Abstract
Previous labeling studies of abscisic acid (ABA) with 18O2 have been mainly conducted with water-stressed leaves. In this study, 18O incorporation into ABA of stressed leaves of various species was compared with 18O labeling of ABA of turgid leaves and of fruit tissue in different stages of ripening. In stressed leaves of all six species investigated, avocado (Persea americana), barley (Hordeum vulgare), bean (Phaseolus vulgaris), cocklebur (Xanthium strumarium), spinach (Spinacia oleracea), and tobacco (Nicotiana tabacum), 18O was most abundant in the carboxyl group, whereas incorporation of a second and third 18O in the oxygen atoms on the ring of ABA was much less prominent after 24 h in 18O2. ABA from turgid bean leaves showed significant 18O incorporation, again with highest 18O enrichment in the carboxyl group. The 18O-labeling pattern of ABA from unripe avocado mesocarp was similar to that of stressed leaves, but in ripe fruits there was, besides high 18O enrichment in the carboxyl group, also much additional 18O incorporation in the ring. In ripening apple fruit tissue (Malus domestica), singly labeled ABA was most abundant with more 18O incorporated in the tertiary hydroxyl group than in the carboxyl group of ABA. Smaller quantities of this monolabeled product (C-1′-18OH) were also detected in the stressed leaves of barley, bean, and tobacco, and in avocado fruits. It is postulated that a large precursor molecule yields an aldehyde cleavage product that is, in some tissues, rapidly converted to ABA with retention of 18O in the carboxyl group, whereas in ripening fruits and in the stressed leaves of some species the biosynthesis of ABA occurs at a slower rate, allowing this intermediate to exchange 18O with water. On the basis of 18O-labeling patterns observed in ABA from different tissues it is concluded that, despite variations in precursor pool sizes and intermediate turnover rates, there is a universal pathway of ABA biosynthesis in higher plants which involves cleavage of a larger precursor molecule, presumably an oxygenated carotenoid.
Full text
PDF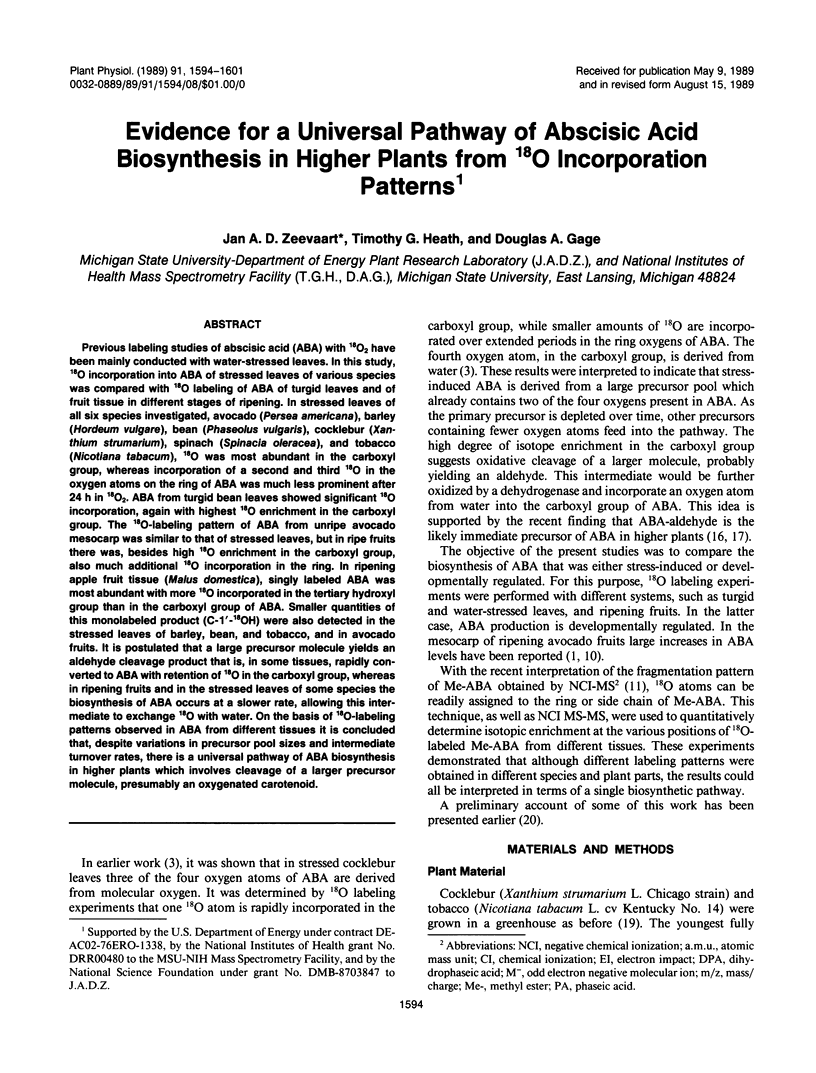
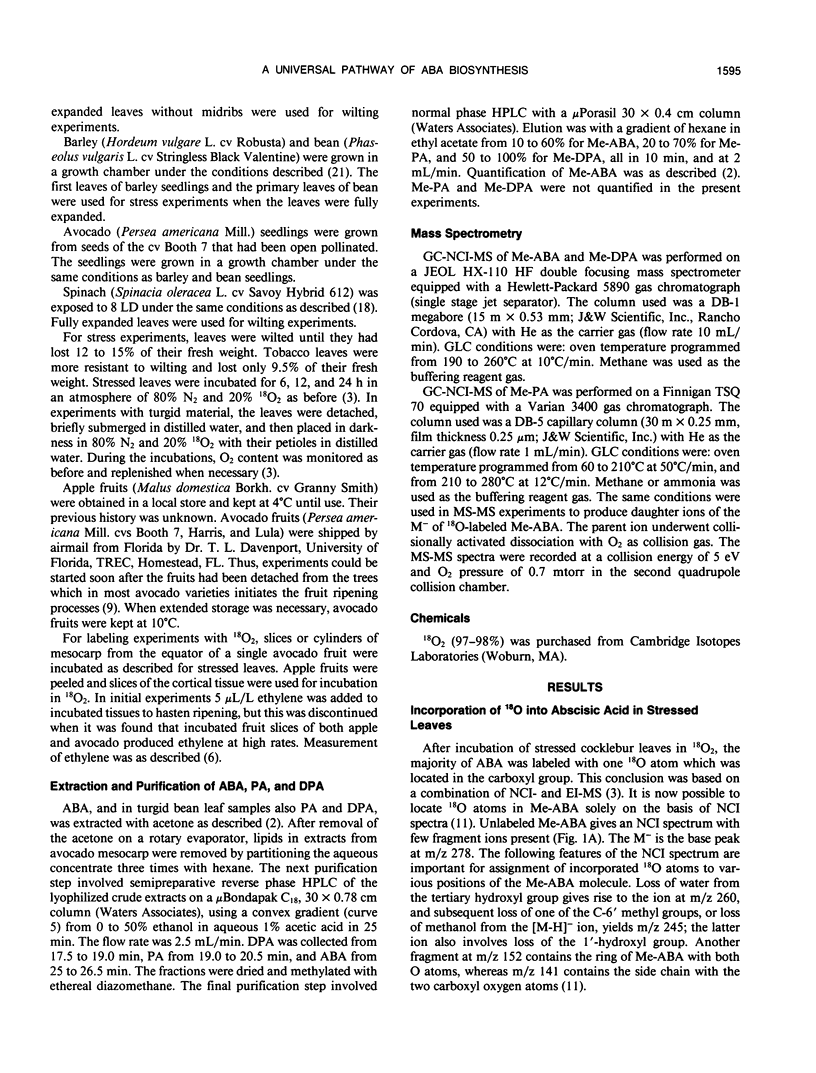
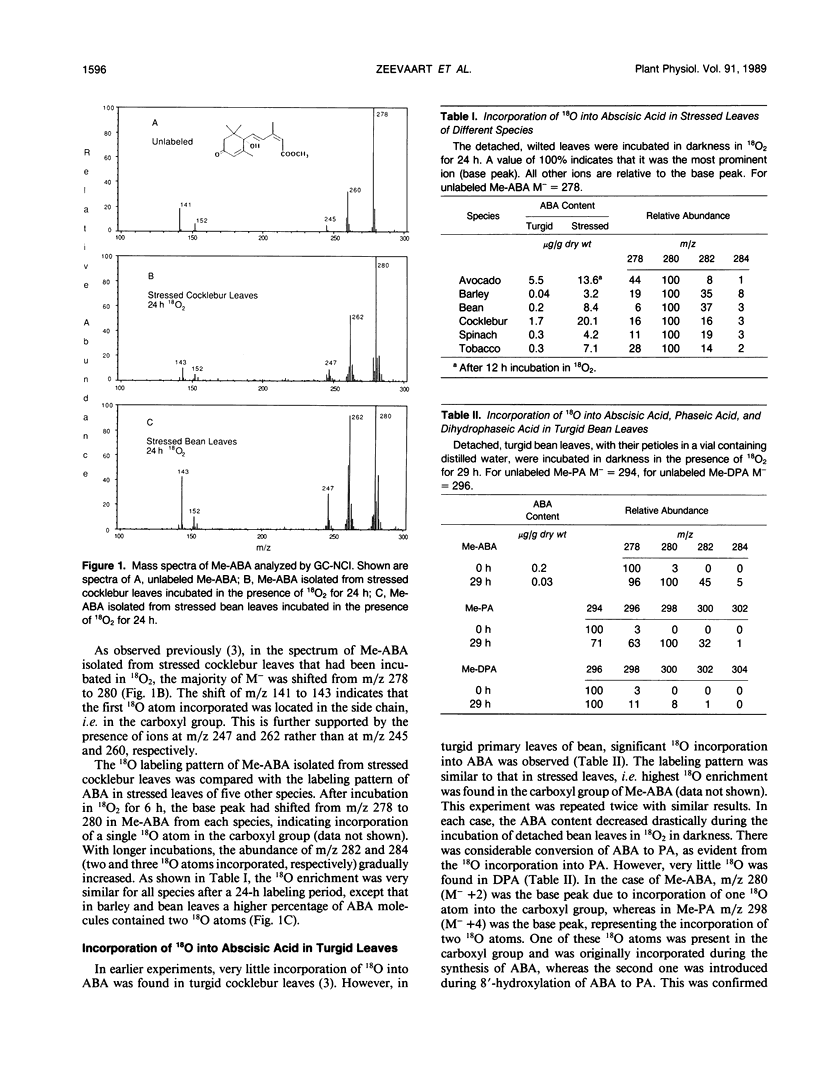
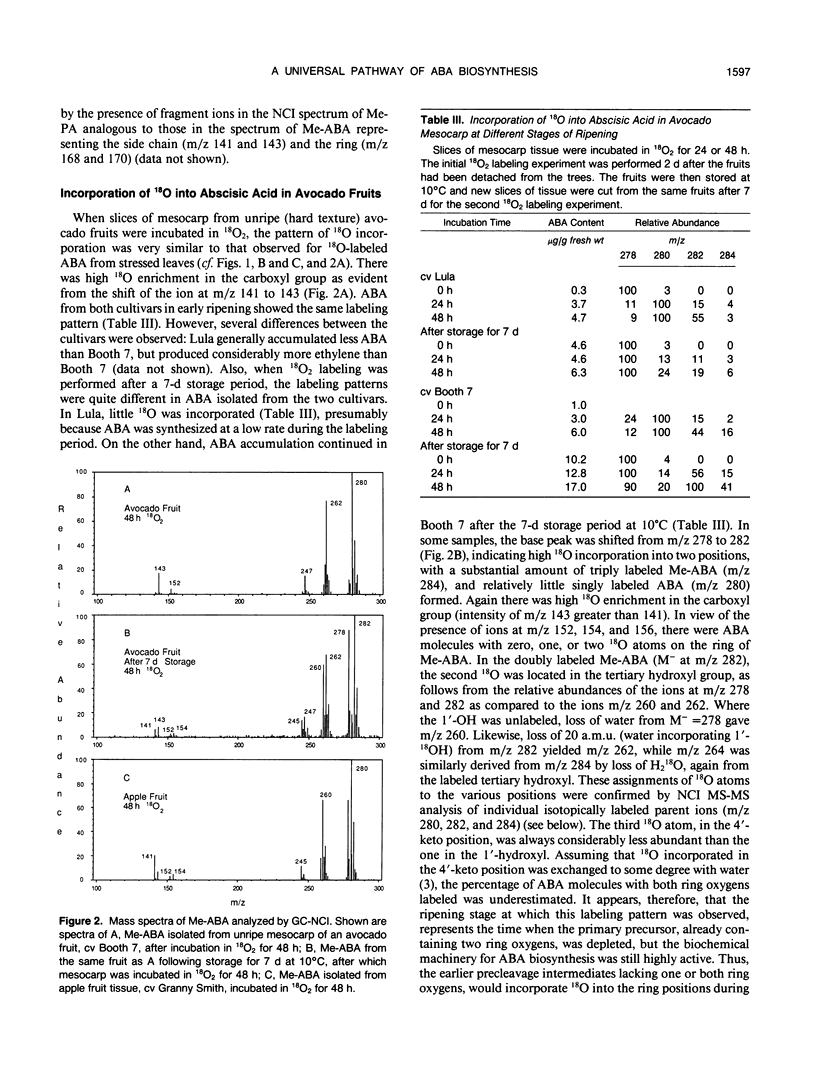
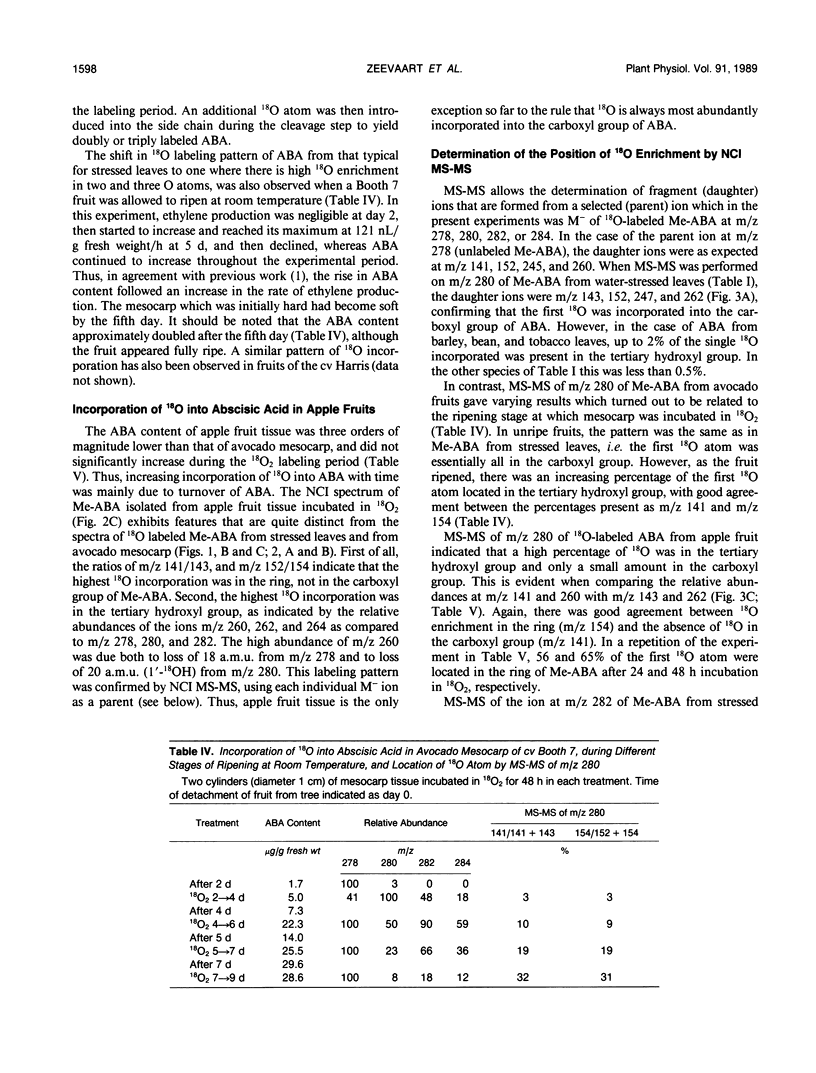
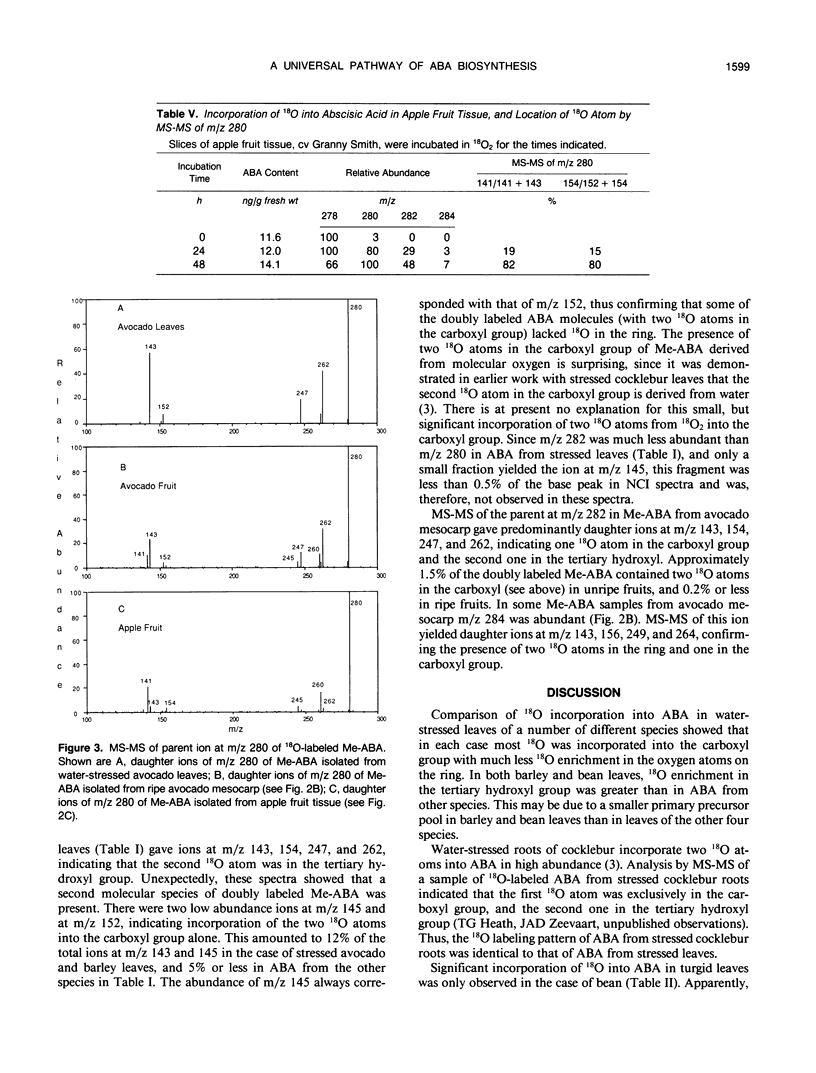
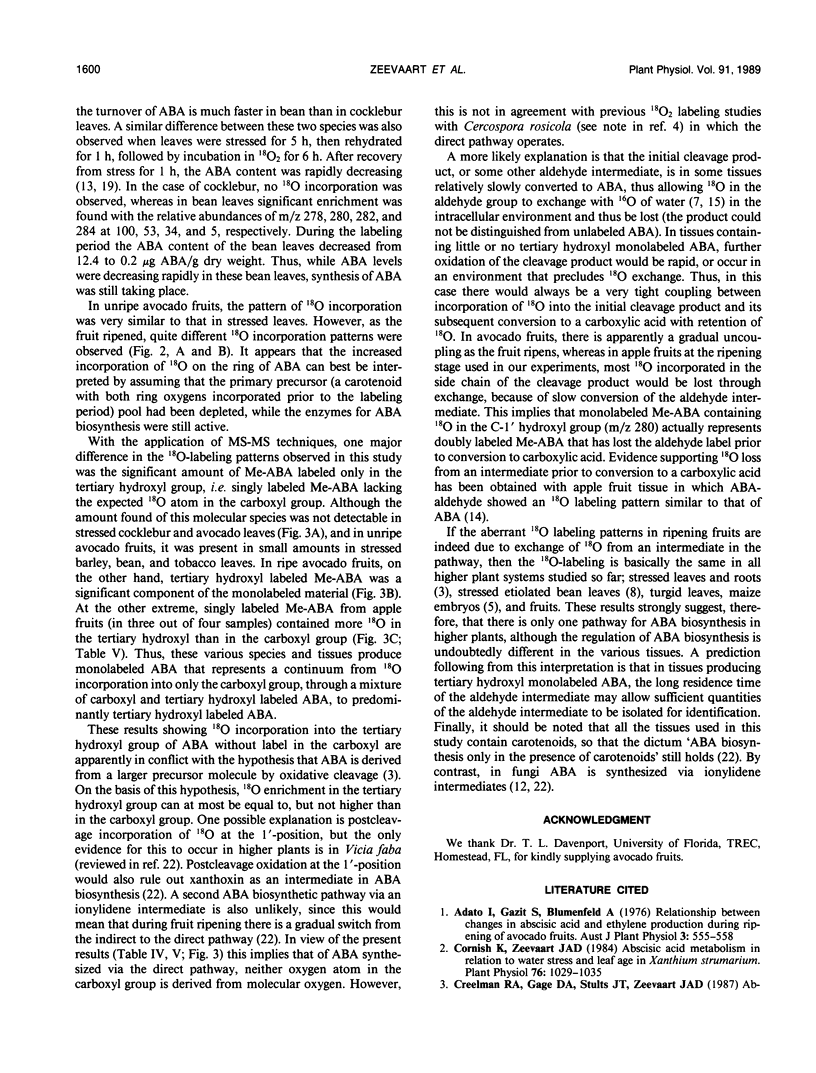
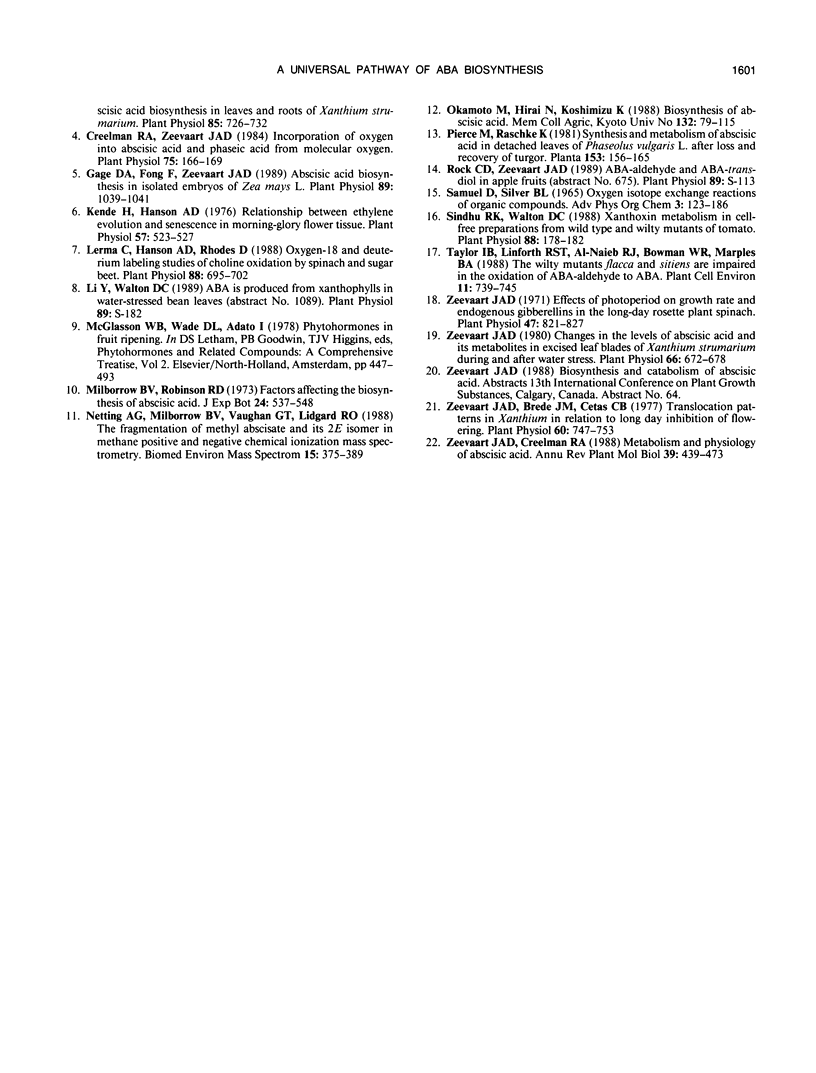
Selected References
These references are in PubMed. This may not be the complete list of references from this article.
- Cornish K., Zeevaart J. A. Abscisic Acid Metabolism in Relation to Water Stress and Leaf Age in Xanthium strumarium. Plant Physiol. 1984 Dec;76(4):1029–1035. doi: 10.1104/pp.76.4.1029. [DOI] [PMC free article] [PubMed] [Google Scholar]
- Creelman R. A., Gage D. A., Stults J. T., Zeevaart J. A. Abscisic Acid Biosynthesis in Leaves and Roots of Xanthium strumarium. Plant Physiol. 1987 Nov;85(3):726–732. doi: 10.1104/pp.85.3.726. [DOI] [PMC free article] [PubMed] [Google Scholar]
- Creelman R. A., Zeevaart J. A. Incorporation of oxygen into abscisic Acid and phaseic Acid from molecular oxygen. Plant Physiol. 1984 May;75(1):166–169. doi: 10.1104/pp.75.1.166. [DOI] [PMC free article] [PubMed] [Google Scholar]
- Fett W. F., Dunn M. F. Exopolysaccharides Produced by Phytopathogenic Pseudomonas syringae Pathovars in Infected Leaves of Susceptible Hosts. Plant Physiol. 1989 Jan;89(1):5–9. doi: 10.1104/pp.89.1.5. [DOI] [PMC free article] [PubMed] [Google Scholar]
- Fett W. F., Dunn M. F. Exopolysaccharides Produced by Phytopathogenic Pseudomonas syringae Pathovars in Infected Leaves of Susceptible Hosts. Plant Physiol. 1989 Jan;89(1):5–9. doi: 10.1104/pp.89.1.5. [DOI] [PMC free article] [PubMed] [Google Scholar]
- Gage D. A., Fong F., Zeevaart J. A. Abscisic Acid Biosynthesis in Isolated Embryos of Zea mays L. Plant Physiol. 1989 Apr;89(4):1039–1041. doi: 10.1104/pp.89.4.1039. [DOI] [PMC free article] [PubMed] [Google Scholar]
- Kende H., Hanson A. D. Relationship between Ethylene Evolution and Senescence in Morning-Glory Flower Tissue. Plant Physiol. 1976 Apr;57(4):523–527. doi: 10.1104/pp.57.4.523. [DOI] [PMC free article] [PubMed] [Google Scholar]
- Lerma C., Hanson A. D., Rhodes D. Oxygen-18 and deuterium labeling studies of choline oxidation by spinach and sugar beet. Plant Physiol. 1988 Nov;88(3):695–702. doi: 10.1104/pp.88.3.695. [DOI] [PMC free article] [PubMed] [Google Scholar]
- Sindhu R. K., Walton D. C. Xanthoxin Metabolism in Cell-free Preparations from Wild Type and Wilty Mutants of Tomato. Plant Physiol. 1988 Sep;88(1):178–182. doi: 10.1104/pp.88.1.178. [DOI] [PMC free article] [PubMed] [Google Scholar]
- Zeevaart J. A., Brede J. M., Cetas C. B. Translocation patterns in xanthium in relation to long day inhibition of flowering. Plant Physiol. 1977 Nov;60(5):747–753. doi: 10.1104/pp.60.5.747. [DOI] [PMC free article] [PubMed] [Google Scholar]
- Zeevaart J. A. Changes in the Levels of Abscisic Acid and Its Metabolites in Excised Leaf Blades of Xanthium strumarium during and after Water Stress. Plant Physiol. 1980 Oct;66(4):672–678. doi: 10.1104/pp.66.4.672. [DOI] [PMC free article] [PubMed] [Google Scholar]
- Zeevaart J. A. Effects of photoperiod on growth rate and endogenous gibberellins in the long-day rosette plant spinach. Plant Physiol. 1971 Jun;47(6):821–827. doi: 10.1104/pp.47.6.821. [DOI] [PMC free article] [PubMed] [Google Scholar]