Abstract
The leaf anatomy and certain photosynthetic properties of nitrate- and ammonia-grown plants of Moricandia arvensis (L.) DC., a species previously reported to be a C3-C4 intermediate, were investigated. Nitrate-grown plants had a high level of malate in the leaves while ammonia-grown plants had low levels of malate. In young leaves of nitrate-grown plants, there was a diurnal fluctuation of malate content, increasing during the day and decreasing during the night. Titratable acidity remained low in leaves of both nitrate- and ammonia-grown plants.
In nitrate-grown plants, the activity of phosphoenolpyruvate (PEP) carboxylase was about 2-fold higher than in ammonia-grown plants, the latter having activity typical of C3 species. Also, in nitrate-grown plants, the ratio of activities of ribulose 1,5-bisphosphate (RuBP) carboxylase/PEP carboxylase was lower than in ammonia-grown plants. Nitrate reductase activities were higher in nitrate- than in ammonia-grown plants and the greatest activity was found in younger leaves.
With nitrate-grown plants, during a pulse-chase experiment the label in malate, as a percentage of the total labeled products, increased from about 7% after a 10-second pulse with 14CO2 up to 17% during a 5-minute chase with 12CO2. The pattern of 14C labeling in various metabolites suggests the primary carboxylation is through RuBP carboxylase with a secondary carboxylation through PEP carboxylase. In similar experiments, with ammonia-grown plants, the percentage label in malate was only 0% to 4% with no increase in malate labeling during the chase period. The CO2 compensation point was lower in nitrate-grown than ammonia-grown plants.
There was no evidence of Kranz-like anatomy in either the nitrate or ammonia-grown plants. Mitochondria of bundle-sheath cells were strikingly positioned along the inner tangential wall. This might allow the chloroplasts of these cells to fix the mitochondrial photorespired CO2 more effectively and contribute to the low CO2 compensation point in the species. Chloroplasts of bundle-sheath cells and contiguous mesophyll cells were similar in size and structure in plants grown on different media, although chloroplast thylakoids and stromata of the ammonia-grown plants stained more intensely than those of nitrate-grown plants. In addition, irregular clusters of phytoferritin particles occurred in the chloroplasts of the ammonia-grown plants.
The results indicate that the substantial activity of PEP carboxylase, incorporation of CO2 into malate, the high malate content, and in part the relatively low CO2 compensation point in Moricandia arvensis may be accounted for by metabolism of nitrate rather than by a state of C3-C4 intermediacy.
Full text
PDF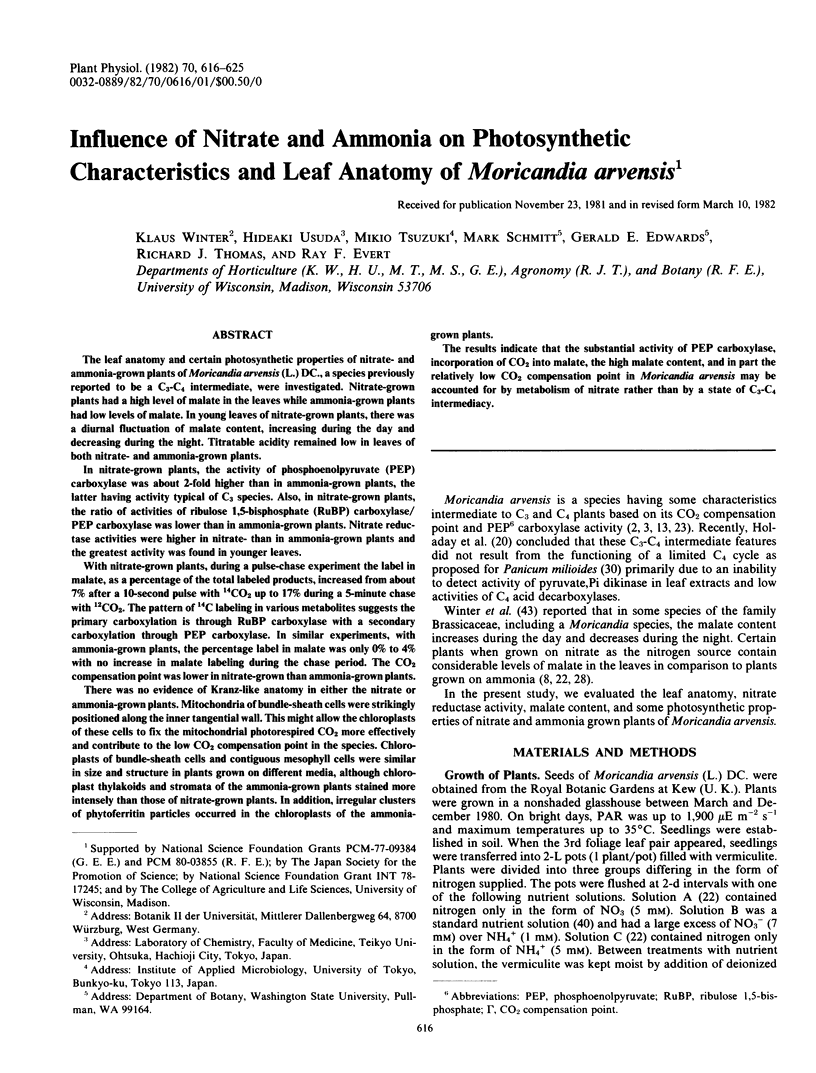
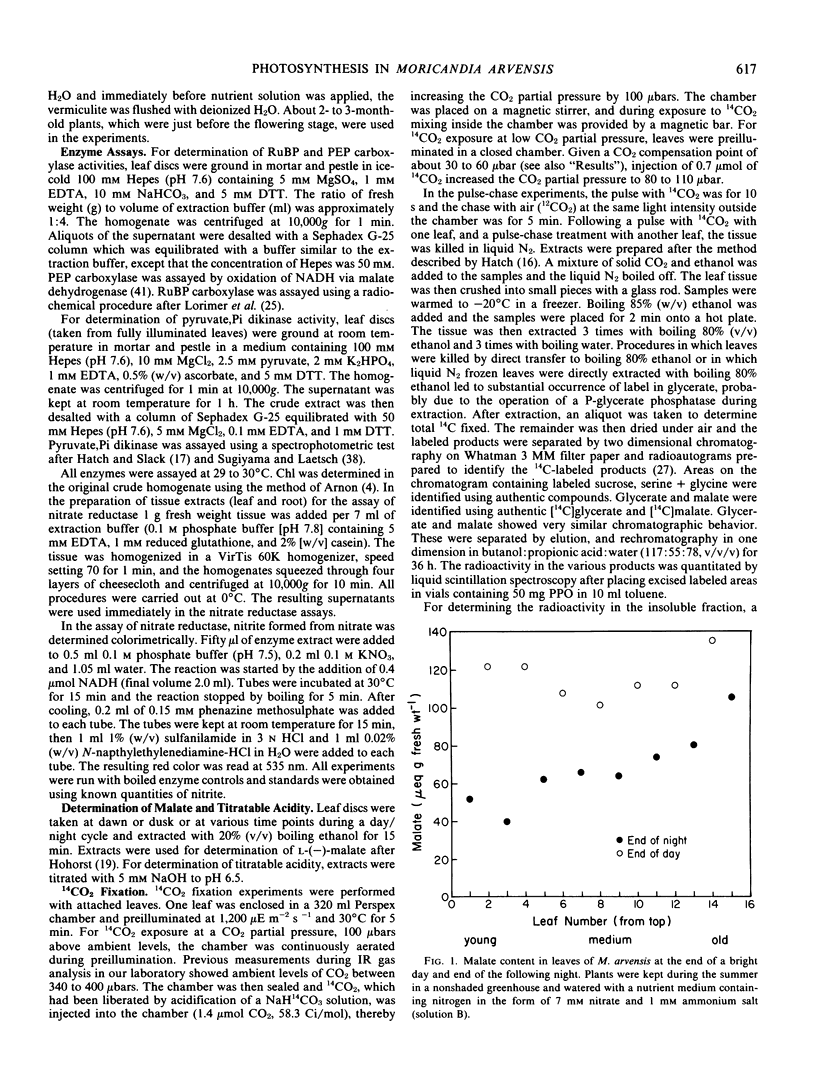
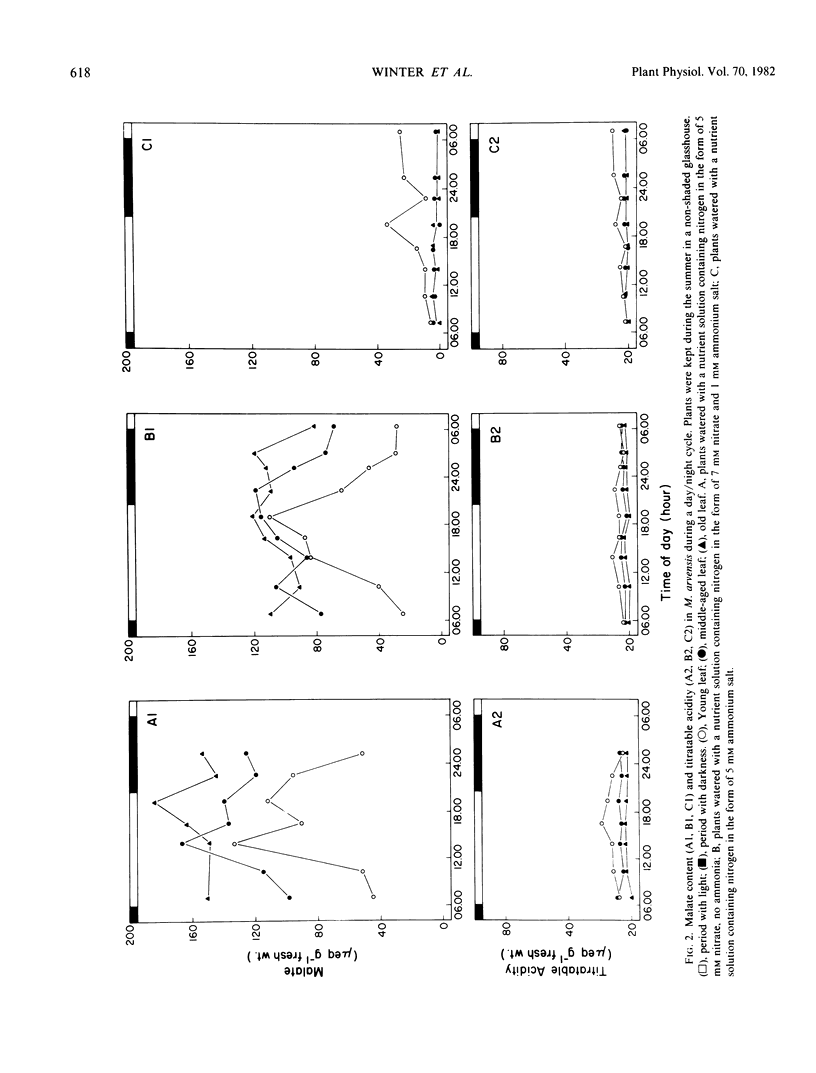
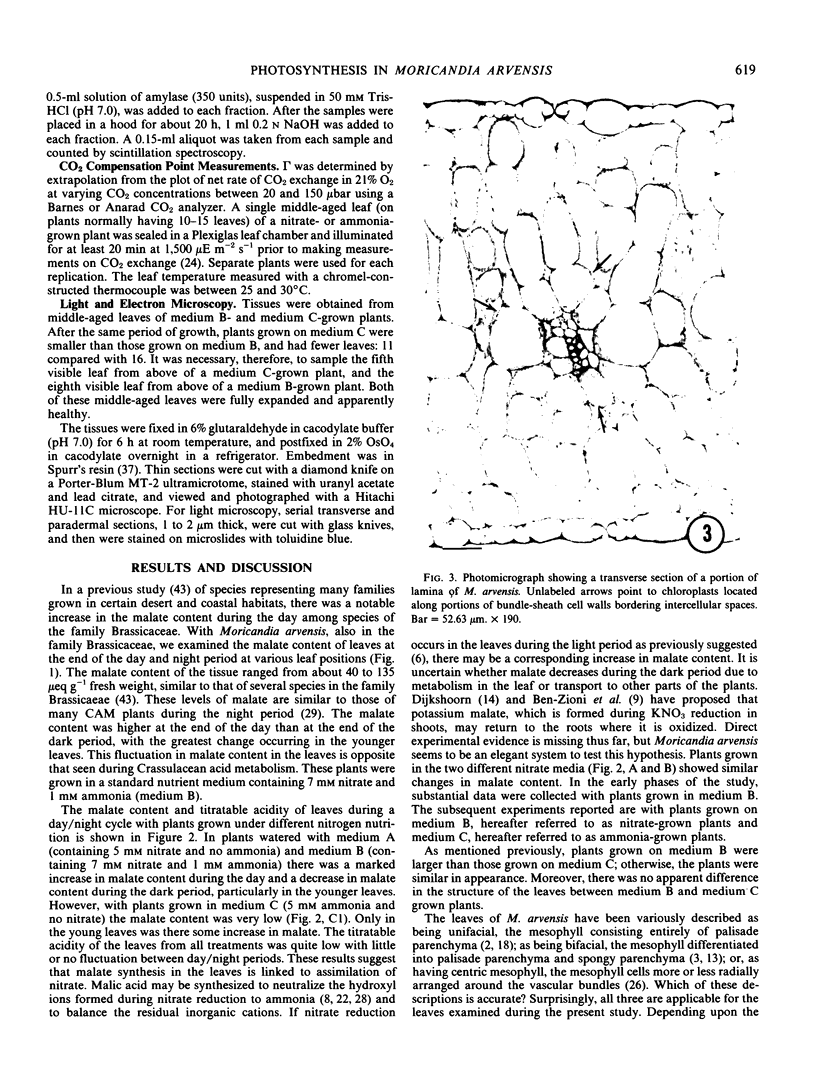
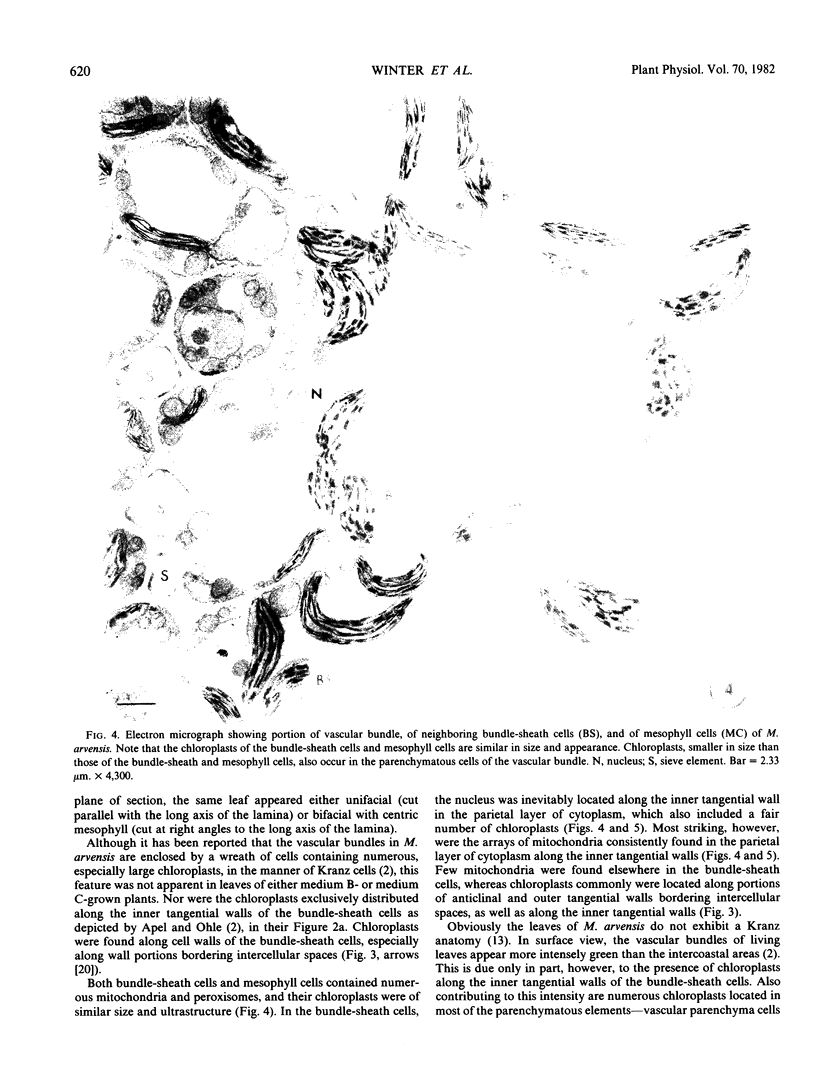
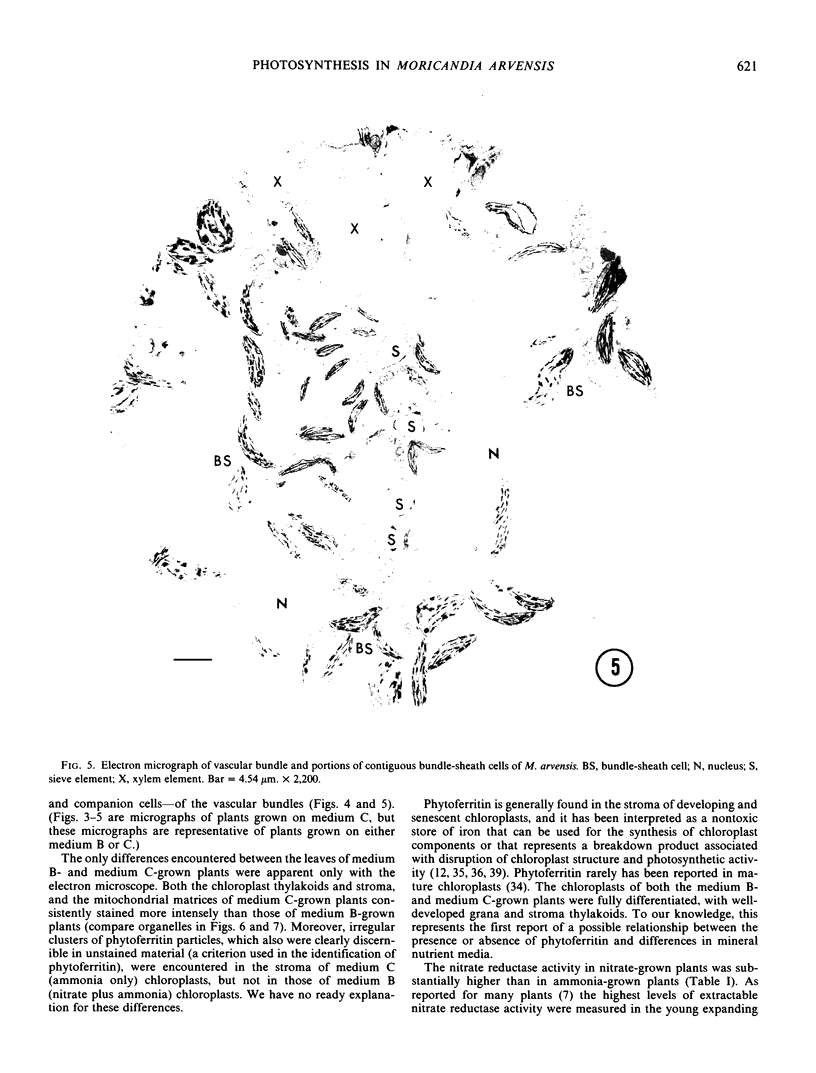
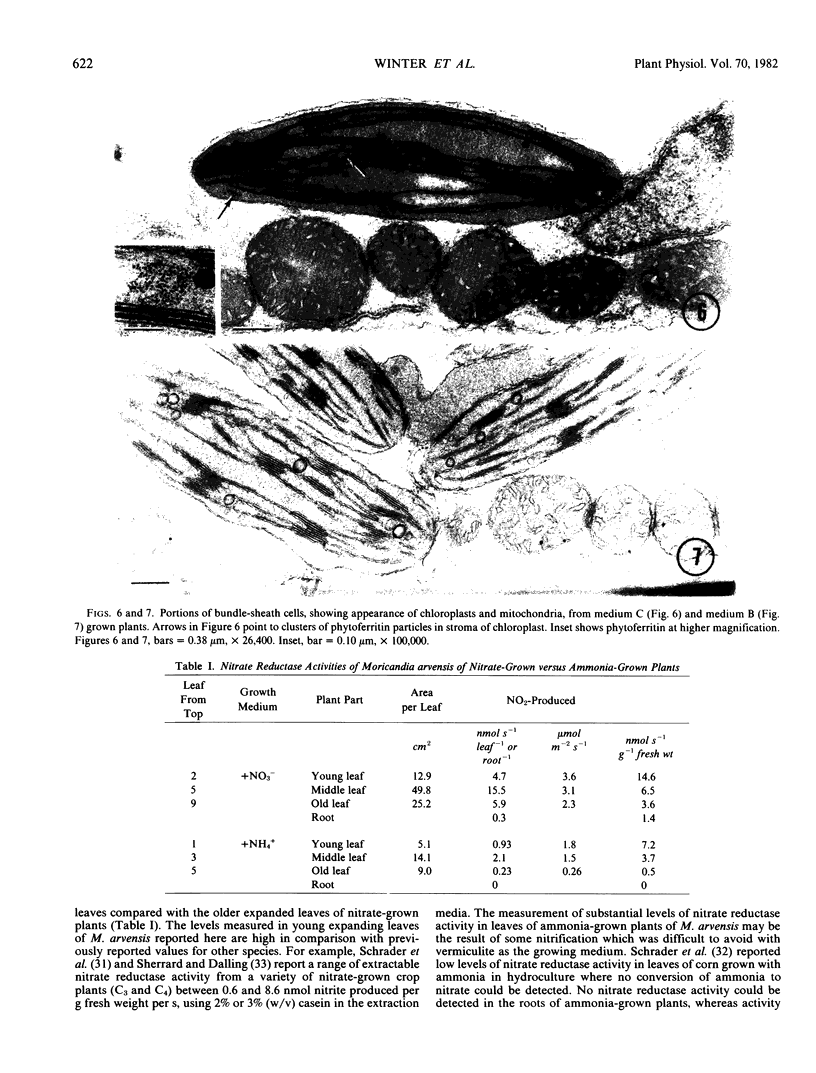
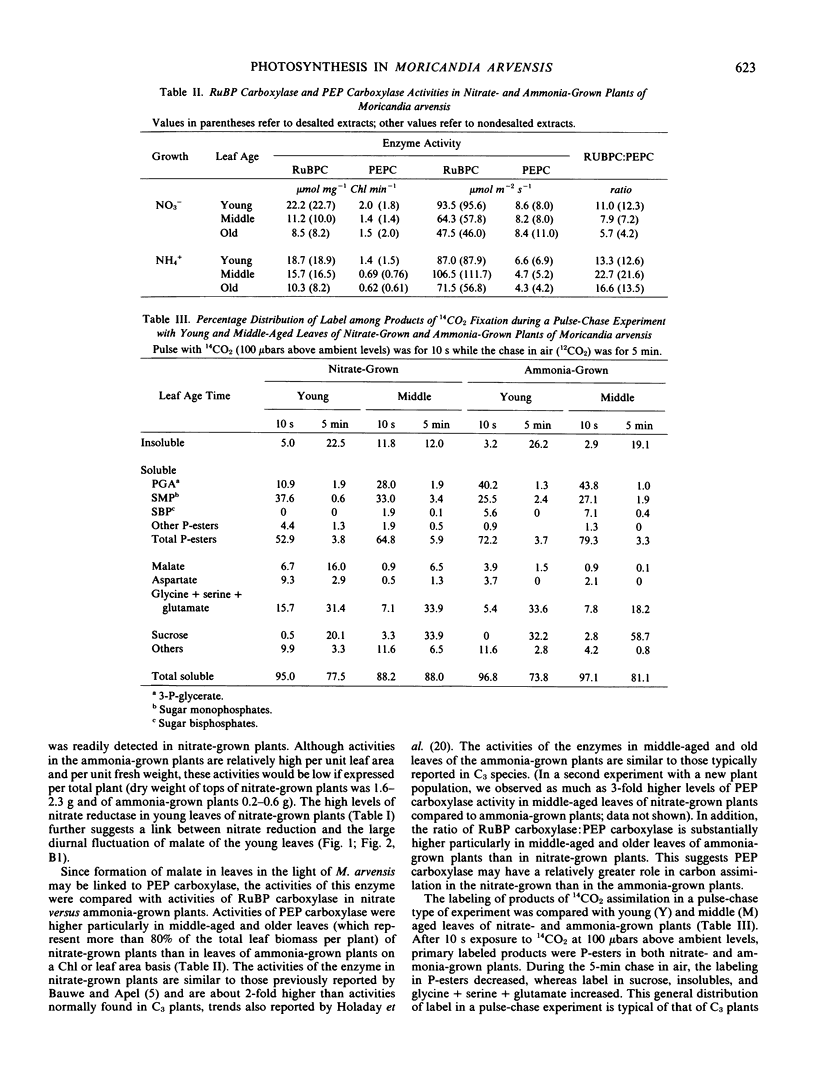
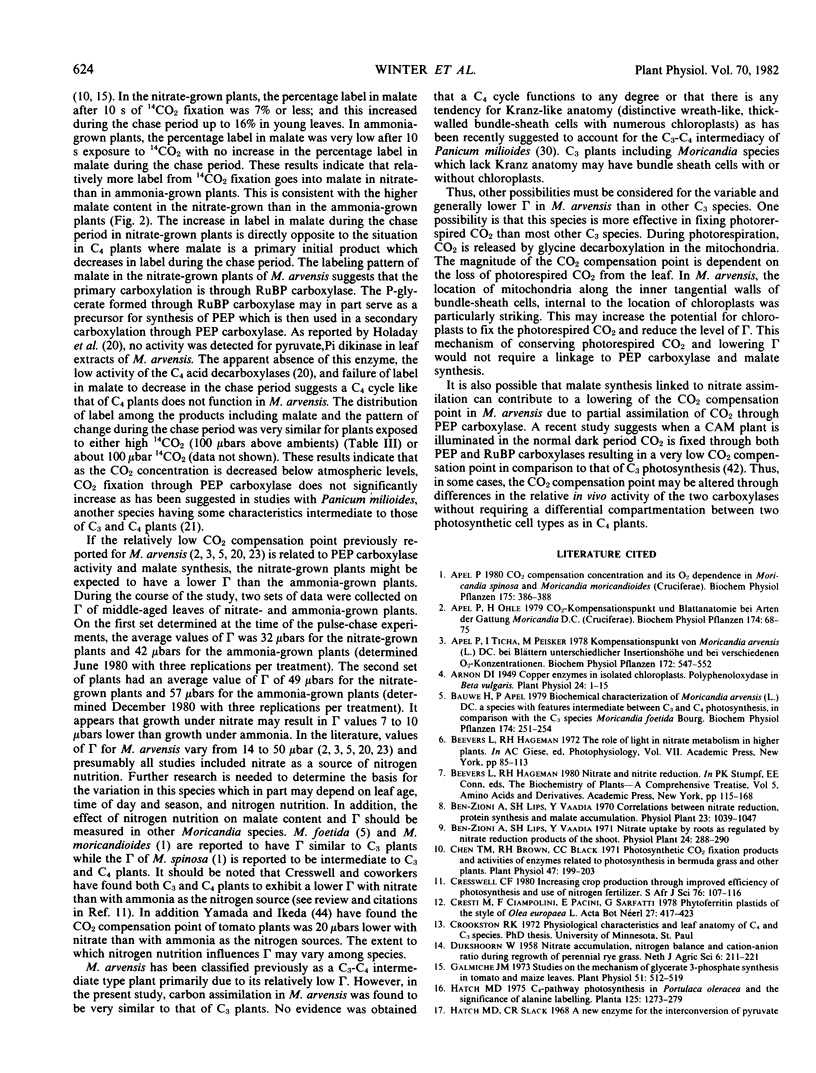
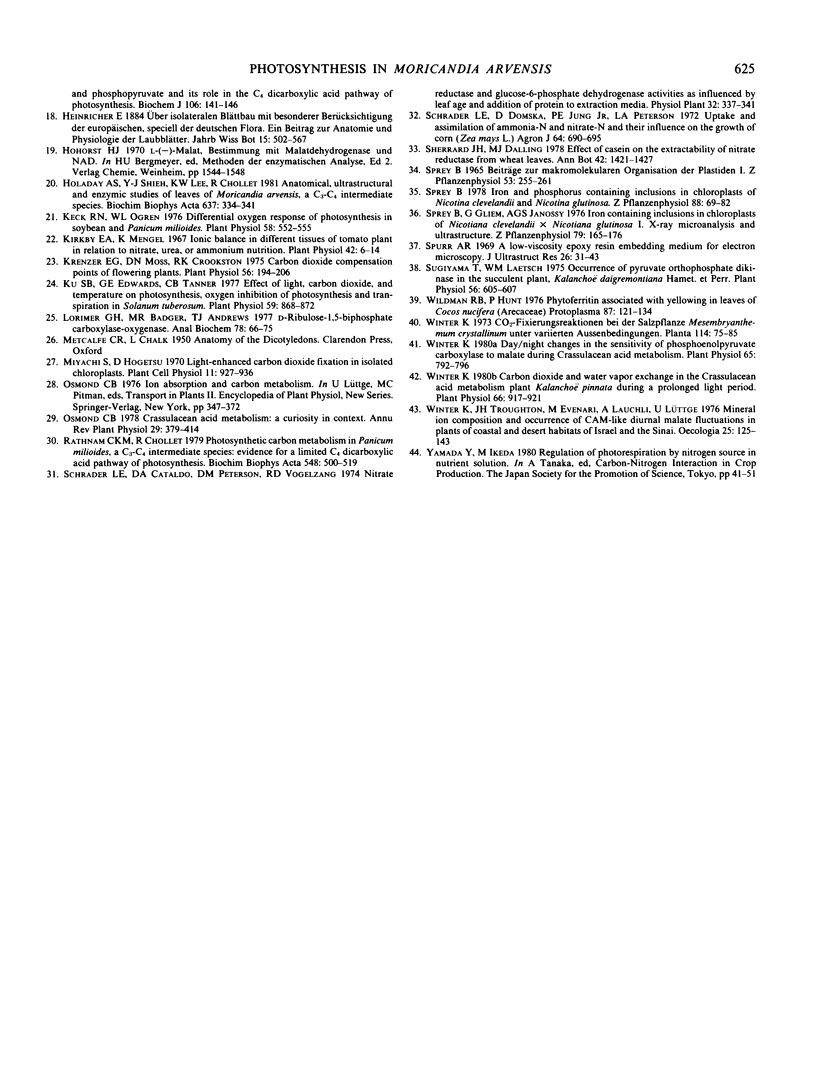
Images in this article
Selected References
These references are in PubMed. This may not be the complete list of references from this article.
- Arnon D. I. COPPER ENZYMES IN ISOLATED CHLOROPLASTS. POLYPHENOLOXIDASE IN BETA VULGARIS. Plant Physiol. 1949 Jan;24(1):1–15. doi: 10.1104/pp.24.1.1. [DOI] [PMC free article] [PubMed] [Google Scholar]
- Beevers L., Hageman R. H. The role of light in nitrate metabolism in higher plants. Photophysiology. 1972;(7):85–113. [PubMed] [Google Scholar]
- Chen T. M., Brown R. H., Black C. C. Photosynthetic CO(2) Fixation Products and Activities of Enzymes Related to Photosynthesis in Bermudagrass and Other Plants. Plant Physiol. 1971 Feb;47(2):199–203. doi: 10.1104/pp.47.2.199. [DOI] [PMC free article] [PubMed] [Google Scholar]
- Galmiche J. M. Studies on the mechanism of glycerate 3-phosphate synthesis in tomato and maize leaves. Plant Physiol. 1973 Mar;51(3):512–519. doi: 10.1104/pp.51.3.512. [DOI] [PMC free article] [PubMed] [Google Scholar]
- Hatch M. D., Slack C. R. A new enzyme for the interconversion of pyruvate and phosphopyruvate and its role in the C4 dicarboxylic acid pathway of photosynthesis. Biochem J. 1968 Jan;106(1):141–146. doi: 10.1042/bj1060141. [DOI] [PMC free article] [PubMed] [Google Scholar]
- Keck R. W. Differential Oxygen Response of Photosynthesis in Soybean and Panicum milioides. Plant Physiol. 1976 Oct;58(4):552–555. doi: 10.1104/pp.58.4.552. [DOI] [PMC free article] [PubMed] [Google Scholar]
- Kirkby E. A., Mengel K. Ionic balance in different tissues of the tomato plant in relation to nitrate, urea, or ammonium nutrition. Plant Physiol. 1967 Jan;42(1):6–14. doi: 10.1104/pp.42.1.6. [DOI] [PMC free article] [PubMed] [Google Scholar]
- Krenzer E. G., Moss D. N., Crookston R. K. Carbon dioxide compensation points of flowering plants. Plant Physiol. 1975 Aug;56(2):194–206. doi: 10.1104/pp.56.2.194. [DOI] [PMC free article] [PubMed] [Google Scholar]
- Ku S. B., Edwards G. E., Tanner C. B. Effects of Light, Carbon Dioxide, and Temperature on Photosynthesis, Oxygen Inhibition of Photosynthesis, and Transpiration in Solanum tuberosum. Plant Physiol. 1977 May;59(5):868–872. doi: 10.1104/pp.59.5.868. [DOI] [PMC free article] [PubMed] [Google Scholar]
- Lorimer G. H., Badger M. R., Andrews T. J. D-Ribulose-1,5-bisphosphate carboxylase-oxygenase. Improved methods for the activation and assay of catalytic activities. Anal Biochem. 1977 Mar;78(1):66–75. doi: 10.1016/0003-2697(77)90009-4. [DOI] [PubMed] [Google Scholar]
- Rathnam C. K., Chollet R. Photosynthetic carbon metabolism in Panicum milioides, a C3-C4 intermediate species: evidence for a limited C4 dicarboxylic acid pathway of photosynthesis. Biochim Biophys Acta. 1979 Dec 6;548(3):500–519. doi: 10.1016/0005-2728(79)90061-6. [DOI] [PubMed] [Google Scholar]
- Spurr A. R. A low-viscosity epoxy resin embedding medium for electron microscopy. J Ultrastruct Res. 1969 Jan;26(1):31–43. doi: 10.1016/s0022-5320(69)90033-1. [DOI] [PubMed] [Google Scholar]
- Sugiyama T. Occurrence of Pyruvate Orthophosphate Dikinase in the Succulent Plant, Kalanchoë daigremontiana Hamet. et. Perr. Plant Physiol. 1975 Nov;56(5):605–607. doi: 10.1104/pp.56.5.605. [DOI] [PMC free article] [PubMed] [Google Scholar]
- Winter K. Carbon Dioxide and Water Vapor Exchange in the Crassulacean Acid Metabolism Plant Kalanchoë pinnáta during a Prolonged Light Period: METABOLIC AND STOMATAL CONTROL OF CARBON METABOLISM. Plant Physiol. 1980 Nov;66(5):917–921. doi: 10.1104/pp.66.5.917. [DOI] [PMC free article] [PubMed] [Google Scholar]
- Winter K. Day/Night Changes in the Sensitivity of Phosphoenolpyruvate Carboxylase to Malate during Crassulacean Acid Metabolism. Plant Physiol. 1980 May;65(5):792–796. doi: 10.1104/pp.65.5.792. [DOI] [PMC free article] [PubMed] [Google Scholar]