellular Localization. Plant Physiol. 1984 Jan;74(1):152–160. doi: 10.1104/pp.74.1.152. [DOI] [PMC free article] [PubMed] [Google Scholar]
- Meyer Y., Chartier Y. Long-lived and short-lived heat-shock proteins in tobacco mesophyll protoplasts. Plant Physiol. 1983 May;72(1):26–32. doi: 10.1104/pp.72.1.26. [DOI] [PMC free article] [PubMed] [Google Scholar]
- Nagao R. T., Czarnecka E., Gurley W. B., Schöffl F., Key J. L. Genes for low-molecular-weight heat shock proteins of soybeans: sequence analysis of a multigene family. Mol Cell Biol. 1985 Dec;5(12):3417–3428. doi: 10.1128/mcb.5.12.3417. [DOI] [PMC free article] [PubMed] [Google Scholar]
- Nover L., Scharf K. D. Synthesis, modification and structural binding of heat-shock proteins in tomato cell cultures. Eur J Biochem. 1984 Mar 1;139(2):303–313. doi: 10.1111/j.1432-1033.1984.tb08008.x. [DOI] [PubMed] [Google Scholar]
- O'Farrell P. H. High resolution two-dimensional electrophoresis of proteins. J Biol Chem. 1975 May 25;250(10):4007–4021. [PMC free article] [PubMed] [Google Scholar]
- Oakley B. R., Kirsch D. R., Morris N. R. A simplified ultrasensitive silver stain for detecting proteins in polyacrylamide gels. Anal Biochem. 1980 Jul 1;105(2):361–363. doi: 10.1016/0003-2697(80)90470-4. [DOI] [PubMed] [Google Scholar]
- Schaffner W., Weissmann C. A rapid, sensitive, and specific method for the determination of protein in dilute solution. Anal Biochem. 1973 Dec;56(2):502–514. doi: 10.1016/0003-2697(73)90217-0. [DOI] [PubMed] [Google Scholar]
- Schöffl F., Key J. L. An analysis of mRNAs for a group of heat shock proteins of soybean using cloned cDNAs. J Mol Appl Genet. 1982;1(4):301–314. [PubMed] [Google Scholar]
- Xiao C. M., Mascarenhas J. P. High temperature-induced thermotolerance in pollen tubes of tradescantia and heat-shock proteins. Plant Physiol. 1985 Aug;78(4):887–890. doi: 10.1104/pp.78.4.887. [DOI] [PMC free article] [PubMed] [Google Scholar]