Abstract
When cells of Anacystis nidulans strain R2 grown under high CO2 conditions (3%) were transferred to low CO2 conditions (0.05%), their ability to accumulate inorganic carbon (Ci) increased up to 8 times. Cytoplasmic membranes (plasmalemma) isolated at various stages of low CO2 adaptation were analyzed by sodium dodecyl sulfate-polyacrylamide gel electrophoresis. There was a marked increase of a 42-kilodalton polypeptide in the cytoplasmic membrane during adaptation; a linear relationship existed between the amount of this polypeptide and the Ci-accumulating capability of the cells. No significant changes were observed during this process in the amount of other polypeptides in the cytoplasmic membranes or in the polypeptide profiles of the thylakoid membranes, cell walls, and soluble fractions. Spectinomycin, an inhibitor of protein biosynthesis, inhibited both the increase of the 42-kilodalton polypeptide and the induction of high Ci-accumulating capability. The incorporation of [35S]sulfate into membrane proteins was greatly reduced during low CO2 adaptation. Radioautograms of the 35S-labeled membrane proteins revealed that synthesis of the 42-kilodalton polypeptide in the cytoplasmic membrane was specifically activated during the adaptation, while that of most other proteins was greatly suppressed. These results suggested that the 42-kilodalton polypeptide in the cytoplasmic membrane is involved in the active Ci transport by A. nidulans strain R2 and its synthesis under low CO2 conditions leads to high Ci-transporting activity.
Full text
PDF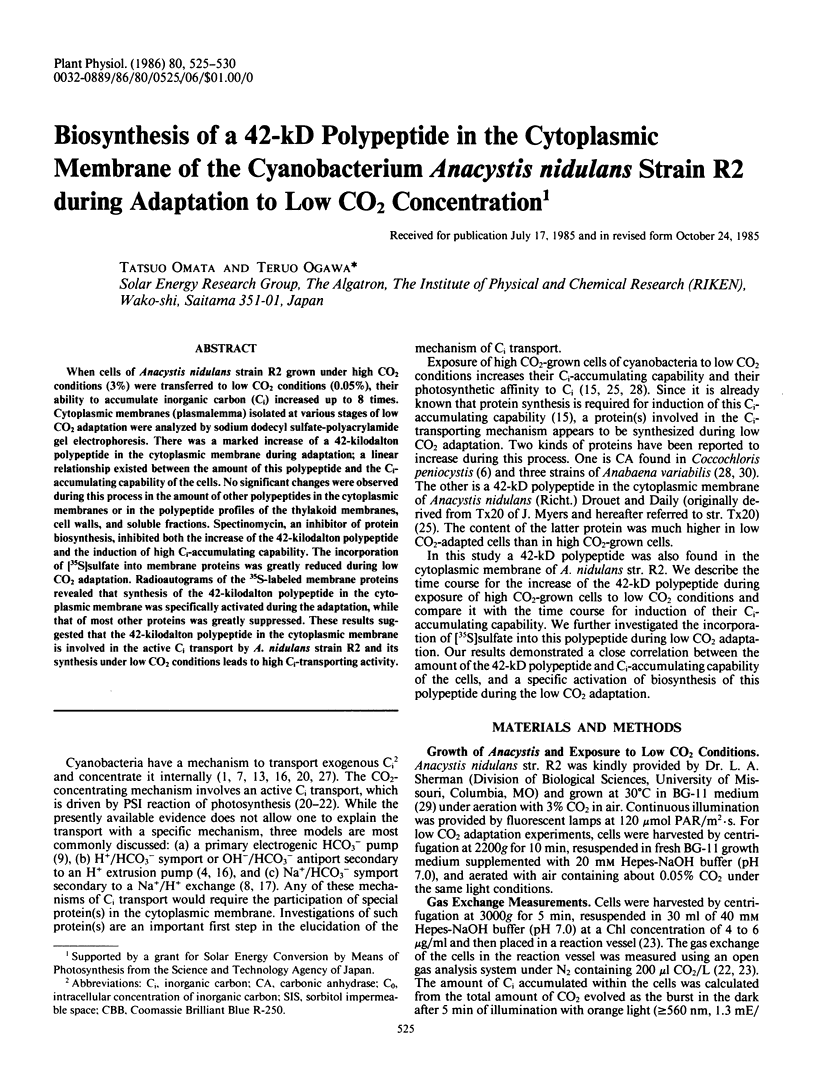
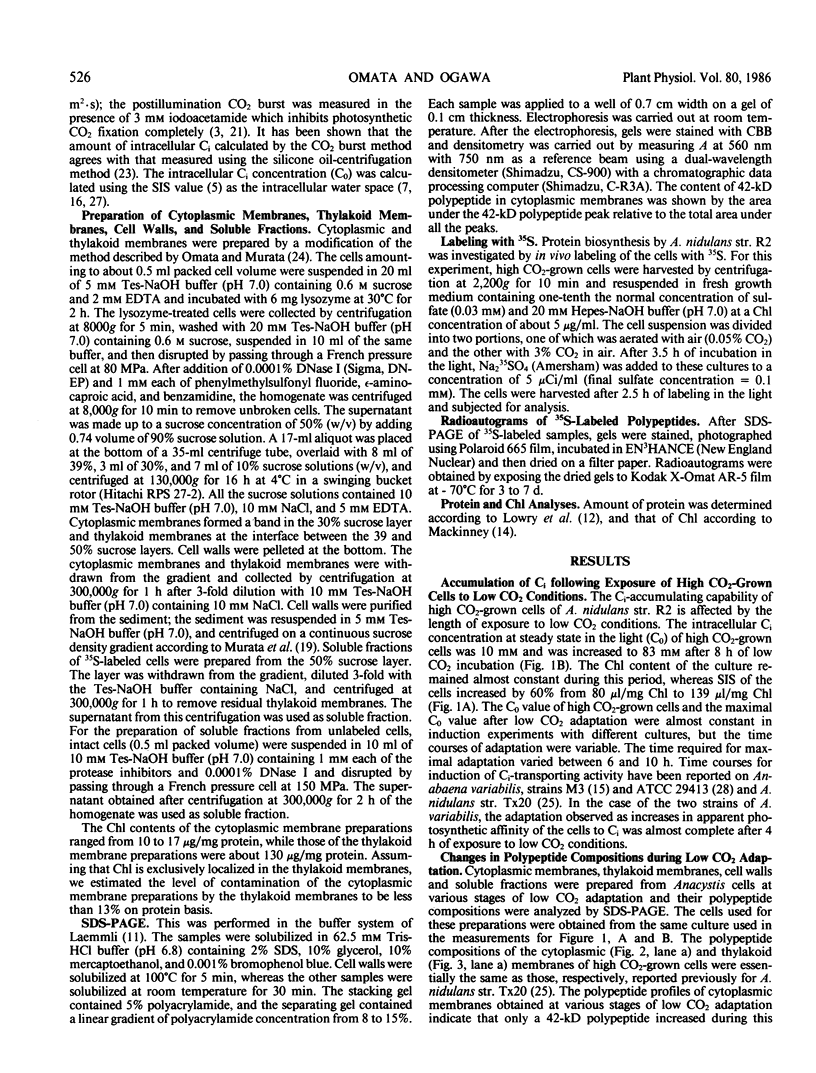
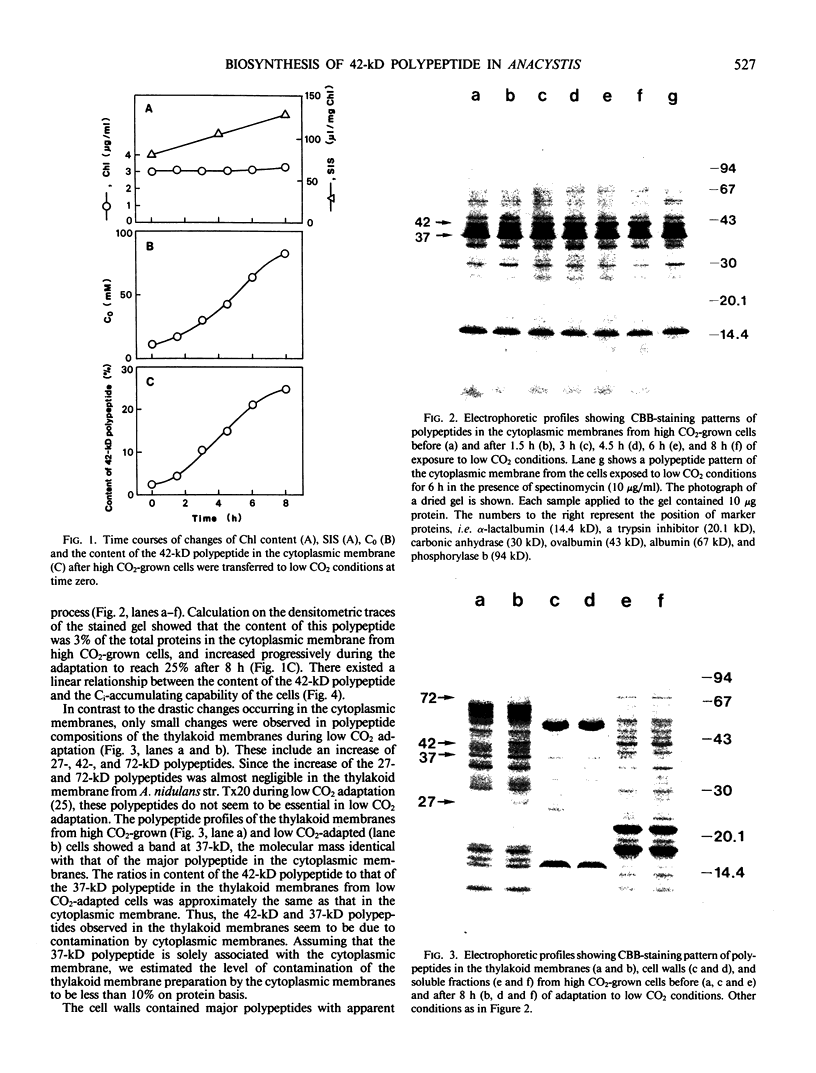
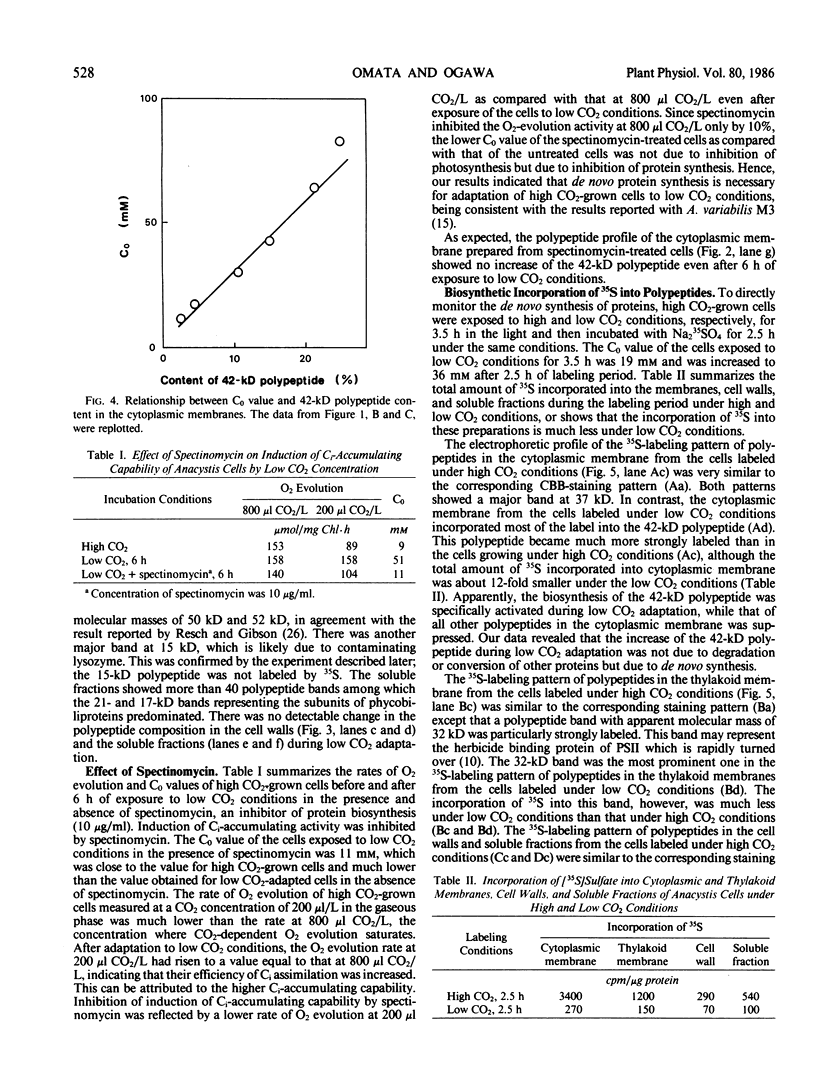
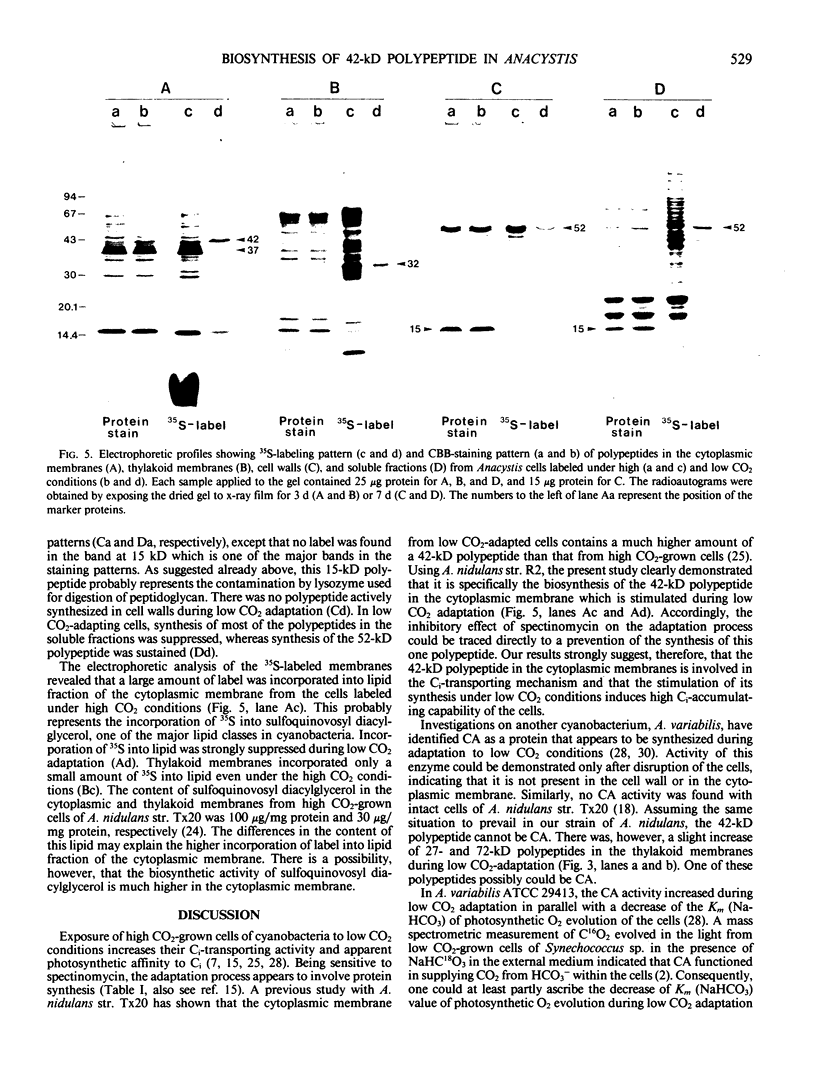
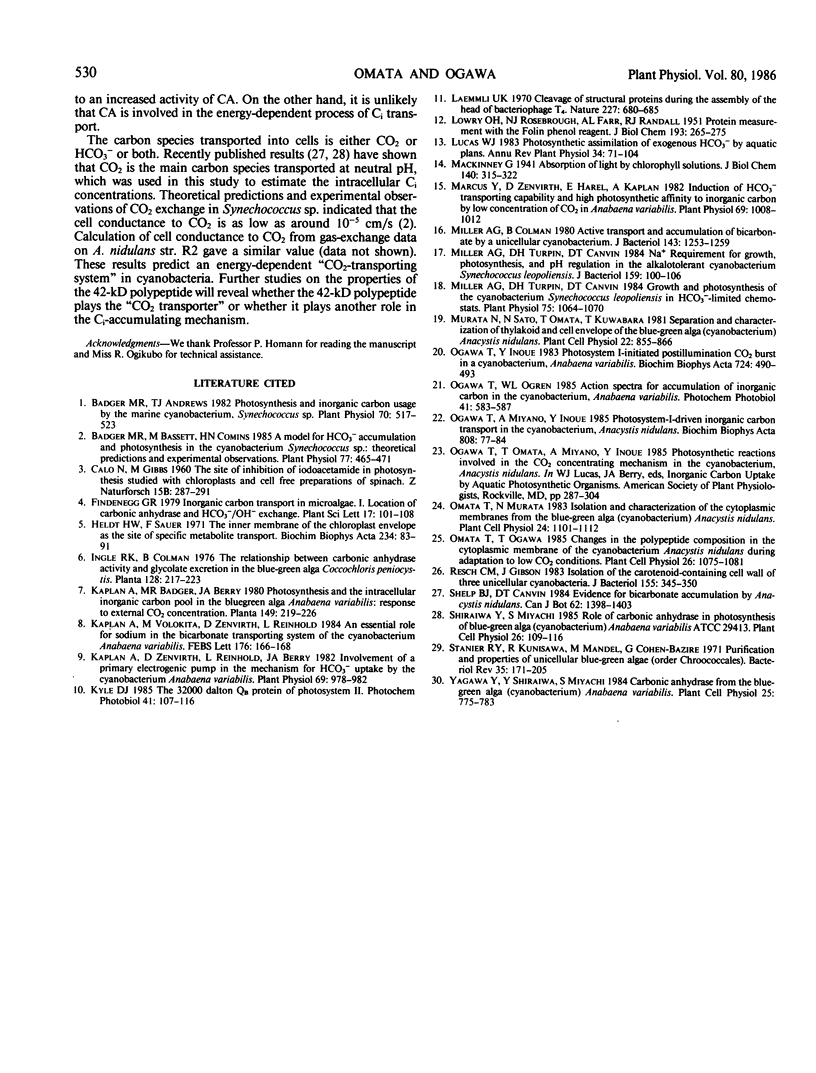
Images in this article
Selected References
These references are in PubMed. This may not be the complete list of references from this article.
- Badger M. R., Andrews T. J. Photosynthesis and Inorganic Carbon Usage by the Marine Cyanobacterium, Synechococcus sp. Plant Physiol. 1982 Aug;70(2):517–523. doi: 10.1104/pp.70.2.517. [DOI] [PMC free article] [PubMed] [Google Scholar]
- Badger M. R., Bassett M., Comins H. N. A Model for HCO(3) Accumulation and Photosynthesis in the Cyanobacterium Synechococcus sp: Theoretical Predictions and Experimental Observations. Plant Physiol. 1985 Feb;77(2):465–471. doi: 10.1104/pp.77.2.465. [DOI] [PMC free article] [PubMed] [Google Scholar]
- CALO N., GIBBS M. The site of inhibition of iodoacetamide in photosynthesis studied with chloroplastses and cell free preparations of spinach. Z Naturforsch B. 1960 May;15B:287–291. doi: 10.1515/znb-1960-0504. [DOI] [PubMed] [Google Scholar]
- Heldt H. W., Sauer F. The inner membrane of the chloroplast envelope as the site of specific metabolite transport. Biochim Biophys Acta. 1971 Apr 6;234(1):83–91. doi: 10.1016/0005-2728(71)90133-2. [DOI] [PubMed] [Google Scholar]
- Kaplan A., Zenvirth D., Reinhold L., Berry J. A. Involvement of a Primary Electrogenic Pump in the Mechanism for HCO(3) Uptake by the Cyanobacterium Anabaena variabilis. Plant Physiol. 1982 Apr;69(4):978–982. doi: 10.1104/pp.69.4.978. [DOI] [PMC free article] [PubMed] [Google Scholar]
- LOWRY O. H., ROSEBROUGH N. J., FARR A. L., RANDALL R. J. Protein measurement with the Folin phenol reagent. J Biol Chem. 1951 Nov;193(1):265–275. [PubMed] [Google Scholar]
- Laemmli U. K. Cleavage of structural proteins during the assembly of the head of bacteriophage T4. Nature. 1970 Aug 15;227(5259):680–685. doi: 10.1038/227680a0. [DOI] [PubMed] [Google Scholar]
- Marcus Y., Zenvirth D., Harel E., Kaplan A. Induction of HCO(3) Transporting Capability and High Photosynthetic Affinity to Inorganic Carbon by Low Concentration of CO(2) in Anabaena variabilis. Plant Physiol. 1982 May;69(5):1008–1012. doi: 10.1104/pp.69.5.1008. [DOI] [PMC free article] [PubMed] [Google Scholar]
- Miller A. G., Colman B. Active transport and accumulation of bicarbonate by a unicellular cyanobacterium. J Bacteriol. 1980 Sep;143(3):1253–1259. doi: 10.1128/jb.143.3.1253-1259.1980. [DOI] [PMC free article] [PubMed] [Google Scholar]
- Miller A. G., Turpin D. H., Canvin D. T. Growth and Photosynthesis of the Cyanobacterium Synechococcus leopoliensis in HCO(3)-Limited Chemostats. Plant Physiol. 1984 Aug;75(4):1064–1070. doi: 10.1104/pp.75.4.1064. [DOI] [PMC free article] [PubMed] [Google Scholar]
- Miller A. G., Turpin D. H., Canvin D. T. Na+ requirement for growth, photosynthesis, and pH regulation in the alkalotolerant cyanobacterium Synechococcus leopoliensis. J Bacteriol. 1984 Jul;159(1):100–106. doi: 10.1128/jb.159.1.100-106.1984. [DOI] [PMC free article] [PubMed] [Google Scholar]
- Resch C. M., Gibson J. Isolation of the carotenoid-containing cell wall of three unicellular cyanobacteria. J Bacteriol. 1983 Jul;155(1):345–350. doi: 10.1128/jb.155.1.345-350.1983. [DOI] [PMC free article] [PubMed] [Google Scholar]
- Stanier R. Y., Kunisawa R., Mandel M., Cohen-Bazire G. Purification and properties of unicellular blue-green algae (order Chroococcales). Bacteriol Rev. 1971 Jun;35(2):171–205. doi: 10.1128/br.35.2.171-205.1971. [DOI] [PMC free article] [PubMed] [Google Scholar]