Abstract
The chemical composition of plasma membrane fractions isolated from orchard grass seedlings (Dactylis glomerata L.) was analyzed and compared with endomembranes. The plasma membrane is characterized by an enrichment of sterols and a lower degree of unsaturation of phospholipids. Steryl glycosides were found to be one of the lipid components of the plasma membrane, but steryl esters and galactolipids were barely detectable. Diphosphatidyl glycerol was characteristically detected in the mitochondrial membrane, but not in the plasma membrane fraction. Plasma mambrane fraction was also characterized by its `lower fluidity' in comparison with the endomembranes. This may be due to the large amount of sterols and the lower degree of phospholipid unsaturation in plasma membranes.
Electrophoretic comparison of polypeptides was also made between different membranes. The distribution patterns of polypeptides revealed on one- and two-dimensional SDS-slab gels were characteristic for those membranes. The presence of glycopeptide complements was a useful criterion for distinguishing plasma membrane from other membranes. The plasma membrane and the ER + Golgi membranes were enriched in glycopeptides. However, a marked difference was revealed in the total number and the molecular weights of the peptides.
During cold acclimation of orchard grass seedlings, the degree of fatty acid unsaturation increased only slightly in plasma membrane, unlike in endomembranes. The change in sterols-to-phospholipids ratio in plasma membrane was also slight. On the other hand, the phospholipid-to-protein ratio increased significantly in the plasma membrane as cold hardiness increased. A significant change in the polypeptide complements of plasma membrane was also demonstrated during cold acclimation.
Full text
PDF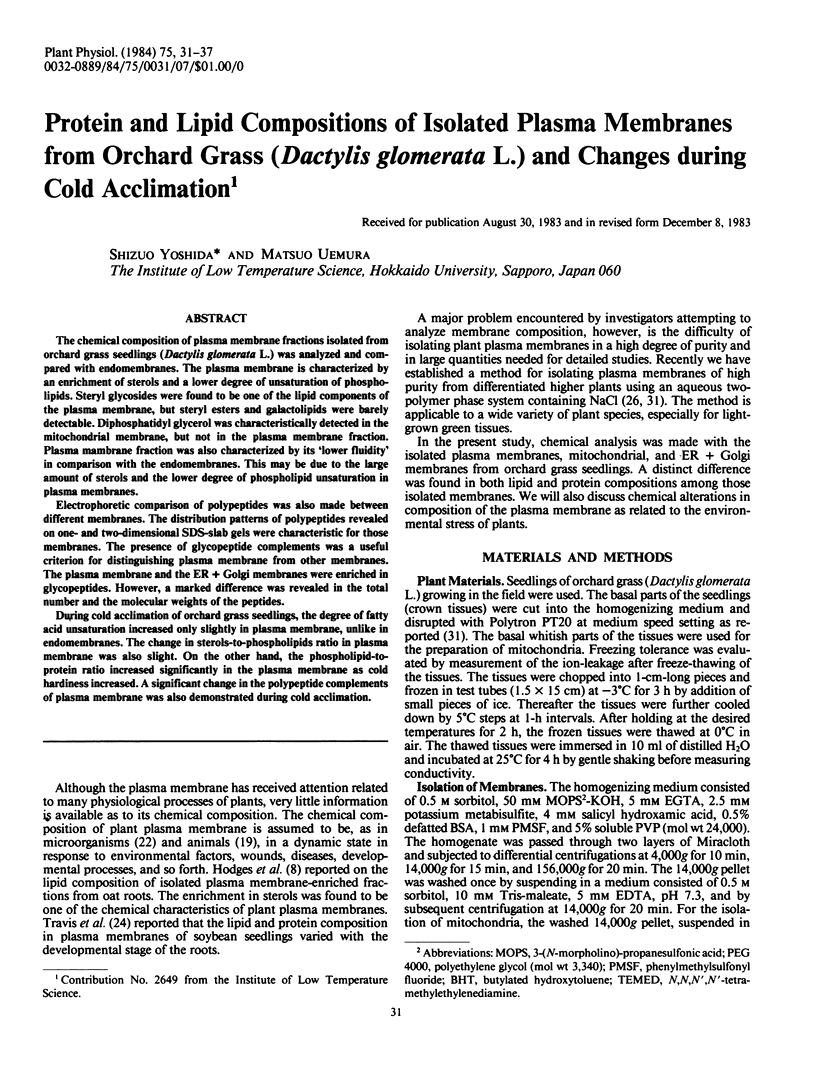
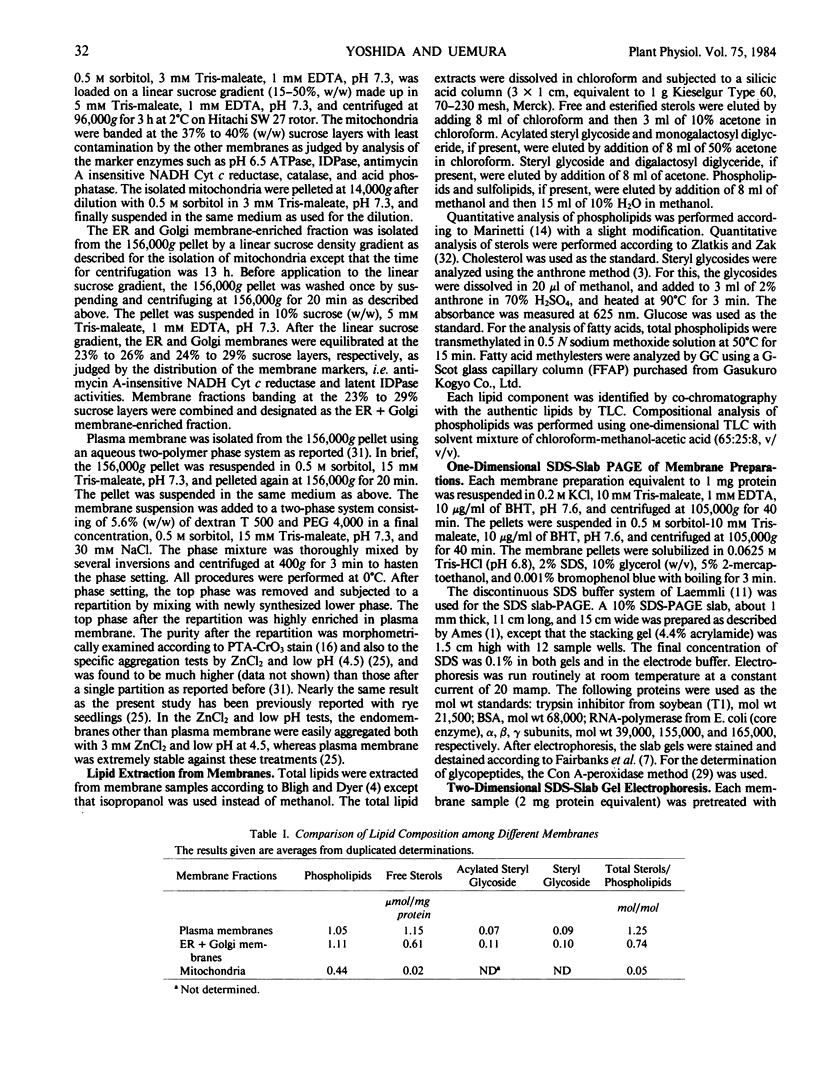
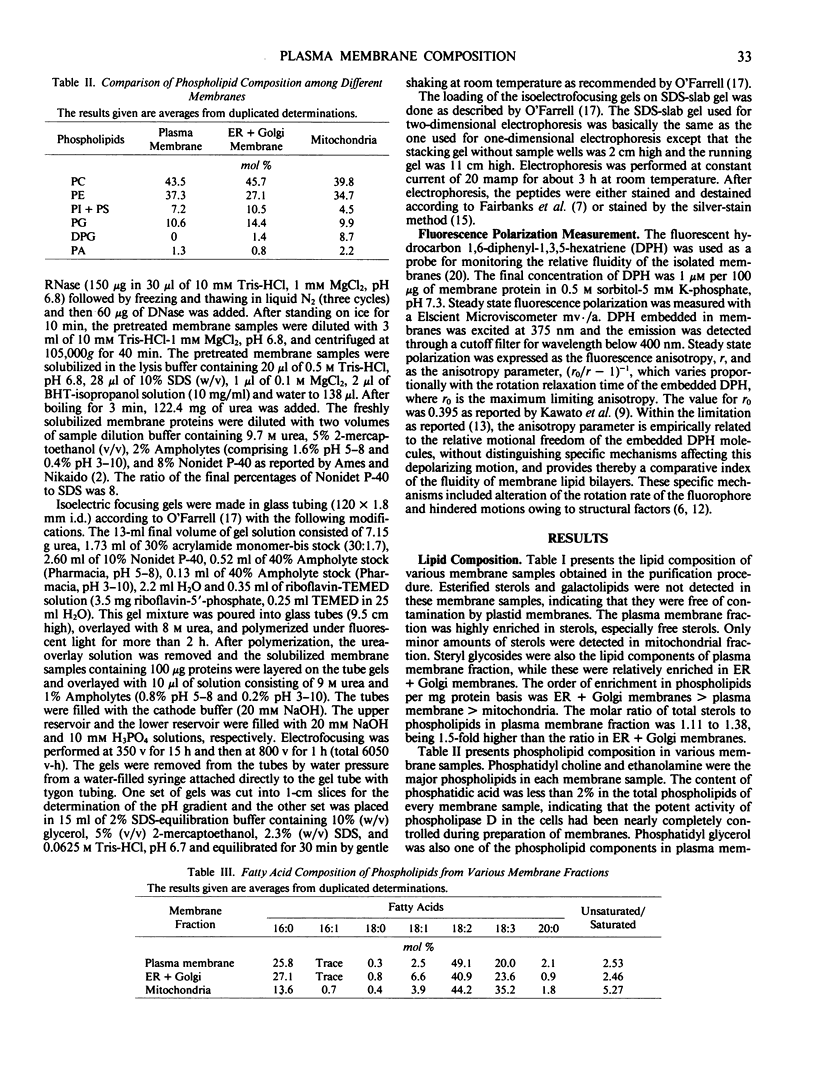
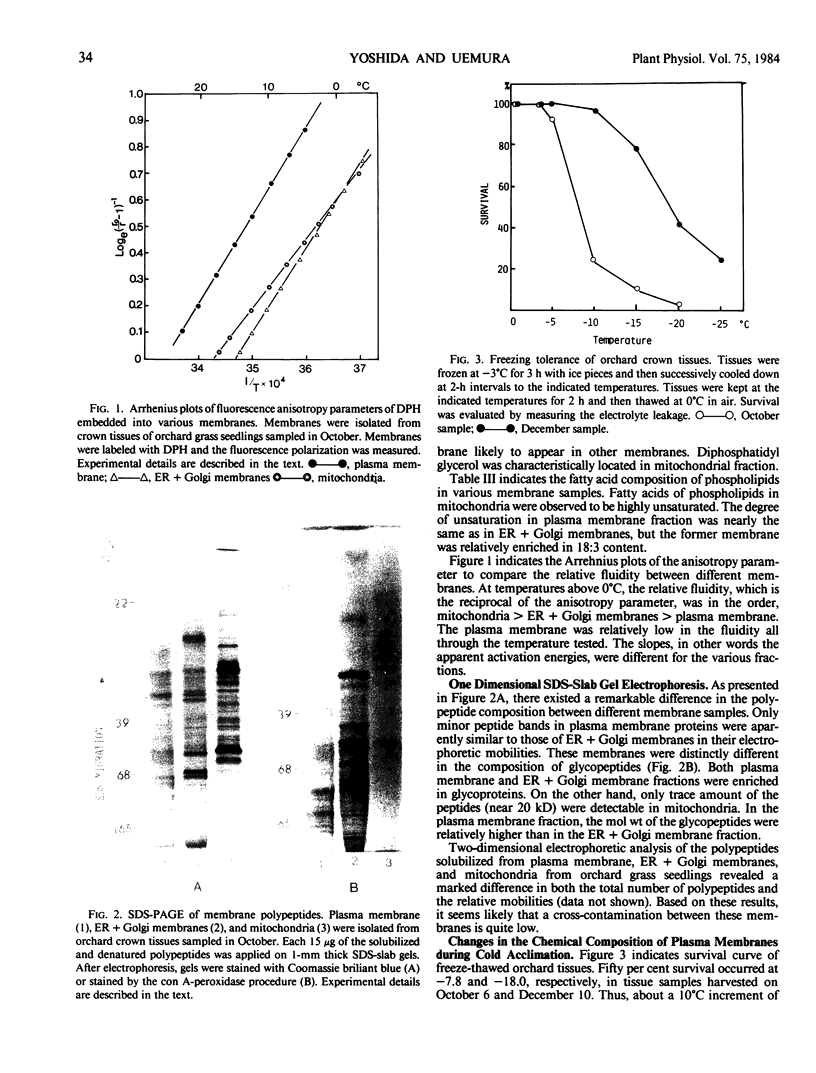
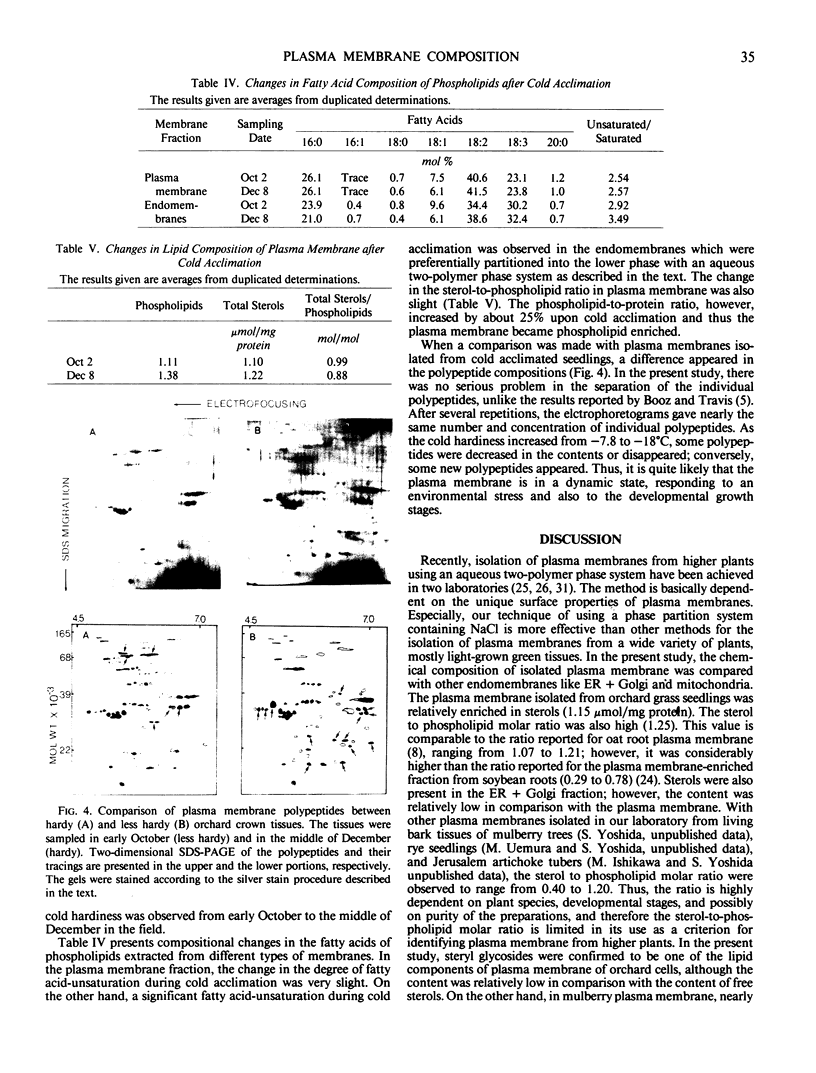
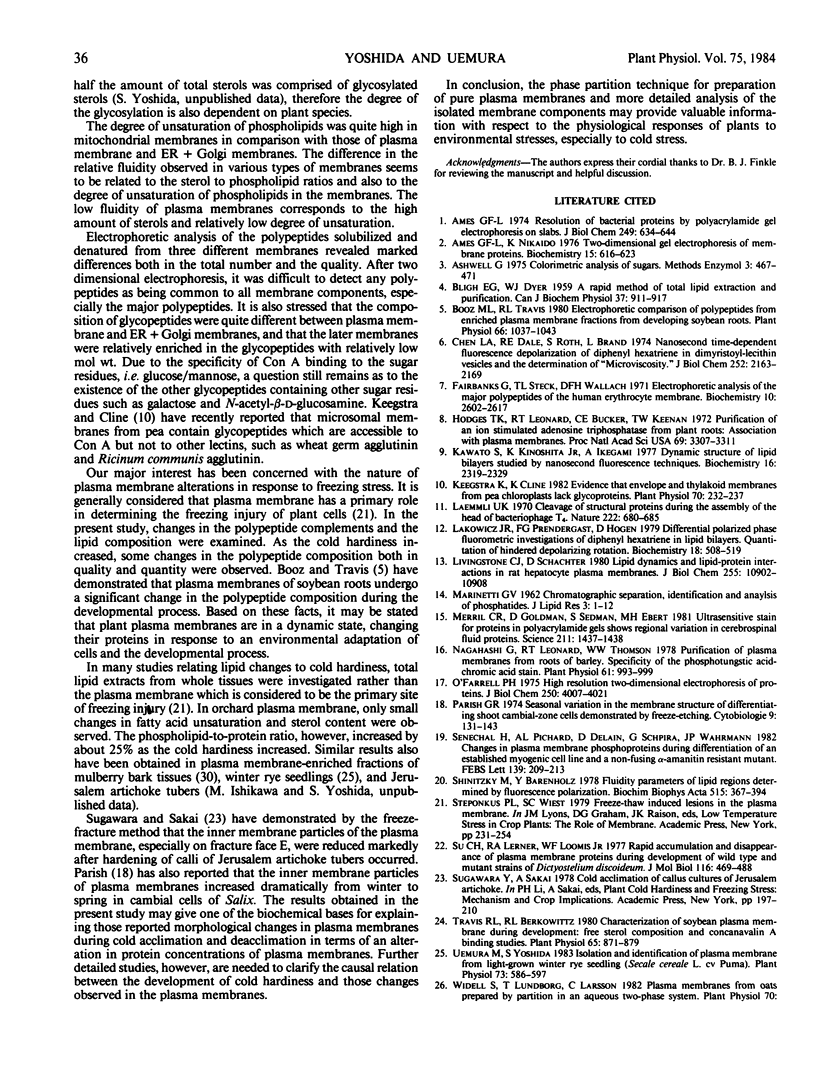
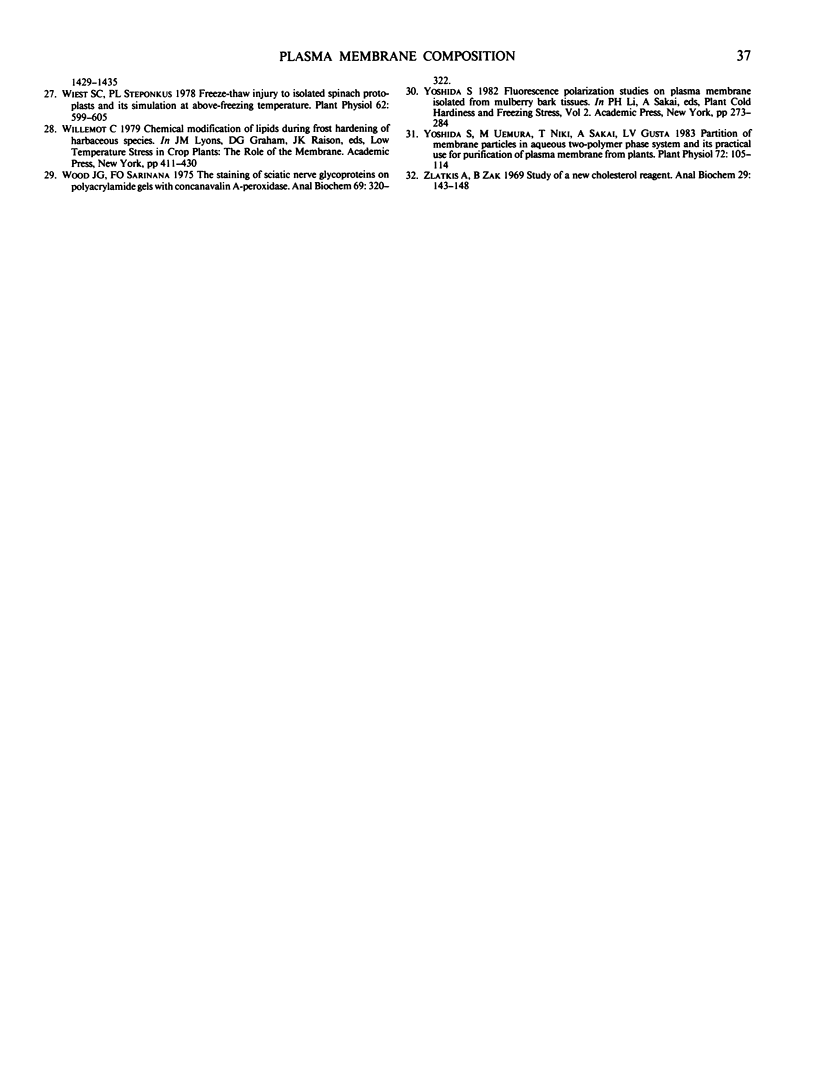
Images in this article
Selected References
These references are in PubMed. This may not be the complete list of references from this article.
- Ames G. F., Nikaido K. Two-dimensional gel electrophoresis of membrane proteins. Biochemistry. 1976 Feb 10;15(3):616–623. doi: 10.1021/bi00648a026. [DOI] [PubMed] [Google Scholar]
- Ames G. F. Resolution of bacterial proteins by polyacrylamide gel electrophoresis on slabs. Membrane, soluble, and periplasmic fractions. J Biol Chem. 1974 Jan 25;249(2):634–644. [PubMed] [Google Scholar]
- BLIGH E. G., DYER W. J. A rapid method of total lipid extraction and purification. Can J Biochem Physiol. 1959 Aug;37(8):911–917. doi: 10.1139/o59-099. [DOI] [PubMed] [Google Scholar]
- Booz M. L., Travis R. L. Electrophoretic comparison of polypeptides from enriched plasma membrane fractions from developing soybean roots. Plant Physiol. 1980 Dec;66(6):1037–1043. doi: 10.1104/pp.66.6.1037. [DOI] [PMC free article] [PubMed] [Google Scholar]
- Chen L. A., Dale R. E., Roth S., Brand L. Nanosecond time-dependent fluorescence depolarization of diphenylhexatriene in dimyristoyllecithin vesicles and the determination of "microviscosity". J Biol Chem. 1977 Apr 10;252(7):2163–2169. [PubMed] [Google Scholar]
- Fairbanks G., Steck T. L., Wallach D. F. Electrophoretic analysis of the major polypeptides of the human erythrocyte membrane. Biochemistry. 1971 Jun 22;10(13):2606–2617. doi: 10.1021/bi00789a030. [DOI] [PubMed] [Google Scholar]
- Hodges T. K., Leonard R. T., Bracker C. E., Keenan T. W. Purification of an ion-stimulated adenosine triphosphatase from plant roots: association with plasma membranes. Proc Natl Acad Sci U S A. 1972 Nov;69(11):3307–3311. doi: 10.1073/pnas.69.11.3307. [DOI] [PMC free article] [PubMed] [Google Scholar]
- Kawato S., Kinosita K., Jr, Ikegami A. Dynamic structure of lipid bilayers studied by nanosecond fluorescence techniques. Biochemistry. 1977 May 31;16(11):2319–2324. doi: 10.1021/bi00630a002. [DOI] [PubMed] [Google Scholar]
- Keegstra K., Cline K. Evidence that Envelope and Thylakoid Membranes from Pea Chloroplasts Lack Glycoproteins. Plant Physiol. 1982 Jul;70(1):232–237. doi: 10.1104/pp.70.1.232. [DOI] [PMC free article] [PubMed] [Google Scholar]
- Laemmli U. K. Cleavage of structural proteins during the assembly of the head of bacteriophage T4. Nature. 1970 Aug 15;227(5259):680–685. doi: 10.1038/227680a0. [DOI] [PubMed] [Google Scholar]
- Lakowicz J. R., Prendergast F. G., Hogen D. Differential polarized phase fluorometric investigations of diphenylhexatriene in lipid bilayers. Quantitation of hindered depolarizing rotations. Biochemistry. 1979 Feb 6;18(3):508–519. doi: 10.1021/bi00570a021. [DOI] [PMC free article] [PubMed] [Google Scholar]
- Livingstone C. J., Schachter D. Lipid dynamics and lipid-protein interactions in rat hepatocyte plasma membranes. J Biol Chem. 1980 Nov 25;255(22):10902–10908. [PubMed] [Google Scholar]
- Merril C. R., Goldman D., Sedman S. A., Ebert M. H. Ultrasensitive stain for proteins in polyacrylamide gels shows regional variation in cerebrospinal fluid proteins. Science. 1981 Mar 27;211(4489):1437–1438. doi: 10.1126/science.6162199. [DOI] [PubMed] [Google Scholar]
- Nagahashi G., Leonard R. T., Thomson W. W. Purification of plasma membranes from roots of barley: specificity of the phosphotungstic Acid-chromic Acid stain. Plant Physiol. 1978 Jun;61(6):993–999. doi: 10.1104/pp.61.6.993. [DOI] [PMC free article] [PubMed] [Google Scholar]
- O'Farrell P. H. High resolution two-dimensional electrophoresis of proteins. J Biol Chem. 1975 May 25;250(10):4007–4021. [PMC free article] [PubMed] [Google Scholar]
- Shinitzky M., Barenholz Y. Fluidity parameters of lipid regions determined by fluorescence polarization. Biochim Biophys Acta. 1978 Dec 15;515(4):367–394. doi: 10.1016/0304-4157(78)90010-2. [DOI] [PubMed] [Google Scholar]
- Siu C. H., Lerner R. A., Loomis W. F., Jr Rapid accumulation and disappearance of plasma membrane proteins during development of wild-type and mutant strains of Dictyostelium discoideum. J Mol Biol. 1977 Nov 5;116(3):469–488. doi: 10.1016/0022-2836(77)90080-8. [DOI] [PubMed] [Google Scholar]
- Sénéchal H., Pichard A. L., Delain D., Schapira G., Wahrmann J. P. Changes in plasma membrane phosphoproteins during differentiation of an established myogenic cell line and a non-fusing alpha-amanitin resistant mutant. FEBS Lett. 1982 Mar 22;139(2):209–213. doi: 10.1016/0014-5793(82)80853-3. [DOI] [PubMed] [Google Scholar]
- Travis R. L., Berkowitz R. L. Characterization of Soybean Plasma Membrane during Development: FREE STEROL COMPOSITION AND CONCANAVALIN A BINDING STUDIES. Plant Physiol. 1980 May;65(5):871–879. doi: 10.1104/pp.65.5.871. [DOI] [PMC free article] [PubMed] [Google Scholar]
- Uemura M., Yoshida S. Isolation and Identification of Plasma Membrane from Light-Grown Winter Rye Seedlings (Secale cereale L. cv Puma). Plant Physiol. 1983 Nov;73(3):586–597. doi: 10.1104/pp.73.3.586. [DOI] [PMC free article] [PubMed] [Google Scholar]
- Wood J. G., Sarinana F. O. The staining of sciatic nerve glycoproteins on polyacrylamide gels with concanavalin A-peroxidase. Anal Biochem. 1975 Nov;69(1):320–322. doi: 10.1016/0003-2697(75)90597-7. [DOI] [PubMed] [Google Scholar]
- Yoshida S., Uemura M., Niki T., Sakai A., Gusta L. V. Partition of membrane particles in aqueous two-polymer phase system and its practical use for purification of plasma membranes from plants. Plant Physiol. 1983 May;72(1):105–114. doi: 10.1104/pp.72.1.105. [DOI] [PMC free article] [PubMed] [Google Scholar]
- Zlatkis A., Zak B. Study of a new cholesterol reagent. Anal Biochem. 1969 Apr 11;29(1):143–148. doi: 10.1016/0003-2697(69)90017-7. [DOI] [PubMed] [Google Scholar]